Introduction
Viral infections during pregnancy are very common; it is estimated that symptomatic viral infections affect at least 5.2% of pregnancies [1]. Reported incidence rates underestimate actual rates as many infections are asymptomatic for the mother and yet can have deleterious consequences for the offspring; as an example, approximately 1% of newborns excrete cytomegalovirus (CMV) at birth. Several factors have to interact for viral infections to affect pregnancy and some of them are amenable to preventive strategies from the clinician’s point of view. A key factor is the proportion of women of childbearing age susceptible to a particular virus and the exposure these women have to a population where the virus circulates. Other factors, including the virus “activity” within the population and innate characteristics of the virus such as infectivity and transmissibility, warrant awareness on the clinician’s part.
Prevention is the foremost strategy to curb the occurrence of viral infections during pregnancy. Ensuring that women of reproductive age receive appropriate vaccinations in a timely manner can certainly prevent a proportion of viral infections during pregnancy, as 50–60% of pregnancies are unplanned [2,3]; preconception testing for immunity status remains an option for planned pregnancies, but this is not the most common scenario the clinician deals with. Pregnancy surveillance may allow identification of women at the highest risk for specific infections and may identify fetal infection. Screening at birth is useful if vaccination or treatment of newborns at risk is available.
Pathogenesis of viral infections during pregnancy
Viruses are obligate intracellular pathogens. They require the host cell apparatus to replicate and by doing so they compromise key cellular processes, such as nucleic acid and protein synthesis, and preservation of membrane integrity. Therefore, they invariably result in premature cell death or abnormal cell survival [4,5]. Among immunocompetent adults, the cellular damage arising from most viral infections is repairable unless infection affects not easily repairable tissues like the central nervous system. Viral infections during pregnancy pose the extra challenge of a developing fetus potentially more susceptible to permanent sequelae.
In general, viruses are composed of a nucleocapsid, consisting of viral nucleic acid, i.e. double- or single-stranded DNA or RNA, packaged in a protein coat, referred as the capsid. The viral nucleic acid often includes the enzymes required for the initial steps in viral replication, whereas the capsid allows survival in the environment and binding to host cells. For most viruses the nucleocapsid is surrounded by a lipid envelope that usually contains the immunogenic proteins that elicit antibody response.
Maternal acquisition of infectious viruses happens from inhalation of droplets or aerosols, e.g. varicella-zoster virus (VZV), ingestion of fecally contaminated food or water, e.g. hepatitis A (HAV) or polio, or exposure to contaminated body fluids or tissues (blood, saliva, urine, genital secretions, or transplanted organs), e.g. herpes simplex virus (HSV) or HIV. Viral spread to lymph nodes, skin, mucosa and viscera varies according to the virus itself, but the virus must be present in the maternal bloodstream, the birth canal or in breast milk for transmission to the fetus or neonate to happen.
The mechanisms by which viral agents may produce adverse effects on the fetus arise from direct viral injury, e.g. chromosomal damage, cellular death and/or antigen/antibody formation, or indirectly from placental dysfunction, e.g. altered placental circulation, placental thrombosis or placentitis, secondary to maternal fever or metabolic derangements.
The fetal or neonatal complications arising from maternal viral infections depend on several factors, including gestational age at which the fetus is exposed to the virus, as placental and fetal immunity evolves during pregnancy; type of maternal infection: primary, reactivation or reinfection, as pre-existing maternal immunity may not be 100% effective to protect the fetus, but does decrease the burden of disease; viral factors, like virulence or cellular tropism. Viral exposure during the first trimester can result in resorption of the embryo, miscarriage, teratogenesis, and/or persistent fetal infection. Viral exposure during the second half of pregnancy is more likely to result in transient fetal infection or subclinical diseases that progress after delivery. Other consequences include prematurity, intrauterine growth retardation (IUGR), neurodevelopmental delay or no apparent sequelae at all.
Modes of transmission to the fetus
Maternal viral infections during pregnancy can be transmitted to the fetus while in utero or at the time of delivery. Maternal viremia may result in placental infection without fetal infection, infection of both placenta and fetus, or absence of both fetal and placental infection. The mechanism for transmission of most in utero infections in this review is transplacental, implying maternal viremia as the starting point followed by placental infection and subsequent release of viral particles into the fetal bloodstream. However, other mechanisms for in utero infection, that occur very rarely, include transamniotic transmission in the setting of chronic chorio-amnionitis[6] and iatrogenic direct viral inoculation into fetal circulation during diagnostic or therapeutic procedures[7].
For the purposes of our discussion, we have classified viral infections in pregnancy according to their main impact on maternal, fetal and/or neonatal morbidity and mortality, based on the currently published data, pointing out for the reader the instances where controversy exists and further research is required (see Table 17.1).
Table 17.1 Consequences of viral infections during pregnancy
Immune response to viral infections during pregnancy
Contrary to commonly held beliefs, pregnancy is not synonymous with general maternal immune suppression, but rather a state of complex adaptations, still poorly understood, that allow the maternal immune system to recognize and react against the foreign antigens of the fetus in a “positive way,” i.e. limiting trophoblast invasion to ensure maternal corporal integrity while ensuring adequate placentation [8]. Maternal antibody production in response to vaccines is preserved in pregnancy, which presents a unique opportunity to prevent disease for the mother and her offspring, and when challenged with various stimuli, the immune response of pregnant women is not significantly reduced when compared to nonpregnant women [9].
Epidemiologic data on increased frequency and/or severity of intracellular infections during pregnancy have led to the assumption that cell-mediated immunity is adversely affected. Effective immunity against viruses requires both arms of the immune system: innate and adaptive. Each arm has humoral (cytokines, complement and antibodies) and cellular components (granulocytes, natural killer (NK) and dendritic cells for the innate arm and T-lymphocytes, both helper and CD8+, and B-cells for the adaptive arm) (Figure 17.1). Innate immunity implies intrinsic resistance to microbes, not acquired through previous contact, like the one mediated by pattern recognition receptors (PRR) or Toll-like receptors (TLR), which recognize highly conserved sequences unique to microbes such as double-stranded RNA, peptidoglycans and lipopolysacharides [10]. Adaptive immunity requires contact with the microbe; hence it is very specific but takes at least 3– 4 days to reach minimally effective levels; however, it is followed by immunologic memory, which offers partial if not total protection against subsequent infections by the same organism. Activation of the adaptive immunity requires the prior activation of innate immunity and “antigen presentation” by antigen-presenting cells (APC) to T-lymphocytes. Antigens are presented by molecular histocompatibility complex (MHC) membrane proteins; MHC-I are present in all somatic cells (except neurons and red blood cells (RBC)) and MHC-II only on APC, like macrophages. Human leukocyte antigens (HLA) are the MHC in humans.
Figure 17.1 Immunology of pregnancy: overview. (A) Effective immunity against viruses requires both arms of the immune system: innate and adaptive. The two arms of the immune system, innate and adaptive, each include a cellular component (granulocytes, natural killer (NK) and dendritic cells for the innate arm, and lymphocytes (T-lymphocytes, both helper and CD8+, and B-cells) for the adaptive arm) and a humoral component (cytokines, complement and antibodies). (B) Pregnancy is associated with a generalized inflammatory response. During pregnancy, the innate immune system is activated and the adaptive immune, particularly T-cell, functions appear to be downregulated during gestation; this complex interaction is thought to be crucial for maintenance of a successful pregnancy. Adapted from Luppi [11].
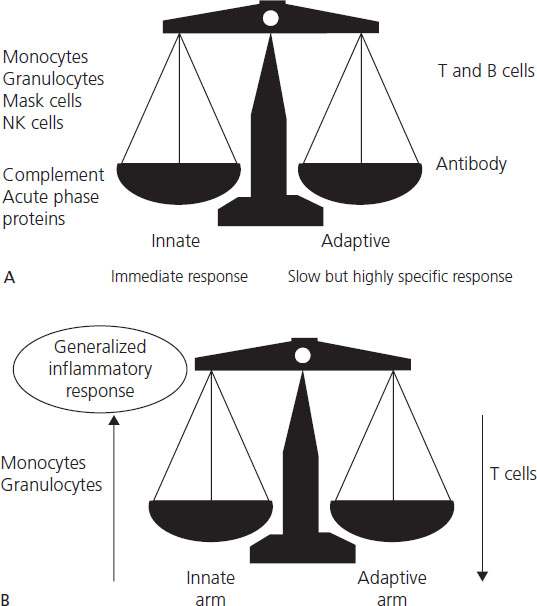
Simplistically, following infection viruses undergo rapid replication using the host cell apparatus for protein synthesis and viral replication; viral antigens then are available to be presented by HLA-I molecules to CD8+ T-cells (cytotoxic T-cells (CTL)), resulting in production of virus-specific clones of B-lymphocytes responsible for antibody production and specific T-lymphocytes.
Innate immunity against viruses (Figure 17.2) is triggered by viral destruction of cells, recognition of infected cells by NK cells (which are able to recognize cells not expressing HLA-I and otherwise evading immune surveillance) or direct interaction of complement with virions, leading to cytokine release, kinin and complement activation and recruitment of monocytes and neutrophils. Normal pregnancy is characterized by generalized upregulation of granulocytes, monocytes and complement [11]. NK cell cytotoxic function is reduced in the peripheral maternal circulation, but NK cells are the predominant leukocyte at the decidua.
Figure 17.2 Immune response to viruses. Effective immunity against viruses requires both arms of the immune system: innate and adaptive. Innate immunity implies intrinsic resistance to microbes -not acquired through previous contact; it is mediated by pattern recognition receptors (PRRs) or toll-like receptors, which recognize highly conserved sequences unique to microbes like double stranded-RNA, peptidoglycans and lipopolysacharides. Acquired (adaptive) immunity requires contact with the microbe. Activation of the acquired immunity requires the prior activation of innate immunity and “antigen presentation” to T- lymphocytes (Th1 or Th2). Antigens are presented by MHC membrane proteins; Class I MHC are present in all somatic cells (except neurons and red blood cells) and Class II MHC only on APC, such as macrophages. HLA are the MHC in humans. Gray arrows represent the pregnancy-related changes on the immune response to viruses. Adapted from Straus JH & Strauss EG. Viruses and Human Diseases, 2nd edn. Elsevier, 2007.
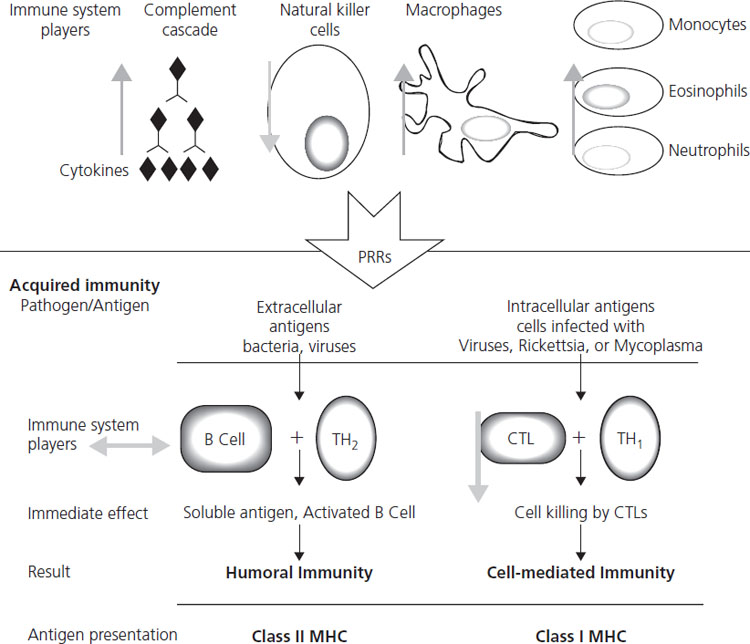
Cell-mediated adaptive immunity changes in pregnancy as well; cytotoxic T-cell function is attenuated, and newly described immunosuppressive factors such as pregnancy-specific glycoprotein 1a (PSG1a), synthesized by the trophoblast, and prostaglandin E2 (PGE2) seem to play a role [10]. The traditionally quoted pregnancy-associated change in the pattern of cytokine production by helper T-lymphocytes toward an anti-inflammatory or “Th2” response (interleukin (IL)-4, 5 and 13) instead of a proinflammatory or “Th1” (Il-2, IFN-gamma and TNF-alpha), although well documented, is not crucial for pregnancy success [12,13], whereas IFN-gamma is crucial for adequate placentation [14] and it clearly confers protection at the materno-placental level against intracellular organisms such as malaria. [15]. B-cell function and antibody production to vaccination are normal in pregnancy. Maternal IgG transfer to fetus starts by approximately 16 weeks gestation, but is not complete until approximately 32 weeks gestation.
Diagnosis of viral infections during pregnancy
Diagnosis of viral infections during pregnancy is challenging, and relies on laboratory diagnosis for mother and fetus and/or imaging the fetus and the placenta. Maternal clinical presentation is almost never pathognomonic for a particular virus; however, if the infection is symptomatic at all, it usually fits into one of the following clinical syndromes: undifferentiated fever, fever and nonvesicular rash, fever and arthritis, fever and neurologic signs, vesicular rash, hepatitis and mononucleosis-like syndrome (Table 17.2). Laboratory diagnosis relies on viral detection, either by rapid molecular diagnostic methods or by culture, and the host immune response to the virus i.e. IgM/IgG or both, usually referred to as serology.
Table 17.2 Clinical syndromes associated with viral infections in pregnancy and diagnostic tests
Traditionally, TORCH serology has been used to aid in the diagnosis of fetal manifestations of disease like IUGR or malformations, but given its poor yield and high cost, it is not widely recommended [16,17]. TORCH testing is an acronym and refers to obtaining antibody titers for Toxoplasma, syphyllis, HBV, Rubella, CMV and HSV.
Fetal ultrasound is used to follow pregnancies with confirmed maternal viral infections or to detect findings suspicious for infection (see Table 17.3). Sequential ultrasound assessment is crucial for confirmed or suspected infections, even if initial assessment is normal, to assess fetal growth and because structural abnormalities may become apparent only with further development.
Table 17.3 Common prenatal ultrasound findings of certain viral infections
Viral infections of predominantly maternal impact
Influenza A and B
Influenza is a vaccine-preventable febrile respiratory illness, which affects 10–40% of the general population over a 6-week period every winter in temperate climates, but year round in the tropics. Pregnant patients are more susceptible to developing influenza complications, particularly pneumonia. Although influenza vaccine is widely recommended for all pregnant women throughout the flu season, vaccination rates remain low.
Virology
Influenza A and B are single-stranded RNA viruses that routinely affect humans every year (seasonal influenza). Influenza A also affects other species like swine and avian. Influenza viruses undergo frequent variations in their capsular antigens, resulting in new circulating strains every year. Small, gradual changes resulting in new strains of an existing influenza subtype are called antigenic drifts. Abrupt, major changes resulting in new influenza subtypes are called antigenic shifts; these may arise from recombination of human and animal Influenza A viruses, i.e. 2009 novel H1N1 Influenza A. These antigenic changes are responsible for the yearly influenza season and major pandemics as the population has little or no immunity against new circulating strains or subtypes.
Pathogenesis
Influenza is acquired by aspiration of small-particle aerosols (<10 μm) created by sneezing, coughing, and talking [18,19]. The incubation period varies from 18 to 72 hours.
Clinical presentation
The most common clinical course is abrupt onset of fever, malaise, headache, severe myalgias and cough. Fever typically lasts 3 days and most people recover within a week. Influenza pneumonia is more common among patients with pre-existing cardiovascular diseases or pregnancy. Epidemiologic studies during the 1918 pandemic, several epidemics until 1960 and the 2009 novel H1N1 Influenza A pandemic have consistently shown that pregnant women account for an excessive proportion of influenza-related deaths [19–22]. Pregnancy is a recognized risk factor for severe illness and hospital admission for seasonal influenza, particularly among women with asthma [23]. The risk of complications increases by threefold from weeks 16 to 36 [24], but persists through the early postpartum period (up to 2 weeks) [19]. Severity of influenza seemed even higher for 2009 novel H1N1 influenza. Late initiation of antiviral treatment (>48 hours from symptom onset) increased risk of ICU admission or death by 4-fold. [19]. progressive increment on cardiac output, oxygen consumption, and changes in lung function may be contributing factors, making pregnant women with asthma, congenital heart disease or chronic renal disease especially susceptible. Myocarditis has been described associated with influenza in pregnancy.
Obstetric considerations
There are no consistent prospective data linking intrauterine exposure to influenza and increased rates of abortion, prematurity or congenital malformations [25]; maternal hyperthermia (which may in and of itself be associated with malformations) may well have been a confounding factor for most reported observations on birth defects [26,27].
Diagnosis
Accurate clinical diagnosis among healthy adults in the setting of an influenza outbreak is possible for 80–90% of cases, given the abrupt nature of symptoms. Recent data reinforces the notion that viral detection from the nasopharynx should be attempted in pregnancy and early postpartum period, but antiviral treatment must not be withheld pending laboratory confirmation (see below). Consultation with referral laboratory services regarding choice of diagnostic test is strongly advised. Rapid antigen detection methods, although widely available, were shown to have poor sensitivity during recent 2009 H1N1 pandemic. Availability of molecular detection methods (i.e. PCR) varies widely [19,28].
Treatment
Four antiviral agents are approved for influenza chemoprophylaxis and therapy: the adamantines (amantadine and rimantadine) and the neuraminidase inhibitors (the oral agent oseltamivir and the inhaled agent zanamivir). Increasing viral resistance to the adamantines and some concerning pregnancy animal data make this class of agents an undesirable option in pregnancy. Prompt initiation of neurominidase inhibitors (within 48 hours of symptoms onset) was recommended for pregnant women with suspected or confirmed influenza, in light of the recently observed benefit on survival and severity of illness [29-31].
Infection control
Patients with proven or suspected influenza illness, if admitted to hospital, should be placed on droplet (mask) and contact (gloves and gowns) precautions until day 6 from symptom onset. There is no evidence that separation of infant from mother or avoidance of breastfeeding lessens the risk of the neonate developing influenza. Adjunctive measures include hand washing and contact prophylaxis.
Prevention
Vaccination is the most effective preventative measure in healthy adults, preventing 70–90% of infections in years when circulating and vaccine viruses are antigenically similar [32]. Unfortunately, vaccine administration rates during pregnancy are low, even among pregnant women with additional risk factors for increased influenza-related morbidity, i.e. asthma, congenital heart disease or chronic renal disease. Influenza vaccination is recommended for all women who will be pregnant during the influenza season (September through January) [32,33] and has been demonstrated to reduce both maternal and infant febrile respiratory illness through 6 months of age [34]. Universal vaccination for pregnant women is likely cost-effective [35]. Healthcare workers should also be vaccinated against influenza each year to help prevent the unknowing transmission of subclinical infection to patients under their care.
Epstein–Barr virus
Epstein–Barr virus (EBV) along with VZV, CMV and HSV, belongs to the human Herpesvirus family, all double-stranded DNA viruses, distributed worldwide. It causes subclinical infection in childhood and infectious mononucleosis syndrome among teenagers or adults. As with other herpes family viruses, it results in lifelong latent infection.
Primary EBV infection during pregnancy is uncommon as only 3–4% of pregnant women are susceptible [36]. Primary EBV infection, reactivation or associated complications do not happen more often in pregnancy [37,38]. Pregnant women presenting with infectious mononucleosis-like syndrome should be investigated because acute CMV infection is the most common cause of Monospot-negative mononucleosis (Monospot is a commonly used rapid test for EBV infection; see below) and, unlike EBV, CMV can have deleterious effects on the fetus and neonate.
Pathogenesis
Epstein–Barr virus is acquired by contact with infected oral secretions. Acute EBV infection results in synthesis of large amounts of IgM against antigens common to those found on the red blood cells of other mammals, called “heterophile antibodies”; this IgM is the one measured by the Monospot test. Asymptomatic reactivation of latently infected EBV cells results in shedding of EBV particles in saliva and cervical secretions.
Clinical presentation
Typical infectious mononucleosis is characterized clinically by fever, exudative sore throat in about 33% of cases and symmetric cervical adenopathy in 80–90% of patients. Splenomegaly is present about 50% of the time. Jaundice and rashes, which may be macular (nonpalpable spots <1 cm in diameter), petechial, scarlatiniform (scarlet fever-like rash, i.e. innumerable small red papules that are diffusely distributed), urticarial (hives) or erythema multiforme-like (pink-red lesions on the extremities with a pale center), are present 5% of the time. The acute phase of the illness is serologically characterized by the transient appearance of heterophile antibodies and atypical lymphocytosis (a lymphocyte count of >50% of total white blood cells or an absolute number of >4500/μL with an atypical appearance on peripheral smear). Liver function tests are elevated in approximately 90% of cases.
Obstetric considerations
Whether EBV infection during pregnancy increases the risk of abortion remains unclear. There have been no consistent anomalies associated with gestational EBV infection, and the data do not demonstrate a causal relationship [25,39]. Data from a large pregnant cohort (n=35,940) indicate that reactivation of EBV infection during pregnancy may shorten pregnancy duration and result in lower birthweight babies [40]. However, pregnant women with acute EBV infection should generally be reassured about their probable pregnancy outcome and the most important aspect of the management of this disorder in pregnancy is to firmly establish the diagnosis to ensure that the patient does not have one of the more concerning mono-like illnesses such as CMV or toxoplasmosis.
Diagnosis
The heterophile antibody test (Monospot) is positive in approximately 85% of patients who have infectious mononucleosis (and has a specificity of close to 100%), but can be falsely negative early in the illness and may remain positive for up to 18 months after acute infection. Specific anti-EBV IgM is better for the diagnosis of recent primary infection, particularly when the Monospot is initially negative. EBV DNA detection in blood by molecular methods is available in some centers and will be positive with both acute infection and reactivation.
Treatment
Treatment is generally supportive, with hydration, analgesics, acetaminophen and rest, as primary EBV infection is usually self-limiting. A meta-analysis of five randomized, controlled trials demonstrated no significant benefit of acyclovir in the treatment of infectious mononucleosis [41]. Complications including autoimmune hemolytic anemia, severe thrombocytopenia, and respiratory compromise or severe pharyngeal edema warrant the use of corticosteroids.
Viral infections of predominantly fetal impact
Human cytomegalovirus
Cytomegalovirus (CMV) is the most common infectious cause of congenital birth defects and accounts for least 15–20% of bilateral, moderate to profound sensorineural hearing loss in childhood [42,43]. CMV infections among immunocompetent hosts are subclinical, but can be devastating for immunocompromised hosts like the developing fetus, AIDS patients, and organ transplant recipients.
Epidemiology
Cytomegalovirus infections are endemic worldwide and have no seasonal variation. Age of acquisition is younger in developing countries and among those of lower socio-economic status in industrialized countries. In the US, only 58.3% of women 15–44 years have CMV antibodies [44] but specific populations have seropositivity rates close to 85%. Annual seroconversion rates range from 2% among mid/high socio-economic background to 6% for women of lower socio-economic background and up to11% for female daycare workers [45]. Young women who care for preschool children and also become sexually active within the 2 years before delivery are at the greatest risk for delivering an infant with congenital CMV infection [46].
Virology
Cytomegalovirus is a large double-stranded DNA virus and, as all other members of the Herpesvirus family, it establishes latent infection. CMV downregulates several aspects of the immune response such as the MHC-1 pathway of antigen presentation.
Pathogenesis
The primary reservoir cell for CMV and the mechanisms by which latent CMV infection is maintained are unknown. CMV has been isolated in saliva, urine, feces, semen, vaginal secretions, breast milk, blood, donated organs and tears. Infection requires close intimate contact with persons excreting the virus in their saliva, urine or other body fluids but transmission also occurs from fomites, such as toys in the daycare setting [47].
Pathogenesis of congenital CMV infection
Congenital CMV infection refers to mother-to-child transmission of CMV in utero (transplacentally), has been defined as the presence of CMV on neonatal urine, blood or saliva in the first 10 days of life, and can potentially result in adverse neonatal outcomes and long-term sequelae, such as hearing loss (see below). Perinatal CMV infection refers to mother-to-child CMV transmission during birth or shortly thereafter from exposure to CMV-infected maternal blood, birth canal secretions or breastfeeding. Although far more common than congenital infection [48], perinatal infection is not usually associated with neonatal disease or long-term sequelae (except for extremely rare cases of pneumonitis among premature infants [47]).
Congenital CMV infection affects 0.7% of all livebirths in developed countries; 87.3% of these infections will be asymptomatic at birth [49] but late sequelae including hearing loss, learning difficulties and/or mental retardation will develop in at least 15% later in life [49]. Only a minority of congenital infections will manifest as fulminant cytomegalic inclusion disease (CID) with a reported mortality rate of 5%. CID is almost exclusively seen in infants of mothers who have had a primary CMV infection during pregnancy (see below for definition of primary infection). CID is characterized by hepatosplenomegaly, intrauterine growth restriction, hematologic abnormalities (including thrombocytopenia), rash (most classically purpura and petechiae described by the unfortunate term “blueberry muffin baby”) and CNS abnormalities including microcephaly, cerebral atrophy, chorioretinitis, ventriculomegaly and sensorineural hearing loss [47]. Intracerebral calcifications in a periventricular distribution and microcephaly [50] appear to be predictive of a poor neurodevelopmental prognosis in this condition.
The risk of congenital CMV infection is related to the type of maternal infection, classified, according to prior immune status, as primary (absence of CMV antibodies prior to pregnancy) or secondary (presence of CMV antibodies prior to pregnancy but reactivation of latent infection or reinfection with a different CMV strain [51]). Maternal immunity decreases the risk of congenital infection at least by 69% [52]. Transmission rate is 30–40% for primary maternal CMV infections versus only 1% for secondary infections. Primary infections during the first half of pregnancy are more likely to result in CNS disease and permanent disability [53].
The pathogenesis of vertical transmission for both primary and secondary infections is not fully understood [54], and the factors preventing transmission among 60–70% of all primary maternal CMV infections are still unknown. Placental CMV infection activates the maternal immune response against the cytotrophoblast by downregulating cytotrophoblast expression of HLA-G [55,56]; this may explain the reported spontaneous abortion rates. Transport of CMV virions across the trophoblast seems to involve the fetal Fc receptor [57]. Once the fetus is infected, CMV replicates in the fetal renal tubular epithelium and virions are excreted to the amniotic fluid.
Clinical presentation
Primary CMV infection during pregnancy is asymptomatic 95% of the time. When symptomatic, its clinical manifestation does not differ from that in nonpregnant hosts. Incubation period ranges from 20 to 60 days, after which a mild mononucleosis-like syndrome ensues, with fever lasting a mean of 19 days, lymphadenopathy, high lymphocyte count and abnormal liver function test results. Rare complications include hepatitis, Guillain–Barré syndrome and myocarditis.
Other obstetric considerations
In a prospective study, when compared to control women with a 2.2% spontaneous pregnancy loss rate, women with documented primary CMV infection had a 15% rate of spontaneous pregnancy loss, a sevenfold increase [58].
Diagnosis of CMV infection in pregnancy
Despite the burden of congenital CMV infection, there is no consistent approach to the diagnosis of CMV infection during pregnancy and at present, universal screening for CMV infection in pregnancy is not recommended. There is substantial controversy regarding the appropriateness of screening in the absence of reproducible and easy-to-interpret diagnostic tests or clearly effective therapies. Identifying primary infections is difficult as in most cases there is no preconception serum available to determine if seroconversion has happened. Even if seroconversion is proven and these women are offered fetal diagnosis to decide on pregnancy continuation or treatment, CMV isolation from amniotic fluid is not sufficiently sensitive before 21–24 weeks of gestational age. In addition, secondary CMV infections still pose a risk for congenital infections and there is no appropriate testing algorithm. The appropriateness of universal screening for congenital CMV infection at birth to reduce the burden of CMV-related hearing loss is being tested in a multicenter study.
Diagnosis of primary maternal infection cannot be made on clinical grounds alone, as most infections are asymptomatic, but in general, every mononucleosis-like syndrome in pregnancy should be investigated. Several definitions of maternal CMV infection during pregnancy have been used:
- seroconversion from CMV IgG-negative status pre-pregnancy or at some point during pregnancy to CMV IgG positive
- documentation of CMV IgM only, followed by both positive CMV IgM and IgG
- fourfold or more increase on CMV IgG titer during pregnancy
- low CMV IgG avidity index (see below)
- amniotic fluid positive for CMV DNA by PCR
- presence of CMV DNA in maternal blood.
Following infection, CMV IgM appears within days and persists for as long as 16 weeks. IgG can be documented as early as 2 weeks and persists for years. However, the IgG secreted during the first months after infection is of low avidity (ability to bind antigens), ranging between 20% within 3 months of infection to 70% for remote infections. Low avidity indexes have been used to diagnose primary infections [59] and a high avidity index before 18 weeks gestation has a reported negative predictive value of 100% for congenital infection [60]. However, avidity assays are not easily reproducible, nor widely available. The level of maternal antibodies at delivery does not predict protection, but the neutralizing activity of the antibody and the avidity of the IgG may be predictive [47].
Determination of CMV DNA in maternal blood, plasma or leukocytes or one of its antigens –pp65 – is commercially available and can be used to diagnose primary CMV infections, but its role in triaging and managing CMV infection during pregnancy is not defined.
Fetal diagnosis
Cytomegalovirus detection in amniotic fluid is the gold standard for prenatal diagnosis, as the virus replicates in the fetal renal tubular epithelium and viruria is consistently found in infected newborns. Reported sensitivity has ranged between 75% and 95%, but there is a several-week delay between primary maternal infection and presence of quantifiable virus in amniotic fluid. Testing for CMV in amniotic fluid by polymerase chain reaction (PCR) is not recommended prior to the 21st week of gestation because of low sensitivity, at 45% [61], or prior to 6 weeks after maternal infection [62]. Quantitative PCR of amniotic fluid and fetal blood does not predict transmission rates or likelihood of symptomatic congenital CMV infection [63]. Fetal IgM measurement is not recommended given that many CMV-infected fetuses do not develop specific IgM until late in pregnancy and because of an estimated 1% risk of pregnancy loss associated with cordocentesis.
In utero infection is suspected by maternal seroconversion or by abnormal ultrasound markers in the fetus, including symmetric growth restriction, intracranial calcifications, ventriculomegaly or echogenic fetal bowel [64].
Treatment
Currently there are no well-studied, effective therapies for CMV during pregnancy. In 2005, one prospective interventional noncontrolled study reported on the use of CMV-specific hyper immune globulin (CMV-HIG) for confirmed and/or presumed CMV primary infection among pregnant women. CMV-HIG treatment was associated with a significantly lower risk of congenital CMV infection and disease [65]. Placental thickness was found to be a marker of CMV infection and severity, and CMV-HIG was associated with significant reductions in placental size [66]. Although this was an uncontrolled study, the magnitude of benefit reported warrants further research.
Antivirals are extensively used to treat CMV among AIDS and solid organ transplant patients, but there is a paucity of data for their use in pregnancy. Anecdotal conflicting reports on the use of ganciclovir and valganciclovir for CMV infections in pregnancy [67,68], in addition to the very narrow therapeutic range from hematologic toxicity, preclude any recommendation for their use.
Prevention and education
In the absence of effective therapies for congenital CMV infection, prevention becomes very important. A live-attenuated vaccine tested among renal transplant recipients successfully induced both humoral and cell-mediated responses and reduced severity of CMV-related illness but failed to reduce infection rates. A subunit vaccine using recombinant envelope glycoprotein B demonstrated 50% efficacy to prevent CMV infection among seronegative women [69].
Women of childbearing age should be educated about CMV and its transmission and the argument can be made to target female daycare workers in particular. Counseling about handling of potentially soiled articles such as diapers and thorough hand washing when around young children or immunocompromised hosts is feasible, and has been launched recently on the CDC website. If seroconversion is documented preconceptionally, recent evidence suggests that pregnancy should ideally be delayed for 2 years [70].
Parvovirus B-19
Parvovirus B-19 infection during pregnancy is a well-recognized cause of fetal loss, nonimmune hydrops fetalis (defined as the presence of two or more of the following abnormal fetal fluid collections: polyhydramnios, skin edema, pericardial effusion, ascites or pleural effusion) and congenital anemia; however, for the majority of children and adults, this infection is benign and self-limiting, resulting in lifelong immunity, that does not require any treatment other than symptom control. Parvovirus B-19 exhibits a characteristic tropism for precursor erythroid cell lines, inhibiting red blood cell production transiently. As a consequence, the infected fetus may be severely affected because red blood cell turnover is high and the immune response relatively immature. Pregnant women can become infected with parvovirus B-19 as these infections are commonplace in the community and occur year round, spreading primarily by respiratory secretions.
Virology
Parvovirus B-19 is a small single-stranded DNA virus, belonging to the large Parvoviridae family. Infection results in long-lasting immunity elicited by its two capsid proteins, VP2 and VP1. It cannot be cultivated in the laboratory and diagnosis relies on serology and molecular detection methods.
Epidemiology
Thirty-five to 45% of women of childbearing age are susceptible to parvovirus B-19 infection and their annual seroconversion rate is 1.5% [71]. Higher attack and annual seroconversion rates are seen for workers in close contact with affected children, such as daycare providers and school personnel [72]. In the USA, Canada and Europe the annual incidence of acute B-19 infection in pregnancy is approximately 1–2% but may rise to >10% during epidemics; conservative estimates indicate that up to 3000 pregnancies are lost every year from B-19 infection in the US [73].
Pathogenesis
Adult patients acquire parvovirus B-19 from personal contact via aerosol or respiratory secretions and from contaminated blood products. The fetus can be infected if the mother is nonimmune at the time of exposure and she becomes viremic with parvovirus B-19, resulting in transplacental transmission. In this viral infection, transmission appears to be poorly blocked by the placenta and can occur throughout gestation.
Parvovirus B-19 binds to the P blood group antigen expressed by late erythroid cell precursors and other cells, such as synovium, cardiac endothelium, liver and lung, resulting in an acute but self-limited (4–8 days) cessation of red cell production and a corresponding decline in hemoglobin level. Among hosts with high red cell turnover, such as the fetus, this temporary failure of erythropoiesis can precipitate profound anemia and the equivalent of an aplastic crisis. By day 7 of infection, viremia peaks and IgM becomes detectable; thereafter, viral replication is contained by cell-mediated immunity driven by Th1 lymphocytes. Immune complex deposition is very common and may explain some clinical manifestations of parvovirus B-19 infection such as the polyarthritis seen in adults (see below).
Pathogenesis of mother-to-child transmission
The factors contributing to the fetal morbidity of parvovirus B-19 infection during pregnancy include the following.
- P antigen levels are highly expressed in the villous trophoblast and placental tissues during the first trimester, declining as the pregnancy progresses to undetectable levels by the third trimester [74].
- The red blood cell turnover during the hepatic stage of fetal hematopoiesis is very high during weeks 8–20 of gestation.
- The passive transfer of maternal IgG is not effective prior to week 25 of gestational age.
The maximal risk of vertical transmission is around day 7 of infection, when maternal viremia reaches its peak and IgM antibodies appear [75]. The most hazardous time for maternal infection is the early second trimester. Fetal myocardial cells express the P antigen and fetal myocarditis from parvovirus B-19 infection has been documented in the third trimester.
Clinical presentation
Parvovirus B-19 infection in pregnancy does not differ from the nonpregnant adult, but given its nonspecific symptoms and the potentially deleterious consequences to the fetus, laboratory diagnosis is recommended [76].
Following a 1-week incubation period, 80% of susceptible adults will develop a biphasic illness. The initial viremic phase is characterized by fever (rarely exceeding 39°C), malaise, myalgias and pruritus coinciding with viral shedding from the upper respiratory tract and appearance of anti-parvovirus B-19 IgM (anti-VP2). The immune complex-mediated stage (days 17–19 from initial infection) consists of rash and symmetric arthritis of the small joints of the hands, wrists, knees or ankles, seen more commonly in women. However, in many cases the symptoms are nonspecific and not recognized as parvovirus infection.
Obstetric considerations
Following exposure of a nonimmune pregnant woman, fetal infection is only a concern if the woman becomes infected. Then, the intrauterine transmission rate is 20–50% [77,78]. Despite this high rate of intrauterine transmission, many in utero fetal infections do not result in fetal disease [77]. Parvovirus B-19 is not teratogenic and does not result in structural anomalies in the fetus [79]. If infection results in fetal death, this usually occurs 4–6 weeks after maternal infection but has been reported at up to 12 weeks. Although fetal deaths have been reported in every trimester, the highest risk of fetal loss seems confined to the first 20 weeks of pregnancy and averages 9% [80]. Third-trimester intrauterine fetal death has been documented mostly in the absence of hydrops [81] and death has been postulated to result from fetal arrhythmias in the setting of myocarditis [82].
Nonimmune hydrops fetalis
The interval between parvovirus B-19 infection and development of nonimmune fetal hydrops ranges from 2 to 6 weeks, but up to 12 weeks in some studies [83]. Nonimmune fetal hydrops is characterized by marked fetal ascites, cardiomegaly, and pericardial effusions and, in advanced stages, generalized edema and hydropic placenta. The mechanism is severe fetal anemia, which leads to high output cardiogenic heart failure, more often during the hepatic stage (8–20 weeks gestation) of fetal hematopoiesis, when the half-life of erythrocytes is at its shortest [84]. Risk of B-19-induced hydrops fetalis is 3.9% throughout pregnancy, with a maximum of 7.1% when infection occurs between 13 and 20 weeks [85].
Diagnosis
The diagnosis of parvovirus B-19 infections during pregnancy and associated complications is a multistep process, including determination of maternal seroconversion, noninvasive fetal assessment to determine presence of fetal anemia with middle cerebral artery peak systolic velocity (MCA-PSV), and fetal blood sampling via cordocentesis for transfusion as necessary. Women infected with parvovirus B-19 (seroconverters) should be referred to a tertiary center with capability for MCA Doppler assessments or level II ultrasound capabilities for fetal assessment and surveillance, as weekly follow-up until 10–12 weeks after maternal exposure is advisable.
Pregnant women exposed to parvovirus B-19 should be tested for presence of parvovirus B-19 IgG to determine immune status. If this is negative, i.e. the woman is nonimmune and susceptible, then IgG should be repeated 2 weeks later to determine if seroconversion has occurred, i.e. she has become infected. In general, maternal recent infections (less than 1 month) are characterized by parvovirus B-19 IgM presence, high IgG titers and/or parvovirus B-19 DNA on maternal blood by PCR. At this stage, fetal complications, even if absent, can still develop [86], stressing the need for close fetal surveillance.
Fetal anemia results in a hyperdynamic pattern of blood flow in the middle cerebral artery due to the early response of brain tissue to anemia. MCA-PSV can be a very sensitive measure to identify fetal anemia secondary to parvovirus B-19 infection [87,88]. Weekly MCA-PSV measurements permit planning for cordocentesis to assess for actual fetal anemia and, if present, for fetal blood transfusions. Centers experienced in interpretation of MCA-PSV measurements and gestational age-specific comparisons should be accessed for accurate use of this tool.
Management
Opportune intrauterine transfusion (IUT) corrects fetal anemia and may reduce the mortality of B-19 infection significantly but there are no prospective data on the ideal timing to do so. Most clinicians proceed with transfusion if the fetus is anemic, even if there is already evidence of recovery of erythropoiesis by a high reticulocyte count. The neurodevelopmental prognosis of children surviving IUT for parvovirus B-19 is generally good, but some cases of delayed neurodevelopment have been associated with resolved parvovirus B-19 in utero infections. However, this does not seem to be related to the severity of fetal anemia and acidemia [89].
Prevention
There is no reasonable strategy to avoid B-19 exposure of pregnant women as exposure usually happens before the index case develops the rash, if symptomatic at all. For occupational exposures, the risk of occupational infection may be similar to or less than that in the community or at home. As a result, women in occupations such as school teaching do not warrant routine screening. However, if school-based outbreaks occur, selective screening may be appropriate.
Rubella
Rubella is a vaccine-preventable disease that usually causes a mild febrile rash in children and adults but it is a clearly recognized teratogenic agent. Congenital rubella syndrome (CRS), a rare occurrence in developed countries thanks to the childhood vaccination programs established 30 years ago, remains a significant cause of deafness, mental retardation and blindness in developing countries.
Virology
Rubella is a single-stranded RNA enveloped virus, with projecting glycoproteins E1, E2, and C. These glycoproteins do not undergo mutations, which makes natural and vaccine immunity attainable and long lasting.
Epidemiology
Rubella immunization dramatically decreased the incidence of infection and CRS in the United States, Canada and Western Europe. Although only a few hundred cases of rubella and less than 50 cases of CRS are reported each year in the US, up to 10% of women of reproductive age remain susceptible to rubella, in particular if they emigrated from countries where immunization has not been widely available. In developing countries 15–20% of women of reproductive age remain susceptible to rubella infection and a minimum of 100,000 cases of CRS happen every year worldwide [90].
Pathogenesis
Rubella infection is acquired by inhalation of virus particles; infection of upper respiratory tract cells is followed by lymphatic spread and subsequent viremia. The virus is detectable in blood and upper respiratory secretions 1 week before onset of symptoms. The rash develops at the same time that antibodies become detectable and viremia is cleared. Rubella-specific IgM usually persists for 8–12 weeks, but may be detected for up to a year. Immunity to rubella is lifelong, but a few cases of asymptomatic reinfection have been demonstrated [91].
Obstetric considerations
The outcomes of rubella infection during pregnancy include placental infection without fetal infection, both placental and fetal infection, resorption of the embryo, spontaneous abortion, stillbirth and congenital rubella syndrome.
Gestational age at the time of maternal rubella infection is the most important determinant of intrauterine infection risk and CRS [92–94]. Intrauterine infection (not a synonym of CRS) follows a bimodal distribution: 60–90% during the first trimester, 25% during the second trimester and 60–100% for infections acquired during the third trimester. However, the risk of CRS is confined to the first 20 weeks of pregnancy [95], as follows: 90% for maternal infections acquired prior to week 11, 33% for infections during weeks 11–12, 11% for weeks 13–14, and 24% for weeks 15–16.
Intrauterine infection arising from maternal reinfection has been the subject of much debate, but is considered very unlikely [96,97].
Clinical presentation
The clinical presentation and severity of rubella infection during pregnancy do not differ from nonpregnant adults; in general, 25% of infections are subclinical.
After an incubation period of approximately 2 weeks, adults develop fever and malaise, followed by a maculopapular rash (flat discolored areas and small raised lesions <1 cm in diameter) that starts on the face and gradually moves down to the feet. The most common complication is self-limited arthritis reported in up to 60% of women from natural infection and in 40% of women after vaccination; other complications include postinfectious encephalopathy (1:6000 cases), transient thrombocytopenia (1:3000), purpuric rash, and very rarely hemolytic anemia and Guillain–Barré syndrome [98].
Diagnosis
Acute rubella in pregnancy
Investigation of all rubella-like illnesses (fever, rash +/−arthralgias) in pregnancy is mandatory, as several other viruses have a very similar clinical presentation, including parvovirus B[19, measles, EBV, roseola, and some enteroviral infections, and yet the consequences for the fetus and the pregnancy can differ substantially among them (see Table 17.2).
In the right clinical context, acute infection is diagnosed by detection of rubella-specific IgM in one serum sample or by a fourfold increase in rubella antibody titers in paired samples (acute and convalescent) using the same assay [99]. Nevertheless, IgM may persist for up to a year after acute infection or vaccination and false-positive IgM results may arise from cross-reactivity with rheumatoid factor and other infections. IgG avidity tests (see explanation in preceding section on testing for CMV infection in pregnancy), where available, are helpful in these situations and a second rubella-specific IgM determination using a different assay is recommended as well, especially in the first 20 weeks of pregnancy [98]. Knowledge of local clinical and laboratory capabilities is critical when interpreting results, especially if pregnancy termination is being considered.
Prenatal diagnosis of fetal infection
Many cases of CRS do not have characteristic ultrasound findings. Viral detection by molecular technology (RT-PCR) in amniotic fluid is the preferred method for prenatal diagnosis of fetal infection, and has a reported sensitivity of 87–100% [100,101], when done at least 6–8 weeks after maternal infection to avoid false-negative results. Rubella virus isolation by chorionic villous sampling is used at some centers but might be misleading, as placental infection does not imply fetal infection [99].
Exposure to rubella during pregnancy
All nonimmune pregnant women exposed to close contacts of rubella or rubella-like illnesses should have rubella IgG paired serum samples (at least 2 weeks apart).
Management
There is no specific treatment for rubella infection, and postexposure prophylaxis with immunoglobulin for susceptible women is not recommended as there is no solid evidence that it prevents rubella infection. The US Centers for Disease Control and Prevention (CDC) does support its use in pregnancy only for those susceptible women who have been exposed to rubella who will not consider a termination under any circumstances. Although the immunoglobulin may ameliorate maternal symptoms to some degree, patients need to be informed that this does not imply that the fetus has been protected from infection [102].
Prevention
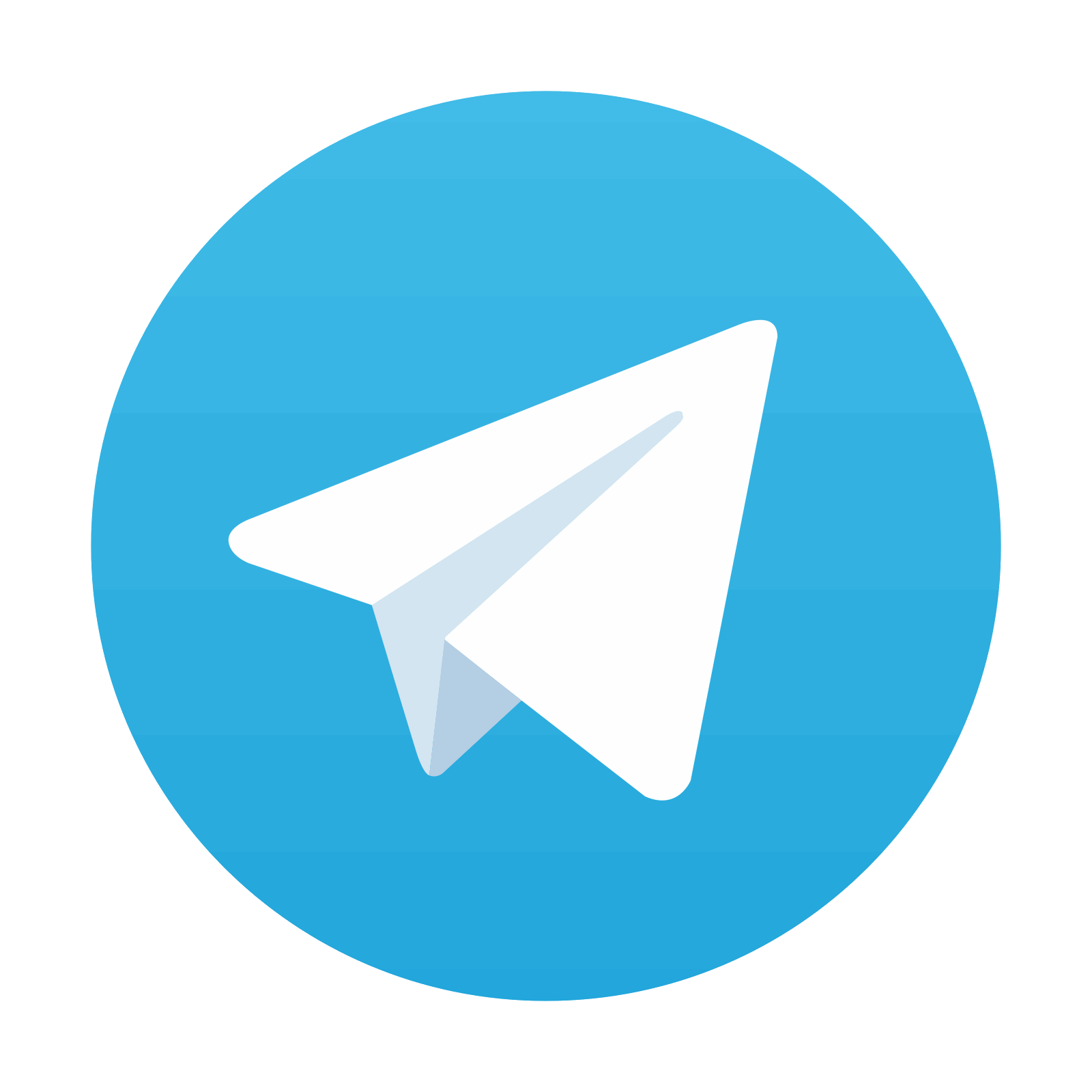
Stay updated, free articles. Join our Telegram channel

Full access? Get Clinical Tree
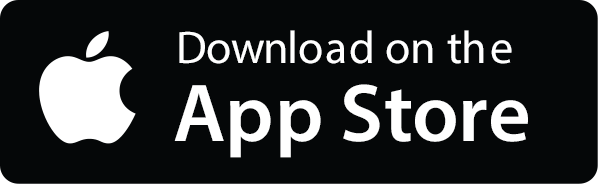
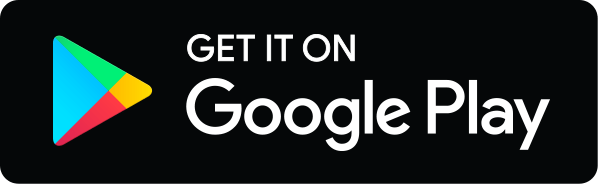