As described in Chapter 15 , two fundamentally different approaches to positive pressure ventilation are possible. In pressure-controlled (PC) ventilation, the primary control variable governing gas delivery to the lungs is inflation pressure, and the tidal volume delivered to the lungs is the dependent variable that changes as the baby breathes and lung compliance and resistance change. In volume-controlled (VC) ventilation, tidal volume delivery is directly controlled and pressure becomes the dependent variable, changing as necessary to compensate for the baby breathing and to overcome resistive and elastic forces of the lungs ( Fig. 20-1 ).

PC, time-cycled, continuous-flow ventilation has been the standard of care in neonatal ventilation for more than 30 years because early attempts at VC ventilation in small preterm neonates were unsuccessful with the devices available at the time. The perceived advantages of PC ventilation are the ability to directly control the inflation pressure and time and to ventilate despite large leaks around the uncuffed endotracheal tubes used with neonates. A preoccupation with high inflation pressure as the chief culprit in ventilator-induced lung injury and air leak has led to a deeply ingrained “barophobia” that has persisted despite mounting evidence that pressure by itself, without generating excessively large tidal volume, is not the main cause of lung injury.
Rationale for Tidal Volume-Targeted Ventilation
Preclinical studies clearly demonstrate that tidal volume, rather than inflation pressure, is the critical determinant of ventilator-induced lung injury. Dreyfuss and colleagues demonstrated as early as 1988 that severe acute lung injury occurred in animals ventilated with large tidal volume, regardless of whether that volume was generated by a high or low inflation pressure ( Fig. 20-2 ). On the other hand, animals whose chest wall and diaphragmatic excursion were limited by external binding experienced much less lung damage despite being exposed to the same high inflation pressure. This work and other similar experiments clearly show that excessive tidal volume, not pressure per se, is chiefly responsible for lung injury. Pressure, without correspondingly high volume, is not by itself injurious to the lungs, although it could be injurious to immature airways.

An equally compelling reason for tidal volume-targeted ventilation (VTV) is the extensive body of evidence documenting that both hypercarbia and hypocarbia are associated with neonatal brain injury. Despite increasing awareness of its adverse consequences, inadvertent hyperventilation remains a common problem with pressure-limited ventilation, especially early in the clinical course when the baby starts breathing, lung compliance changes rapidly in response to clearing of lung fluid, surfactant is administered, and lung volume is optimized. Luyt et al. demonstrated that 30% of ventilated infants had at least one blood gas with PaCO 2 < 25 torr during the first day of life.
While there are important differences in how volume targeting is achieved with various ventilators, the primary benefit of VTV probably rests in the ability to regulate and maintain an appropriate tidal volume (V T ), regardless of how that goal is achieved. When V T is the primary control variable, inflation pressure will fall as lung compliance and patient inspiratory effort improve, resulting in real-time weaning of pressure, in contrast to intermittent manual lowering of pressure in response to blood gases. Real-time lowering of pressure avoids excessive V T and achieves a shorter duration of mechanical ventilation. The inflation pressure will also rise if for some reason the set V T is not delivered. Two meta-analyses that included a combination of several different modalities of VC and targeted ventilation documented a number of advantages of VC/VTV, compared to pressure-limited ventilation, including significant decrease in the combined outcome of death or bronchopulmonary dysplasia (BPD), lower rate of pneumothorax, less hypocarbia, decreased risk of severe intraventricular hemorrhage/periventricular leukomalacia, and significantly shorter duration of mechanical ventilation ( Table 20-1 ). These results are very encouraging, but some limitations should be recognized. Included studies were quite small, used a variety of modalities, and many of the key outcomes reported in the meta-analysis were not prospectively collected or defined. In some of the studies, other variables beyond volume versus pressure targeting also differed. The studies focused on short-term physiologic outcomes, rather than BPD as a primary outcome. Except for one follow-up study based on parental questionnaire, no long-term pulmonary or developmental outcomes have been reported as of this writing.
Outcome | No. of Studies | No. of Subjects | RR (95% CI) or Mean Diff (95% CI) |
---|---|---|---|
Mortality | 11 | 759 | 0.73 (0.51-1.05) |
BPD at 36 weeks ∗ | 9 | 596 | 0.61 (0.46-0.82) |
Any IVH ∗ | 11 | 759 | 0.65 (0.42-0.99) |
Cystic PVL ∗ | 7 | 531 | 0.33 (0.15-0.72) |
Grade 3-4 IVH ∗ | 11 | 707 | 0.55 (0.39-0.79) |
Pneumothorax ∗ | 8 | 595 | 0.46 (0.25-0.86) |
Any hypocarbia ∗ | 2 | 58 | 0.56 (0.33-0.96) |
Failure of assigned mode ∗ | 4 | 405 | 0.64 (0.43-0.94) |
Duration of supplemental oxygen (days) ∗ | 2 | 133 | −1.68 (−2.5 to −0.88) |
∗ Indicates a statistically significant benefit of volume-targeted ventilation.
Volume-Controlled versus Volume-Targeted Ventilation
VC, also known as volume-cycled, ventilators deliver a constant, preset V T into the ventilator circuit with each inflation. In theory, these ventilators allow the operator to select V T and respiratory rate and therefore directly control minute ventilation. Pressure rises passively, in inverse proportion to lung compliance, as the V T is delivered, reaching its peak just before the ventilator cycles off, allowing little time for intrapulmonary gas distribution. The ventilator delivers the set V T into the circuit, generating whatever pressure is necessary to overcome lung compliance and airway resistance, up to a set safety pop-off, typically set at a pressure >40 cm H 2 O. A maximum inflation time is also set as an additional safety measure. The ventilator cycles into expiration when the preset V T has been delivered or when the maximum inflation time has elapsed. The latter ensures that with very poor lung compliance, the ventilator does not generate a very prolonged inflation in an attempt to deliver a set V T that cannot be reached at the pressure pop-off value.
The major limitation of any VC ventilator is that what is controlled is the volume injected into the ventilator circuit and NOT the V T that enters the patient’s lungs . This limitation is based on the fact that the V T is measured at the ventilator end of the circuit and does not account for compression of gas in the circuit and humidifier or distention of the compliant circuit. In large patients with cuffed endotracheal tubes (ETTs), this loss is negligible and easily compensated for. But such is not the case in small preterm infants, whose lungs are only a fraction of the total volume of the circuit ( Fig. 20-3 ). Most modern ventilators have provisions to compensate for circuit compliance/gas compression, but this ability breaks down with the ubiquitous and highly variable leak around uncuffed ETTs used in newborn infants. These limitations can be overcome to a degree by using a separate flow sensor at the airway opening to monitor exhaled V T . This will allow the user to manually adjust the set V T (also known as V del ) to achieve the desired exhaled V T . Unfortunately, the ETT leak is usually variable, and thus frequent monitoring and adjustment may be necessary. An alternate approach to VC is to rely on clinical assessment of adequacy of chest rise and breath sounds to set the V del , which typically needs to be set at 10 to 12 mL/kg, to achieve effective V T of 4 to 5 mL/kg, and to make subsequent adjustments based on blood gas measurement. Despite these limitations, VC has been shown to be feasible even in small preterm infants when a flow sensor at the airway opening is used.

Neonatal Tidal Volume-Targeted Ventilation
In contrast to traditional VC ventilation, V T -targeted ventilation modalities are modifications of pressure-controlled ventilation designed to deliver a target V T by microprocessor-directed adjustments of inflation pressure or inflation time. Some devices regulate V T delivery based on flow measurement during inflation and others during exhalation. Each approach has advantages and disadvantages: leak is greater during inflation and thus exhaled V T more closely approximates true V T . Use of exhaled V T results in regulation of the peak pressure based on the previous ventilator cycle, whereas using inflation volume makes same-cycle control possible, but it is then not possible to compensate for ETT leak in real time. If the inflation volume were 10 mL and the ETT leak 50%, then the baby would be getting a V T of only 5 mL. When a large ETT leak is present, exhaled V T may underestimate true volume and inspiratory measurement will overestimate the true V T that enters the lungs. On balance, the use of exhaled V T appears to offer the best balance of safety and effectiveness. Newer modalities of VTV have increasingly come to closely resemble volume guarantee ventilation, which focuses on expired V T , as described below.
Volume Guarantee
Volume guarantee (VG) is an option available on the Dräger Babylog 8000+, the VN 500 (Dräger Medical, Lübeck, Germany), and the Leoni Plus (Heinen + Löwenstein GmbH, Bad Ems, Germany—not available in the United States). More recently, a version of VG has been implemented on the Avea ventilator (CareFusion, San Diego, CA) and the GE Engström Carestation (GE Healthcare, Chicago, IL). VG may be combined with any of the basic ventilator modes (continuous mandatory ventilation, assist/control [AC], synchronized intermittent mandatory ventilation [SIMV], pressure support ventilation). It is a volume-targeted, time- or flow-cycled, pressure-controlled form of ventilation. The operator chooses a target V T and a pressure limit up to which the ventilator operating pressure (working pressure) may be adjusted. The microprocessor compares the exhaled V T of the previous inflation and adjusts the working pressure up or down to target the set V T ( Fig. 20-4 ). The algorithm limits the pressure increment from one inflation to the next to a percentage of the amount needed to reach the target V T to avoid excessive oscillations, up to a maximum increase of 3 cm H 2 O. Consequently, with rapid, large changes in compliance or patient inspiratory effort, several cycles are needed to reach target V T . If the ventilator is unable to reach the target V T with the set inflation pressure limit, a “low V T ” alarm will sound, alerting the operator that an assessment is needed. The VG modality, as implemented on the Dräger Babylog 8000+ and VN 500 ventilators, which are designed specifically for newborn infants, employs separate controls for triggered and untriggered inflations. This is an important feature when ventilating spontaneously breathing preterm infants whose respiratory effort is highly variable, because as with all forms of synchronized ventilation, the V T is determined by a combination of the positive pressure from the ventilator and the negative intrapleural pressure resulting from the spontaneous effort of the infant ( Fig. 20-5 ). Consequently VG leads to a more stable V T than would be seen in similar modalities that use a single control algorithm ( Fig. 20-6 ). The impact of VG with the dual control algorithm compared to simple PC ventilation is seen in Figure 20-7 . A secondary safety feature designed to prevent delivery of excessively large inflations terminates an inflation on the same cycle if the V T target is exceeded by >30% based on inspiratory volume measurement (corrected for leakage). In an awake, actively breathing infant the variable patient contribution to transpulmonary pressure is always perturbing the equilibrium, causing the V T to fluctuate around the target V T . Thus the term volume guarantee is arguably a misnomer. However, there is good evidence that a completely constant V T leads to atelectasis over time; thus a physiologic variability of V T is actually desirable.




The VG modality, as implemented on a Dräger ventilator, has been studied more thoroughly than other modes of VTV. VG reduces the incidence of hypocarbia and the number of excessively large V T s. Specific clinical guidelines for VG have been published and are also provided below and in Table 20-2 . VG has been shown to be more effective when used with AC than with SIMV, probably because all inflations are subject to volume targeting.
Recommendation | Rationale |
---|---|
Initiation of VTV | |
|
|
Subsequent Adjustment | |
|
|
Weaning | |
|
|
Extubation | |
|
|
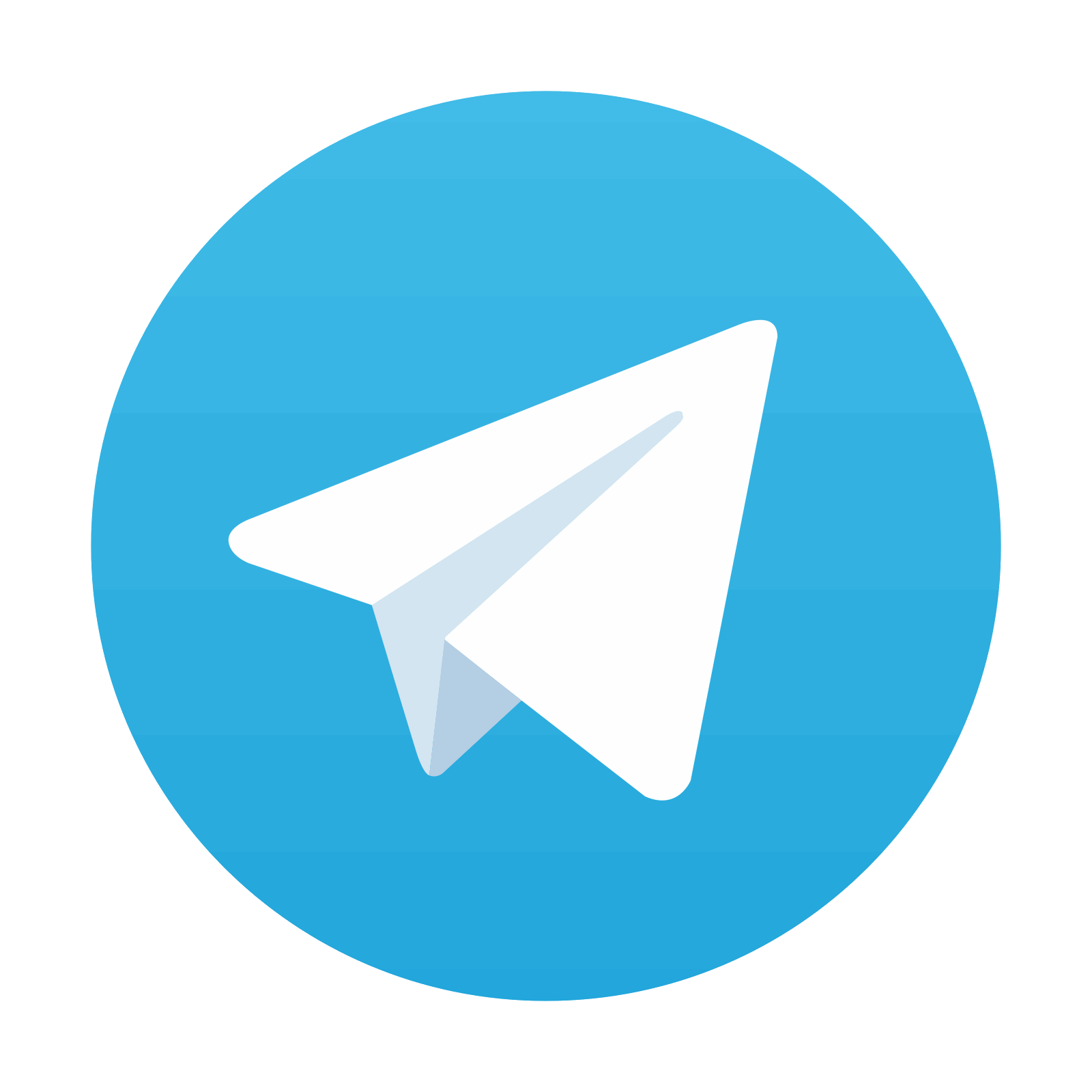
Stay updated, free articles. Join our Telegram channel

Full access? Get Clinical Tree
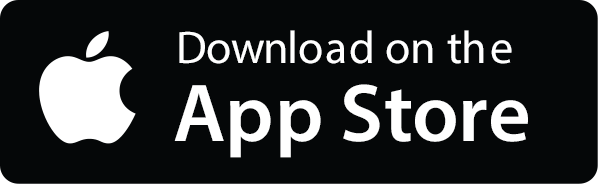
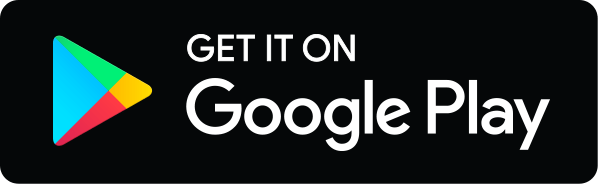
