KEY POINTS
- 1.
Hypoxic-ischemic encephalopathy (HIE) is a major cause of neonatal morbidity and mortality. More than 50% of all infants with HIE will die or have severe neurologic disabilities by the age of 2 years.
- 2.
The need to institute therapies such as whole-body hypothermia in a timely fashion make rapid identification of neurologic injury critically important.
- 3.
The focus of biomarker development in neonatal brain injury has been on the identification of moderate to severe injury, which is most likely to be treatable.
- 4.
Glial biomarkers such as glial fibrillary acidic protein (GFAP) holds the most promise. GFAP is a cytoskeletal intermediate filament protein seen mainly in astroglial cells.
- 5.
A single biomarker may not be sufficient to estimate the severity of a multifaceted complex disease such as HIE or traumatic brain injury. A combination with other potential brain injury biomarkers or with clinical tools may help.
Worldwide it is estimated that 1.15 million babies develop hypoxic-ischemic encephalopathy (HIE) every year. Up to 60% of infants with HIE will die or have severe disabilities by the age of 2, including mental retardation, epilepsy, and cerebral palsy. The costs related to HIE exceed $11 billion annually in the United States. HIE is defined by a constellation of symptoms in the neonate, but no definitive diagnostic test is available. One of the greatest challenges in perinatal medicine is assessing the fetus during labor and the neonate shortly after birth for evidence of brain injury. The presence of meconium, nonreassuring fetal heart rate tracing, Apgar scores, umbilical artery blood gases, and physical exam are tools currently used to identify brain injury in the fetus and neonate, but all of these lack precision.
The availability of therapies such as whole-body hypothermia, which must be instituted within 6 hours of birth, make the rapid identification of a baby with neurologic injury critically important. How to rapidly and objectively identify the fetus and neonate with brain injury may be solved by borrowing an approach from traumatic brain injury research. Extensive effort has been applied in the field of traumatic brain injury to identify acute blood biomarkers as diagnostics to identify these patients, discriminate severity, and monitor treatment efficacy and as prognostics for recovery and long-term disabilities. Although urine can be obtained noninvasively, and due to its rich source of proteins may contain many potential biomarkers, severely asphyxic newborns shunt blood away from their kidneys, which can lead to acute renal injury and oliguria, making interpretation of urinary biomarkers difficult. Biomarkers of brain injury can also be obtained from cerebrospinal fluid (CSF); however, obtaining CSF is more invasive and potentially complicated than obtaining blood and is not amenable to serial sampling.
The focus of biomarker development in traumatic brain injury has been on the identification of moderate to severe injury ; however, the greatest potential impact of blood biomarkers to change clinical practice in perinatology is for mild injury because diagnostic and prognostic challenges presented for mild injuries are more difficult to identify and monitor. There is no current standard therapy for mild injury, and increasingly late follow-up suggests that mild injury results in identifiable pathology in children.
It is unlikely that a single biomarker will reflect the full picture of the injured brain for a multifaceted, complex disease such as HIE or traumatic brain injury. Combining biomarkers from multiple cellular pathways has shown superior sensitivity and specificity in the identification of traumatic brain injury. Simultaneous measurements of neuronal and glial biomarkers may complement each other to identify distinct injury mechanisms and determine the timing of injury. A study of severe traumatic brain injury found that glial biomarker elevations are primarily a reflection of focal mass lesions and that diffuse injuries primarily result in neuronal biomarker elevations. Because patients with diffuse injuries may require different therapies than patients with focal lesions, such a combination of biomarkers may enable us to select patients for targeted therapies. , In perinatal medicine this might allow differentiation of large major vessel stroke from more diffuse hypoxic-ischemic injuries. The ideal biomarker panel may include multiple biomarkers produced by different brain cell types, and currently available multiplex immunoassay platforms make it possible to measure up to 10 biomarkers with a high degree of sensitivity from small volumes of plasma. Investigators have proposed developing a point-of-care handheld device that can quickly and accurately measure brain injury biomarkers, similar to the handheld dextrometer for diabetic patients that can measure a glucose level within seconds.
Optimally, the level of the brain injury biomarkers should correlate with the size, location, and severity of the lesion, clinical outcome, and response to treatment. Ideally, serum biomarkers should provide information on the pathophysiology of injury, improve stratification of patients by injury severity, assist in the monitoring of secondary insults and injury progression, monitor response to treatment, and predict functional outcome. Circulating brain injury biomarker levels in neonatal HIE could indicate brain injury and reflect the extent of damage, solving a clinical dilemma in the discrimination of mild versus moderate-severe injury. Neonatal brain injury is also complex, with the initial hypoxic-ischemic injury followed by secondary injury, which maybe inflammatory in nature. Biomarkers that can discriminate acute injury and interrogate secondary injury are likely to be the most helpful for outcome prognostication and discriminating treatment effects ( Box 51.1) .
- •
Early change in blood levels that show mild-moderate injury in its early phase, which might be potentially treatable
- •
Combinations of neuronal and glial biomarkers might improve sensitivity and specificity
- •
Combinations of biomarkers from multiple cellular signaling pathways might improve sensitivity and specificity
- •
Ease of measurement, possibly with a portable, handheld device
- •
Blood change linearly with increasing severity of injury
- •
Identify secondary insults and injury progression
- •
Identify response to treatment and predict eventual outcome
- •
Clear understanding of the significance of levels in blood versus the cerebrospinal fluid
Because currently there is no gold standard for diagnosing mild traumatic brain injury, not even by conventional assessment through neuroimaging techniques, these approaches have been applied to identify brain-specific markers of injury ( Fig. 51.1) . During brain injury, neural proteins or their breakdown products are released into the extracellular environment, reaching the CSF in relatively high concentration and the bloodstream via the compromised blood-brain barrier. In premature infants in particular, the blood-brain barrier is particularly fragile, potentially increasing the likelihood that brain proteins may reach the circulation after injury. The clearance and half-life of the biomarkers contribute to the final concentration that can be measured in blood.

Of the numerous candidate biomarkers for traumatic brain injury, glial fibrillary acidic protein (GFAP) holds the most promise. One of the main strengths of GFAP as a brain injury biomarker is that it is only found within the central nervous system. GFAP is a cytoskeletal intermediate filament protein that forms networks that support astroglial cells and is found only in the astroglial cytoskeleton. Astrocytes are important to brain injury because their foot processes compose part of the blood-brain barrier, and with disruption after injury, astrocyte damage results in early release of GFAP. , GFAP levels peak at 1 to 2 days following severe brain trauma and are normal in patients with other trauma that does not include traumatic brain injury, an indication of GFAP’s brain specificity. In neonates, serum GFAP levels have been shown to be significantly elevated at the time of birth and during the first week of life in term and near-term infants with HIE who have abnormal brain magnetic resonance imaging (MRI) scans at 1 week of life and in premature neonates who develop periventricular white matter injury. In addition, GFAP may provide insights into the pathobiology of therapeutic hypothermia because significant elevations in GFAP occur after rewarming from therapeutic cooling for HIE in the neonates that later have an abnormal MRI, suggesting that the neonates with severe injury manifest reperfusion injury after therapeutic cooling. This may offer an opportunity to discriminate mild from moderate to severe HIE and triage high-risk neonates to evolving adjunctive therapies.
Blood biomarkers discovered in traumatic brain injury could significantly improve the management of neonates with HIE, particularly those with mild and moderate injury, by providing more accurate early diagnosis and prognosis and by monitoring therapies in the acute care setting. Biomarkers could help determine the severity and mechanism of injury and quantitatively measure injury progression, which would provide major opportunities for clinical research.
Several potential epilepsy biomarkers have been proposed in recent years, including blood biomarkers of inflammation, blood-brain barrier damage, and brain injury. Given the complexity of epilepsy, it is unlikely that a single biomarker is sufficient for predicting epileptogenesis, but a combinatorial approach may be able to identify appropriate biomarkers at different stages of the evolution of the disease.
The pathology of perinatal HIE is very heterogeneous, and one “magic” biomarker may not be the solution, but a panel of biomarkers may prove to be most useful in distinguishing the different pathologic-anatomic processes that compose the injury. The brain consists of many elements, and depending on the mechanism and severity of injury, various damage patterns may be reflected by different combinations of biomarkers. Biomarkers will probably supplement existing tools such as the Glasgow Coma Scale and neuroimaging for the initial classification of brain injury in the near future. Because the hypoxic injury pattern on MRI is not diagnostic for 7 to 14 days, a blood biomarker that could fill the clinical gap for predicting current and worsening neurologic status or long-term disability would have great clinical utility. With the combinations of different pathophysiology related to each biomarker, a multibiomarker analysis would seem to be the most effective way to assess brain injury and would likely increase diagnostic accuracy.
Blood biomarkers offer an objective and quantitative way to identify and follow a neonate with brain injury. They could be used to triage neonates to the current standard of 72 hours of hypothermia as well as investigational therapies such as erythropoietin, xenon gas, melatonin, and stem cell treatment. Blood biomarkers could possibly provide information about the extent of injury in the acute phase before ultrasound or MRI can identify abnormalities and determine the timing of injury as well. Rather than imprecise measures of fetal and neonatal brain injury, such as fetal heart rate abnormalities, meconium, cord gas at delivery, and Apgar scores, a multiplex combining glial and neuronal biomarkers could provide objective evidence of the extent and pattern of injury that would lead to improved identification of the brain-injured fetus or neonate and triage to appropriate therapy after birth and would serve as an objective measure of the effectiveness of treatment.
Applications for circulating brain injury biomarkers may also become clinically relevant in the future for infants who suffer severe cardiopulmonary failure or cardiac arrest in the neonatal period. Interventions employed in the neonatal intensive care unit may contribute to additional risk of neurologic injury in these scenarios. One such intervention is extracorporeal life support in the form of extracorporeal membrane oxygenation (ECMO) used in the neonatal period for infants with severe refractory persistent pulmonary hypertension of the newborn, meconium aspiration syndrome, congenital diaphragmatic hernia, congenital heart disease, and other more rare indications. Over 30,000 neonatal ECMO cases have been reported to the Extracorporeal Life Support Organization (ELSO) registry from inception in 1989 to July 2019. Although the number of neonatal ECMO cases has stabilized, there has been a continued and steady increase in the number of cardiac and extracorporeal cardiopulmonary resuscitation neonatal ECMO cases reported to the ELSO registry in the past decade. Despite this increase in the total number of ECMO cases, survival in the past 5 years has remained stable at 68% for neonatal respiratory ECMO cases, 50% for neonatal cardiac ECMO cases, and 43% for neonatal extracorporeal cardiopulmonary resuscitation cases. ECMO is associated with high risk for neurologic injury, including intracranial hemorrhage and stroke. Both complications have been shown to be associated with reduced survival. Therefore, neurologic monitoring is paramount for prompt diagnosis of brain injury with implementation of neuroprotective interventions, and after ECMO decannulation, of rehabilitation interventions. Several neurologic monitoring methods have been deployed in neonatal ECMO patients with varying success, including daily head ultrasounds, amplitude integrated electroencephalography, standard or continuous electroencephalography, transcranial Doppler ultrasound, cerebral oximetry monitoring, and circulating brain injury biomarkers. Serial monitoring of brain-specific proteins circulating in blood has been shown to be associated with abnormal neuroimaging findings during or immediately after ECMO support, with survival after critical illness requiring ECMO support, and with neurofunctional status at hospital discharge in former ECMO patients. , Given the heterogeneity of injury encountered during ECMO support, efforts were made to develop panels of brain injury biomarkers in this population that have the ability to reflect neuronal injury (e.g., neuron specific enolase [NSE], brain derived neurotrophic factor), astrocytic injury (e.g., GFAP, S100b), and neuroinflammation (e.g., monocyte chemoattractant protein 1 [MCP1], intercellular adhesion molecule-5). In a study of 80 neonatal and pediatric patients, the performance characteristics for a combination of biomarkers of brain injury included an area under the receiver operating characteristic curve that reached significance for GFAP, MCP1, NSE, and S100b (0.71, 0.64, 0.68, and 0.66, respectively). A model for unfavorable outcome prediction that removed collinear biomarker predictors yielded an improved area under the curve of 0.73 for the combination of GFAP and NSE. It is foreseeable that neonates requiring intensive care interventions will, in the future, be monitored with serial measurements of circulation brain injury biomarkers during periods of high risk for injury, including ECMO, cardiopulmonary bypass for congenital heart disease repair, or after cardiac arrest.
REFERENCES
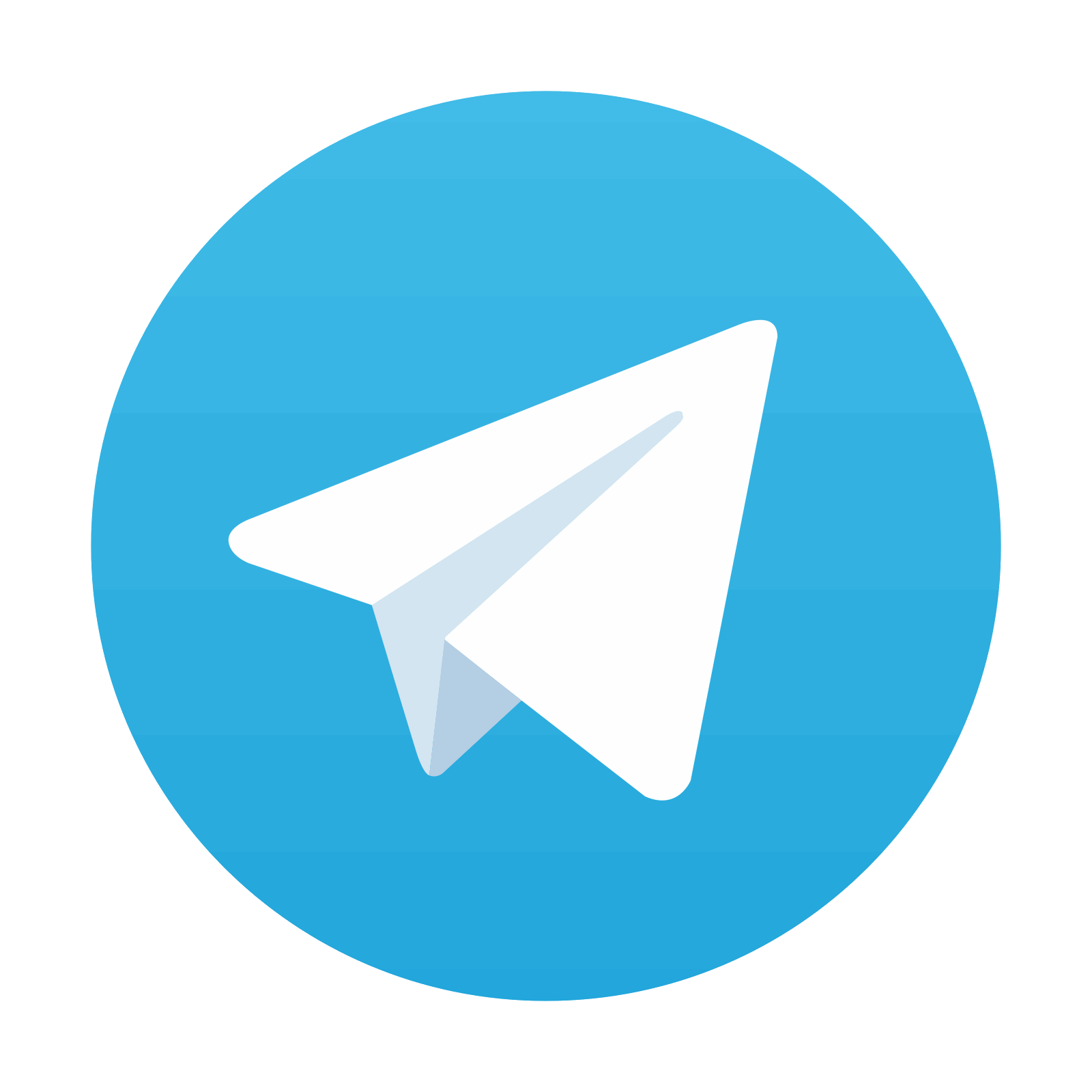
Stay updated, free articles. Join our Telegram channel

Full access? Get Clinical Tree
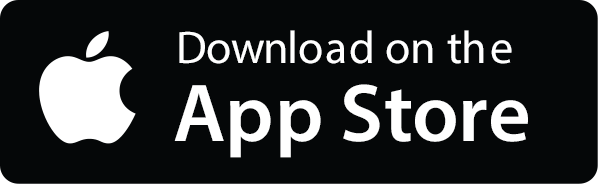
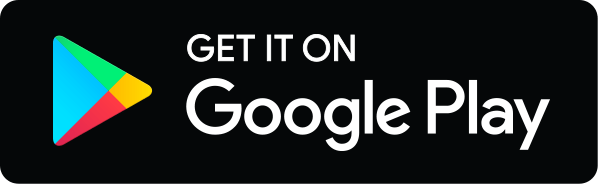
