KEY POINTS
- 1.
Genomic medicine has emerged as a new discipline to analyze the human genome and genetic information as a part of clinical care.
- 2.
There are various types of molecular techniques to detect various genetic variations. Each method has unique strengths and limitations, from conventional karyotyping to genome sequencing.
- 3.
Clinicians needs to understand the limitations of the methods used in genetic testing to know what test to order and how to interpret a nondiagnostic test result.
- 4.
Genomic testing ideally should be performed rapidly to have the greatest impact on neonatal management.
Diagnostic Techniques in Clinical Genetics
There are many methods used in clinical genetic testing, each of which has strengths and limitations. These methods are applied to particular clinical-use cases such as newborn screening, carrier testing, noninvasive prenatal screening, presymptomatic testing, diagnostic testing, and pharmacogenomics. Each method is optimized to address a specific type of genetic variation, and the methods are somewhat overlapping and largely complementary. Tandem mass spectrometry (MS) has a significant role in expansion of newborn screening. A karyotype is used to detect aneuploidies, large deletions or duplications, and chromosomal rearrangements. Fluorescence in situ hybridization (FISH) detects microdeletions and microduplications (although with less fidelity) but requires a correct hypothesis about which probe to use to detect the cytogenetic anomaly. By contrast, a chromosomal microarray (CMA) is hypothesis free and provides high resolution to detect small copy-number variants throughout the genome in a single test. Sanger sequencing was the traditional method to sequence and identify single-nucleotide variations and insertions/deletions of a few bases. With the development of next-generation sequencing (NGS) and massive parallel sequencing, the cost/base of sequencing decreased and throughput increased dramatically, making feasible the sequencing of panels of genes to diagnose genetically heterogeneous diseases for conditions such as cardiomyopathies and hereditary cancer. Exome sequencing (ES) assesses only coding regions of most genes in the genome whereas genome sequencing (GS) assesses both coding and noncoding regions. ES and GS offer versatility and can be used to assess sequence-level variants and, with appropriate analysis copy number, variants and structural variants in the case of GS.
A summary comparing the different clinical genetic testing methods is provided in Table 77.1 .
Karyotype | Chromosome SNP Microarray | FISH | Sanger Sequencing | Sequencing Panel | ES | GS | |
---|---|---|---|---|---|---|---|
Single-nucleotide variations (SNVs) | X | X | X | X | |||
Copy number variations (CNVs) | X | X | ± | X | |||
Balanced chromosomal rearrangement | X | X | ± | ||||
Identification of new disease genes | X | X | |||||
Incidental findings | X | X | |||||
Cost | Low | Low | Low | Low | Low | High | High |
Chromosomal Disorders and Karyotyping
Chromosome disorders are an important category of genetic disease, occurring in approximately 1 of every 150 live births. They are a common cause of intellectual disability and pregnancy loss. Chromosomal disorders can be divided into two groups: numerical and structural abnormalities. Numerical abnormalities result from the gain or loss of one or more chromosomes, referred to as an aneuploidy (e.g., trisomy, monosomy, or tetrasomy), or the addition of one or more complete haploid genomes, referred to as polyploidy (e.g., triploidy, tetraploidy).
Structural chromosome abnormalities can be unbalanced (the rearrangement causes a gain or loss of chromosomal material) or balanced (the rearrangement does not produce a loss or gain of chromosome material). Unbalanced abnormalities of chromosomes cause congenital anomalies and neurodevelopmental disorders more commonly than do balanced rearrangements. Structural alterations can be caused by translocations (reciprocal or Robertsonian translocations), ring chromosomes, insertions, deletions, or complex rearrangements.
Mosaicism
Mosaicism is the presence of at least two cell populations derived from the same zygote. Mitotic nondisjunction, trisomy rescue, or occurrence of a somatic new mutation can lead to the development of genetically different cell lines within the body. Mosaicism is possible for any type of genetic change, from a chromosome to a single nucleotide. Mosaicism can affect any cells or tissue within a developing embryo at any point after conception through adulthood. In gonadal mosaicism, the mosaic cells are restricted to the gonads and do not have a clinically observable phenotype but can be passed on to multiple progeny in the next generation. If the mosaic cells are found only in the placenta and absent in the embryo, this is known as confined placental mosaicism, which may be detectable on a chorionic villus sample and may be associated with intrauterine growth restriction but not with congenital anomalies, neurodevelopmental disorders, or any other phenotype if the genetic anomaly is not present in the fetus.
Aneuploidy
Aneuploidy refers to missing or additional chromosomes that do not represent a multiple of 23 chromosomes. Trisomies of chromosomes 13, 18, and 21 are among the most clinically important chromosome aneuploidies present in live-born infants. Monosomy (only one copy of a chromosome in an otherwise diploid cell) is much less common and more severe than trisomy (three copies of a chromosome) and is rarely viable. Nondisjunction causes errors in chromosome segregation and is the cause of aneuploidies. Multiple congenital anomalies, growth restriction, and intellectual disability are the most common phenotypes associated with trisomies.
Each of the common trisomies has a distinct neonatal phenotype that is recognizable by an experienced clinician. Trisomy 13 and 18 are both less common than trisomy 21, and survival beyond the first year is rare for trisomy 13 and 18. In contrast, individuals with Down syndrome usually have a life expectancy greater than 50 years. Most other autosomal trisomies result in early pregnancy loss, with trisomy 16 being a particularly common finding in first-trimester spontaneous miscarriages.
Trisomy 21, or Down syndrome, is the most common autosomal aneuploidy seen among live births. Approximately 95% of Down syndrome cases are caused by nondisjunction, and Robertsonian translocations cause most of the remainder. Mosaicism is seen in 2% to 4% of Down syndrome cases and is often associated with a milder phenotype. The most frequent cause of mosaicism in trisomies is a trisomic conception followed by loss of the extra chromosome during mitosis in some embryonic cells (trisomic rescue).
Trisomy 13 and 18 are sometimes compatible with survival to term, although 95% or more of affected fetuses are spontaneously aborted. These trisomies produce more severe disease than trisomy 21, with 90% to 95% mortality during the first year of life.
Turner syndrome is a sex chromosome aneuploidy and is most commonly associated with 45,X. Although this disorder is common at conception, it is relatively rare among live births, reflecting a high frequency of spontaneous abortion. Mosaicism increases the probability of survival to term. Klinefelter syndrome (47, XXY) is the most common disorder of sex chromosomes, with a prevalence of 1 in 500 in males. It is associated with mild phenotypic characteristics and infertility. The most common features are summarized in Table 77.2 .
Syndrome | Karyotype | Main Clinical Features |
---|---|---|
Down | Trisomy 21 | Single palmar crease, hypotonia, intellectual disability, open mouth with large tongue, upslanting palpebral fissures, epicanthal folds, depressed nasal bridge, short neck, congenital heart disease (atrioventricular canal defects) |
Edwards | Trisomy 18 | Clenched hands with overlapping fingers, prominent heels, prominent occiput, low-set malformed ears, micrognathia, small eyes, mouth, and nose, severe intellectual disability, congenital heart disease, horseshoe kidney |
Patau | Trisomy 13 | Cleft lip and/or palate, clenched hands, intellectual disability, polydactyly, congenital heart disease, midline brain anomalies, genitourinary abnormalities |
Turner | 45,X | Short stature, webbed neck, congenital heart disease (coarctation), widely spaced nipples, low hairline, premature ovarian failure |
Klinefelter | 47,XXY | Tall stature, small testes, azoospermia/infertility, gynecomastia |
Triple X | 47,XXX | Female with normal genitalia and fertility, at risk for educational and behavioral problems, early menopause |
Karyotype is a method of visualizing the chromosomes ( Fig. 77.1 A ). There are many staining techniques, but Giemsa staining (G-banding) is the most common. The conventional metaphase karyotype has a resolution of 400 to 550 bands; however, a high-resolution prometaphase karyotype can resolve up to 860 bands. Imbalances greater than 5 to 10 Mb are generally visible on a karyotype. Chromosome structures such as supernumerary (marker) chromosomes (derivative chromosomes such as ring or isochromosomes) are most easily identified with karyotyping.

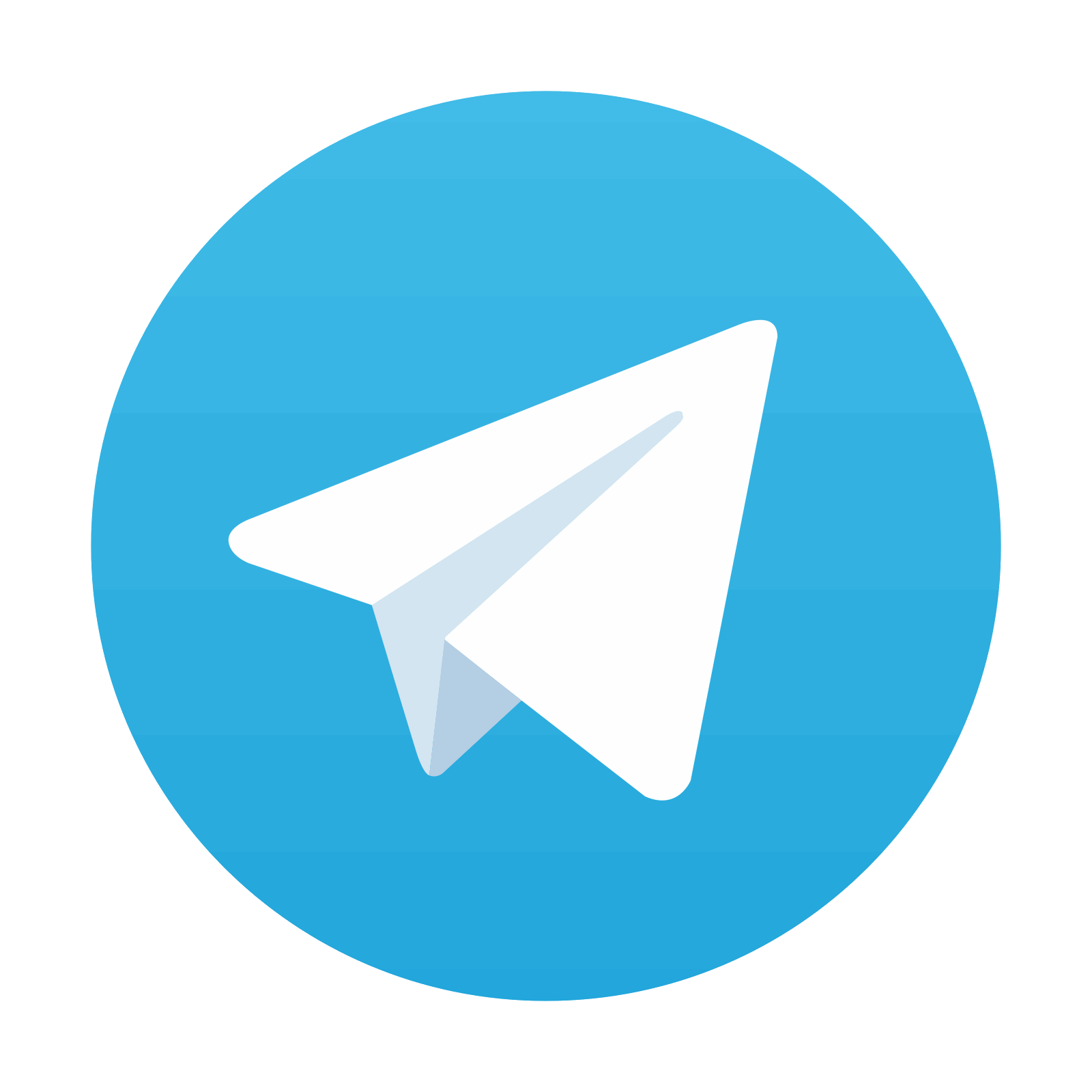
Stay updated, free articles. Join our Telegram channel

Full access? Get Clinical Tree
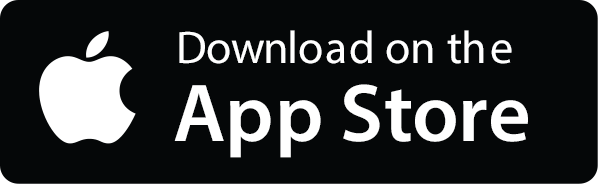
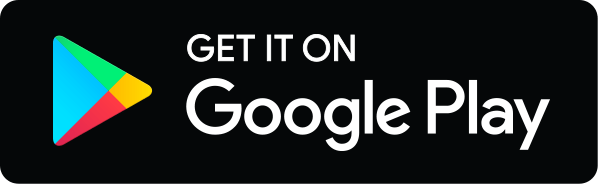
