Abstract
Cell-free DNA-based noninvasive prenatal testing (NIPT) has emerged as a valuable prenatal test option without intervention risk and capable to detect trisomy 13, 18, 21 and gonosomal aneuploidies with high sensitivity and specificity. Although a plethora of validation studies in high- and low-risk patients underline its excellent performance, several case reports with result discordancy to the direct fetal karyotype justify a confirmation of all positive cfDNA-based test results by invasive testing. Therefore cfDNA prenatal testing was characterized as a screening method, rather than a diagnostic test. The main reason for this is that cell-free placental DNA in maternal blood serves as a proxy for the fetus with large amounts of maternal cfDNA in the background. Discordances can arise due to placental mosaicism, cotwin demise, chromosomal- and subchromosomal aberrations in the mother and fetal fraction affecting maternal factors. This chapter discusses these phenomena and their clinical implications.
Keywords
Noninvasive prenatal testing, cfDNA, Cell-free fetal DNA, Cell-free placental DNA, Confined placental mosaicism, True fetal mosaicism, Vanishing twin, Rare autosomal trisomies, Sex discordancy, Maternal factor
Introduction
Since the commercial introduction of cfDNA-based prenatal tests in 2011, a plethora of studies with designs of proof of concept, validation, case reports, large cohort studies, and meta-analyses have been published. Although cfDNA-based prenatal screening for trisomy 21, 18, and 13 has made big steps forward from the very early stages of its use, it soon became evident that false-positive and false-negative results might occur and that many of these have biological causes. Knowledge and understanding of the causes of a false result is essential to enable clinicians and genetic counselors to counsel the patients comprehensively and appropriately, both prior to a test as well as after having received the test result. This will ensure responsible application of an innovative prenatal testing method and allow evidence-based decision making for a mother and subsequently her fetus.
Diagnostic Tests and Screening Tests
The performance of a new test method is usually described by four characteristics: sensitivity, specificity, and positive and negative predictive value. The latter two depend on the prevalence of the disease in a given population. The combination of sensitivity and false-positive rate (1 − specificity) allows a test method to be classified as a screening- or a diagnostic test for a given prevalence and cutoff. Sensitivity is defined as the ability of a test to correctly identify patients with a disease. In contrast, specificity describes the ability of a test to identify persons without the disease. These two test variables are fundamentally different between a diagnostic test and a screening test: diagnostic tests require both sensitivity and specificity to be as close as possible to 100%, whereas screening tests are usually characterized by either a high sensitivity or a high specificity. cfDNA-based prenatal testing for trisomy 21 has both, a high sensitivity and a high specificity . The occurrence of false results though prevents it from being a diagnostic test. Most of these false results find their origin in the placenta or in the mother. They may have an irreversible impact on pregnancy management and decisions about pregnancy termination. cfDNA-based prenatal testing is still considered as a screening test implicating confirmation of all positive test results by an independent test method.
Sensitivity and Specificity of CELL-FREE DNA-Based Prenatal Testing and Their Consequences for Predictive Values
Several meta-analyses on cfDNA-based prenatal testing have been published to date . To illustrate the current performance of cfDNA-based prenatal tests, the results of Mackie et al. are shown here .
Table 1 shows the sensitivity and specificity of cfDNA-based prenatal tests for trisomy 13, 18, 21 and monosomy X, which for trisomy 21 reach levels close to 100%. The sensitivity is highest for trisomy 21 and decreases for trisomy 18, monosomy X, and trisomy 13. Neither the cfDNA NIPT method (whole genome or targeted sequencing; qPCR or microarray data were not included) nor the test population’s a priori risk (high risk vs low risk) impacted test characteristics. Reasons for false results mentioned in individual studies were as follows: fetal fraction below a predefined cutoff, confined placental mosaicism (CPM), and maternal copy number variations (CNVs) were the most common explanations provided. Gil et al. , who only included studies reporting clinical outcome in more than 85% of cases, reported slightly higher detection rates: 99.7% for trisomy 21, 97.9% for trisomy 18, 99% for trisomy 13, and 95.8% for monosomy X.
Performance | Trisomy 21 | Trisomy 18 | Trisomy 13 | Monosomy X |
---|---|---|---|---|
n tested | 148,344 | 146,940 | 134,691 | 6712 |
Sensitivity (%;95%CI) | 99.4 (98.3–99.8) | 97.7 (95.2–98.8) | 90.6 (82.3–95.8) | 92.9 (74.1–98.4) |
Specificity (%;95%CI) | 99.9 (99.9–100.0) | 99.9 (99.8–100.0) | 100.0 (99.9–100.0) | 99.9 (99.5–99.9) |
To further illustrate the performance metrics, we assume a hypothetical general obstetric population of 100,000 patients with a trisomy 21 prevalence of 0.5%. This translates into 500 pregnant women carrying a fetus with trisomy 21. cfDNA-based prenatal testing, with its meta-analytical sensitivity of 99.4% identifies 497 of them, while three cases will be missed. From the 99,500 pregnancies without trisomy 21, ~ 100 will receive a false-positive result given the specificity of 99.9%. A positive predictive value (PPV) can be calculated with these values and shows how likely it is that a pregnant woman with a trisomy 21 positive cfDNA NIPT report actually carries a fetus with trisomy 21: given a disease prevalence of 0.5%, the PPV will be 83.2%. By comparison, in a higher risk population with a trisomy 21 prevalence of 3%, 3000 out of 100,000 patients carry a fetus with trisomy 21: 2982 trisomy 21 cases will be detected but 18 will be missed; 97,000 pregnant women are trisomy 21 negative, but 97 will receive a false-positive result. The PPV will be 96.8%. These hypothetical calculations demonstrate that PPVs depend on disease prevalence and that the personal a priori risk influences the significance of an abnormal cfDNA NIPT result for the individual patient. As trisomy negative pregnancies are always in excess in a pregnant population (representing a high nondisease prevalence) and meta-analytical specificity values of cfDNA-based NIPT reach almost 100% independent of the chromosome tested, the negative predictive value (NPV) is always close to 100% both in high- and low-risk populations ( Table 2 ).
Outcome | Predictive Value of the Test | |||
---|---|---|---|---|
Trisomy | Disomy | |||
NIPT result | Abnormal | True positive (TP) | False positive (FP) | Positive predictive value (PPV) TP/TP + FP |
Normal | False negative (FN) | True negative (TN) | Negative predictive value (NPV) TN/TN + FN | |
Test performance | Sensitivity TP/TP + FN False-negative rate (FNR) = 1 − sensitivity | Specificity TN/TN + FP False-positive rate (FPR) = 1 − specificity |
False-Positive Results of CELL-FREE DNA-Based Prenatal Testing
The main reason why cfDNA-based prenatal testing is currently classified as a screening test is the occurrence of false-positive results. These have important implications for pregnancy management and parental decisions.
Biological Reasons for False-Positive Results of CELL-FREE DNA-Based Prenatal Testing
Confined placental mosaicism
CPM is the existence of two (or more) cell lines with different chromosomal complements in a fetoplacental unit derived from a single zygote . Genetic mosaicism can be a result of two types of cell division errors ( Figs. 1 and 2 ). First, after the development of a euploid zygote, mitotic errors can occur in part of the cells, and three different cell lines may be generated: a normal diploid cell line, a trisomic cell line, and a monosomic one; in general, the latter cannot proliferate further and undergoes apoptosis. Depending on the developmental stage of the embryo at the time of this mitotic error, a generalized- or a confined placental mosaicism evolves.


A second origin of CPM are meiotic errors during germ cell production. These result in a trisomic zygote; in later developmental stages this aneuploid zygote can be “rescued” by an additional mitotic error. The karyotype in the embryo depends on its developmental stage at the time of rescue: rescue in early stages implies a generalized mosaicism; a late stage trisomy rescue can confine the trisomy to the extra-embryonic compartment, the future placenta. The postzygotic correction can also lead to (mosaic) uniparental disomy (UPD), a disomy with a pair of homologous chromosomes from one parent only.
Depending on the affected tissue types, one can categorize fetoplacental discrepancies in three subtypes of CPM when the karyotype in amniocytes is normal and three subtypes of true fetal mosaicism (TFM) when the karyotype in amniocytes is abnormal . Differences between each category and expected cfDNA NIPT results are outlined in Fig. 3 .

Cell-free “fetal” DNA originates from the cytotrophoblast and syncytiotrophoblast. Aneuploidy in this external layer of the chorionic villus leads to CPM types 1 and 3 and causes false-positive cfDNA NIPT results. In TFM type 5 and complete fetoplacental discordance, either the cytotrophoblast or both cytotrophoblast and mesenchyme are normal, while the fetus is not, generating false-negative cfDNA NIPT results . Experience with chorion villus (CV) analysis shows an overall prevalence of mosaicism of 2%. CPM type 1 occurs more frequently than CPM type 3. TFM type 5 is the least probable scenario . Trisomy 18 and trisomy 21 are less frequently involved (4% and 2% of mosaic cases, respectively) than trisomy 13 and monosomy X (22% and 59% of all cases) . Also, the likelihood that a mosaic trisomy in CV is confirmed in the fetus differs per chromosome. Fetal confirmation rates were highest for trisomy 21 (34%) followed by monosomy X (26%), trisomy 18 (17%), and trisomy 13 (2.4%) . Similar figures were reported in another publication by the same group . Taken together, these data explain why cfDNA-based NIPT has better PPVs for trisomy 21 than for trisomy 18 and 13: trisomy 21 rarely presents as a mosaic in chorionic villi and if so, is more likely to be confirmed in the fetus.
Even if not confirmed in the fetus, CPM is not harmless. A (mosaic) trisomic placenta is not a healthy placenta as has already been demonstrated many years ago . Spontaneous miscarriage, intrauterine fetal growth restriction, preterm delivery, and fetal demise can all be a result of altered placental function due to CPM . This will be discussed in more detail in Chapter 7 . Imprinting effects due to UPD after trisomy rescue are responsible for several clinical syndromes, for example, transient neonatal diabetes (paternal UPD 6), Silver-Russell syndrome (maternal UPD 7), and Prader-Willi and Angelman syndrome (maternal and paternal UPD15) . Apart from imprinting effects, UPD also increases the risk for recessive disorders due to loss of heterozygosity.
Any CPM with a sufficient percentage of trisomic cells in the cytotrophoblast can be identified by cfDNA NIPT. Mosaicism is likely if the trisomic fraction is lower than the fetal fraction . Another point of consideration is that in case of a small placenta as a result of CPM the fetal fraction may be lower, and this might translate in a cfDNA NIPT test failure. This correlates with the observation that there is a 2.5–6.7 times higher prevalence of aneuploidy among failed cfDNA NIPT cases analyzed with targeted enrichment-based NIPT methods requiring a minimum fetal fraction of 4% as compared to MPS-based cfDNA NIPT tests . Additionally, CPM can negatively influence the effective trisomic fraction. The fetal fraction, by definition, is the sum of all locations of the placental cytotrophoblast releasing cfDNA into the maternal blood stream. CPM might not be distributed equally throughout the placenta: some regions can have alternate ratios of normal to trisomic cells compared to the rest of the placental regions. This phenomenon is illustrated in a case report, where a mosaic double trisomy 18 + 21 (T18 + T21) resulted in a NIPT result positive for T21, but negative for T18 . By multilocus placenta biopsy, regions in the placenta could be identified, which had significantly more T21 positive cells (61%) than T18 positive cells (22%). These regions were responsible for a decreased T18 trisomic fraction, also called the “effective” fetal fraction for T18. The term effective fetal fraction in the context of CPM is also illustrated well by a case report of low-level CPM of trisomy 18 where the effective fetal fraction was calculated : in multiple placental biopsies, the mosaic level for T18 was on average 30% for all cells. The overall fetal fraction was 7.4%, and the mosaicism hence resulted in an effective fetal fraction of ~ 2.2%, which was too low for the NIPT test method used to detect the trisomy 18.
In summary, in this section we have described the types, frequency, origins, and other clinical effects of CPM including its influence on cfDNA NIPT accuracy. In the context of cfDNA NIPT, CPM has to be considered one of the main reasons for “false” NIPT results. Strictly spoken, these results are not false, since cfDNA NIPT is a liquid biopsy of the placenta and accurately reflects the karyotype of the cytotrophoblastic layer and the proportion of abnormal cells. But due to the phenomenon of fetoplacental discrepancies, cfDNA NIPT does not always reflect the fetal genome. The term “cell-free fetal DNA” is therefore incorrect and should be replaced by “cell-free placental DNA” or “cell-free DNA” since maternal plasma will always contain a pool of both, fetal and maternal cfDNA.
Vanishing twins
A second cause of false-positive cfDNA NIPT results is vanishing twins. A vanishing twin is defined as a fetus of a multigestational pregnancy that died in utero. A large cohort study reanalyzed biobanked mother-child samples of cases of false-positive prenatal fetal rhesus D ( RHD ) assignment and estimated the frequency of vanishing twins to be 0.6% . The reported incidence of vanishing twins after IVF/ICSI was ~ 13% of all twin pregnancies . The frequency of twin pregnancies increases with maternal age and with the use of assisted reproduction. In a study including 32 vanishing twins, median maternal age was significantly higher than in viable twins .
In spontaneous abortions both in cases with a normal and an abnormal karyotypes placental cfDNA and total cfDNA increases . A dichorionic twin placenta releases two individual fractions of cfDNA into the maternal circulation and these are analyzed in concert during cfDNA-based NIPT. An aneuploid vanishing twin might therefore cause a false-positive cfDNA NIPT result for the fetus that is alive . Theoretically, an “overrepresented” euploid vanishing twin, releasing excess amounts of cfDNA in the maternal circulation, could mask aneuploidy in the fetus that is alive and lead to a false-negative cfDNA NIPT result. This has not been described in literature. Along the same lines an unnoticed male vanishing twin can be responsible for a discrepancy between fetal sex according to cfDNA NIPT results and ultrasound imaging. Fetal sex discordances are discussed further in this chapter.
In cases of vanishing twins with discordant sex, comparing sex-dependent with sex-independent methods of fetal fraction estimation allows to deduce the fetal subfraction of each twin . Likewise, if the vanishing twin is aneuploid and the living twin is not, an aneuploid fraction that is significantly lower than the fetal fraction can point toward a vanishing twin, or placental mosaicism .
The effect of vanishing twins on NIPT results depends on their ability to release cfDNA into the maternal blood stream. There is little data about how long after demise the placenta of the demised fetus still contributes to the “fetal” DNA pool in maternal plasma. SNP technologies allow to identify an extra haplotype. With this technology the presence of a third haplotype has been demonstrated in known vanishing twin cases up to 8 weeks after the estimated spontaneous demise of the cotwin. . Thurik et al. demonstrated that a vanishing twin was the most likely cause of genotyping a 27-week fetus as RHD positive, while the newborn was serotyped RhD negative, meaning that in this case, cfDNA of a vanishing twin had persisted into the second trimester of pregnancy .
Early detection of a vanishing twin can be achieved by ultrasound examination. In the context of first trimester screening, early ultrasound with attention for the eventual presence of a vanishing twin is indispensable for correct test result interpretation. Likewise, an abnormal cfDNA NIPT result should be followed by an ultrasound explicitly looking for a vanishing twin.
The use of cfDNA NIPT in intact twin pregnancies is discussed in more detail in Chapter 6 .
Rare autosomal trisomies and large segmental aberrations
Rare autosomal trisomies are trisomies other than those involving the chromosomes 13, 18, 21, X and Y. In nonmosaic form, these trisomies are usually not compatible with life and they have therefore rarely been seen as results of invasive prenatal diagnosis in amniotic fluid cells. In mosaic form, rare autosomal trisomies have been associated with intrauterine growth restriction, fetal death, true fetal mosaicism, and UPD. All cell-free DNA NIPT methods that are based on comparison of targeted chromosomes with reference chromosomes will be influenced by a trisomy or monosomy or large CNV in the reference chromosomes. Whole genome sequencing and analysis offers an opportunity to fully understand the underlying biological causes of these unusual results and decrease the false-positive rate . Bioinformatic causes of false-positive results are discussed in Chapter 3 and Rare autosomal trisomies in Chapter 7 .
Fetal sex discordances
Noninvasive fetal sex determination can reliably be done by quantitative PCR (qPCR). Amplification of the sex determining region of the Y-chromosome ( SRY ) gene, either exclusively or in combination with the DYS14 marker, is sufficient to achieve high sensitivity and specificity, provided the presence of enough cell-free placental DNA is controlled for . A meta-analysis reviewing 90 studies published between 1997 and 2010 evaluated the performance of noninvasive cfDNA-based fetal sex determination and calculated a median sensitivity of 96.6% and a median specificity of 98.9% . For fetal sex determination for medical reasons in the context of noninvasive prenatal diagnosis (NIPD), as for women who are carriers of sex-linked disorders, the specificity should be 100%. For NIPD stringent diagnostic algorithms and additional tests to control for the presence of fetal DNA have therefore been put in place .
There are biological reasons for discordancies in sex assignment between cfDNA NIPT, ultrasonography, and fetal or newborn karyotyping ( Fig. 4 ).

Full access? Get Clinical Tree
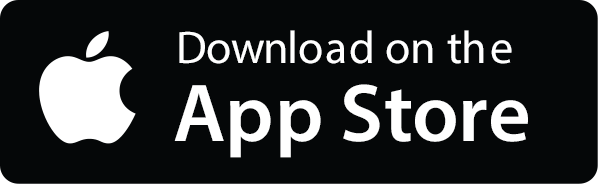
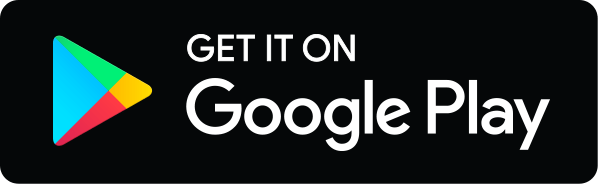