Therapy for Perinatal and Neonatal Infections
David W. Kimberlin, Debra L. Palazzi, and Richard J. Whitley
GENERAL CONSIDERATIONS
Limitations in the immunologic response predispose the infant to certain viral and bacterial infections acquired during the prenatal, perinatal and immediate postpartum period. The development of the immune system, and the mechanisms of response to both bacterial infection are discussed in Chapter 186. Common perinatal viral and bacterial infections are discussed in this chapter.
NEONATAL VIRAL PATHOGENS
Viral pathogens can infect the fetus or neonate in utero via transplacental or ascending transmission of microorganisms, the peripartum period at delivery by passage through an infected birth canal, or the postpartum period by acquisition within the first several weeks of life. Human cytomegalovirus (HCMV) and rubella virus can cause significant sequelae when acquired in utero, whereas herpes simplex virus types 1 and 2 (HSV-1, HSV-2) and enteroviruses can cause life-threatening disease when acquired in the peripartum and postpartum periods.
The usual mode of transmission, clinical presentations, diagnosis and treatment of common neonatal viral infections are discussed in the following section and summarized in Table 230-1.
HERPES SIMPLEX VIRUS
Herpes simplex virus (HSV) infections can be acquired in utero (5%), peripartum (85%), or postpartum (10%). Peripartum or postpartum disease can be classified as disseminated disease involving multiple visceral organs, including lung, liver, adrenal glands, skin, eye, and/or the brain (disseminated disease); central nervous system disease, with or without skin lesions (CNS disease); and disease limited to the skin, eyes, and/or mouth (SEM disease). This classification system is predictive of both morbidity and mortality.1-5 Patients with disseminated or SEM disease generally present to medical attention at 10 to 12 days of life, and patients with central nervous system disease on average present somewhat later at 16 to 19 days of life.2
Historically, disseminated HSV infections have accounted for approximately one half to two thirds of all children with neonatal HSV disease. However, this figure has been reduced to about 25% since the development and utilization of antiviral therapy, likely the consequence of recognizing and treating SEM infection before its progression to more severe disseminated disease.6 Central nervous system involvement is a common component of this category of infection, occurring in 60% to 75% of infants with disseminated disease.7 Although the presence of a vesicular rash can greatly facilitate the diagnosis of HSV infection, over 20% of neonates with disseminated HSV disease will not develop cutaneous vesicles during the course of their illness.2,6,8,9Patients commonly present with viral sepsis, including respiratory collapse, liver failure, and disseminated intravascular coagulopathy (DIC). Hepatitis and pneumonitis are common in disseminated neonatal HSV disease. Death from disseminated neonatal HSV infection is usually the result of the severe coagulopathy, liver dysfunction, and pulmonary involvement of the disease.
Table 230-1. Neonatal Viral Pathogens and Treatment
Almost one third of all neonates with HSV infection are categorized as having central nervous system (CNS) disease (with or without SEM involvement).6 Clinical manifestations of CNS disease include seizures (both focal and generalized), lethargy, irritability, tremors, poor feeding, temperature instability, and bulging fontanelle. Between 60% and 70% of babies classified as having CNS disease have associated skin vesicles at any point in the disease course.2,8 In the absence of skin lesions and frank CNS signs, the initial presentation can be indistinguishable from other viral and bacterial infections that lead clinicians to suspect possible sepsis in neonates. With CNS neonatal HSV disease, mortality is usually the product of devastating brain destruction, with resulting acute neurologic and autonomic dysfunction. Neonatal HSV can involve any and often multiple parts of the brain, in contrast with the typical temporal lobe predilection seen with herpes simplex encephalitis with onset beyond the neonatal period.
Infection localized to the skin, eye, and/or mouth (SEM disease) historically accounted for approximately 20% of all cases of neonatal HSV disease. With the introduction of early antiviral therapy, this frequency has increased to approximately 45%.6 By definition, babies with SEM disease have limited infection, and 80% to 85% have associated vesicular lesions apparent on physical examination.2
DIFFERENTIAL DIAGNOSIS
A number of other conditions, both infectious and noninfectious, can mimic neonatal HSV infection. These include hyaline membrane disease, intraventricular hemorrhage, necrotizing enterocolitis, and various ocular or cutaneous disorders. Bacterial pathogens of newborns with systemic and/or cutaneous manifestations that can be confused with neonatal HSV disease include group B Streptococcus, Staphylococcus aureus, Listeria monocytogenes, and gram-negative bacteria. Exanthemous viral agents that can be confused for neonatal HSV infection include varicella-zoster virus infection, enteroviral disease, and disseminated cytomegalovirus infection. Other infectious pathogens on the differential diagnosis list include toxoplasmosis, rubella, and syphilis. Noninfectious cutaneous disorders should also be considered, including erythema toxicum, neonatal melanosis, acrodermititis, and incontinentia pigmenti. (See Chapter 309.)
OUTCOMES
In the pre-antiviral era, 85% of patients with disseminated neonatal HSV disease died by 1 year of age, as did 50% of patients with central nervous system (CNS) neonatal HSV disease.5 With current antiviral therapy, 12-month mortality has been reduced to 29% for disseminated neonatal HSV disease and to 4% for CNS HSV disease.1 Lethargy and severe hepatitis are associated with mortality among patients with disseminated disease, and prematurity and seizures are associated with increased mortality in patients with CNS disease.2
Improvements in morbidity rates with antiviral therapies have not been as dramatic as with mortality. The proportion of survivors of disseminated neonatal HSV disease who have normal neurologic development has increased from 50% in the pre-antiviral era5 to 83% today.1 In the case of CNS neonatal HSV disease, there has been no change at all, with 33% of patients in the pre-antiviral era and 31% of patients today having normal neurologic development.1,5 Seizures at or before the time of initiation of antiviral therapy are associated with increased risk of morbidity both in patients with CNS disease and in patients with disseminated infection.2
In contrast to disseminated or CNS neonatal HSV disease, morbidity following SEM disease has dramatically improved during the antiviral era. Prior to the development of vidarabine or acyclovir, 38% of SEM patients experienced developmental difficulties at 12 months of age.5 Today, fewer than 2% of acyclovir recipients have developmental delays following recovery from SEM disease.1,3
PREVENTION
A number of approaches are employed by obstetricians to try to prevent neonatal herpes simplex virus (HSV) disease. Cesarean delivery in a woman with active genital lesions can reduce the infant’s risk of acquiring HSV111,112 and is recommended when genital HSV lesions or prodromal symptoms are present at the time of delivery.113 Physicians caring for neonates delivered by cesarean section should be aware that neonatal HSV infections have occurred in spite of cesarean delivery performed prior to the rupture of membranes.6,114
An increasingly common practice among obstetricians is the use of oral antiviral suppressive therapy near the end of pregnancy to prevent genital HSV recurrences at delivery. Several small studies suggest that suppressive acyclovir or valaciclovir therapy during the last weeks of pregnancy decreases the occurrence of clinically apparent genital HSV disease at the time of delivery,115-118 with an associated decrease in cesarean section rates for the indication of genital HSV.115,116,118 However, because viral shedding still occurs (albeit with reduced frequency),117-120 the potential for neonatal infection likely is not completely avoided. Cases of neonatal HSV disease have occurred among babies born to women who were on suppressive antiviral therapy at the end of pregnancy.121 Of the small number of studies evaluating suppressive antiviral therapy near the end of pregnancy, only 2 have investigated the babies for evidence of toxicity.118,120 Even though these studies did not identify any neonatal side effects from maternal suppression, only 123 babies whose mothers received valaciclovir were evaluated.118,120 Given how widespread the use of antiviral suppression has become in pregnant women113 and the suggestion of toxicity in infants receiving suppressive therapy following neonatal herpes simplex virus (HSV),122 additional studies are urgently needed to define the safety (and arguably the efficacy) of this approach.123 Pending these data, pediatricians should not assume that the risk of neonatal HSV is completely eliminated by a woman receiving suppressive oral antiviral therapy at the time of delivery.
Currently an HSV glycoprotein vaccine is under investigation. Even if efficacious for genital HSV infection, its impact on neonatal herpes will remain to be established.
See Chapter 309 for further discussion of herpes simplex virus.
ENTEROVIRUSES
CLINICAL FEATURES AND DIFFERENTIAL DIAGNOSIS
Enteroviral infection is a serious and important health problem in the newborn infant.10 Prospective studies have documented that nonpolio enteroviruses are the most commonly identified cause of fever without an apparent focus among infants under 1 month of age who present for emergent care.11-13 During the summer and fall months, enteroviruses account for at least 53% to 63% of these cases.13 Fever is often the sole examination finding among babies with postnatally acquired enteroviral disease, although some infants will present with other nonspecific findings such as irritability, lethargy, poor feeding, vomiting, diarrhea, exanthems, and signs of upper respiratory tract infection.14 Approximately half of all enterovirus-infected infants younger than 3 months of age who undergo lumbar puncture for fever are diagnosed with meningitis,12,15 although there are no clinical features that distinguish those with meningitis from those without meningitis.15
Patients with neonatal enteroviral sepsis syndrome usually present in the first 2 weeks of life with any number of symptoms including fever, irritability, anorexia, lethargy, rash, emesis, diarrhea, or abdominal distention.16,17 Other symptoms and signs relate to specific organ infections and include jaundice, hepatomegaly, seizures, apnea, and bulging anterior fontanel. Common laboratory abnormalities include cerebrospinal fluid (CSF) pleocytosis, chest radiographic infiltrates, thrombocytopenia, hyperbilirubinemia, leukocytosis, and transaminase elevations.17 Severe hepatitis can result from neonatal echovirus infection or may occur along with myocarditis and other manifestations of neonatal coxsackievirus B disease. Although severe hepatitis is usually the consequence of echovirus 11 infection,10 neonatal disease caused by echovirus serotypes 6, 7, 9, 14, 17, 19, and 21 can also demonstrate dramatic hepatic involvement.18-23 Extensive hepatic necrosis and overwhelming hepatic failure can result. Severe hepatitis syndrome has been defined as a serum transaminase level of greater than three times the upper limit of normal; a prothrombin time or partial thromboplastin time greater than twice the control value; or evidence of hepatic necrosis or extensive hemorrhage at postmortem examination.10 Postmortem examination of neonates who die of echovirus disease usually reveals inflammatory changes limited to the liver.19,24,25 Survivors may have residual hepatic dysfunction.17 Neonatal myocarditis is most frequently caused by group B coxsackievirus serotypes 2 through 5. Such infants frequently demonstrate concomitant meningoencephalitis, pneumonia, hepatitis, pancreatitis, or adrenalitis.
Enteroviral infections in neonates are especially prevalent during the summer and early fall months.13,26 Commonly observed enteroviral isolates include several serotypes of the echovirus genus as well as coxsackievirus A and B; unclassified enteroviruses are infrequently isolated.13 Echoviruses are isolated most frequently in cases of neonatal enteroviral sepsis syndrome, with coxsackievirus group B occurring less often.26 The most common sites of virus isolation are blood, urine, throat, feces, and cerebrospinal fluid.13,16
Approximately 60% to 70% of infants diagnosed with enteroviral disease within the first 10 days of life acquire their infection by transmission from the mother at the time of delivery.10,27 Infected mothers usually report a febrile gastrointestinal or respiratory illness in the 2-week period preceding delivery of the infant. Congenital infection is rare but often fatal.28,29 Importantly, perinatal transmission of enteroviral infections in newborn nurseries has also been implicated as an important route of spread of the disease in newborn infants.10,30-32 Postnatal transmission of enteroviral infections during seasonal peaks of enterovirus activity occurs commonly.33 During periods of high prevalence of enterovirus infection in the community, there are many potential sources of infection both during and after discharge from the nursery, including the mother, other family members, and hospital staff.34
DIAGNOSTIC EVALUATION
The diagnosis of enterovirus infection has traditionally been based on isolating virus in cell culture from specimens obtained during the acute illness. The echoviruses and group B coxsackieviruses are isolated readily in the cell lines used by most clinical virology laboratories. A combination of human fibroblasts, primary monkey kidney cells, and human rhabdomyosarcoma (RD) cells are optimal for cell culture isolation of a broad range of enteroviruses.34,89
Echoviruses and group B coxsackieviruses can be recovered from infants with severe myocarditis or hepatitis from multiple sites, including respiratory secretions, feces, urine, CSF, and blood. Despite being the “gold standard,” the utility of diagnosis of enteroviral infections by viral culture is limited by a sensitivity of only 65% to 75%.90 Polymerase chain reaction detection of enteroviral RNA has been shown to be an effective alternative to viral culture in the diagnosis of enteroviral meningitis using CSF specimens91,92 and in the diagnosis of neonatal enteroviral infections with serum and urine specimens.93 Sensitivity and specificity, respectively, of PCR for the detection of enteroviral RNA have been reported as 100% and 97% (CSF); 92% and 98% (serum or plasma); 95% and 88% (throat); and 77% and 95% (urine).90
TREATMENT
Management of children with neonatal enteroviral sepsis syndrome is directed toward the symptom complex. Cultures of body fluids for bacterial and viral pathogens are performed and broad spectrum antibiotic therapy initiated until the results of bacterial cultures are made available. Acyclovir is frequently administered as well until HSV disease is excluded. Supportive care has been the rule in the management of these patients. Intravenous gammaglobulin has been utilized in case reports and small clinical trials,99,100 but definitive proof of efficacy has not been established. No enteroviral-specific antiviral medication is currently available for the management of these gravely ill neonates, although the experimental drug pleconaril is undergoing evaluation by the National Institute of Allergy and Infectious Diseases (NIAID) Collaborative Antiviral Study Group (CASG) in babies with neonatal enteroviral sepsis syndrome. Because there is no targeted antiviral medication for the treatment of enteroviral disease, complications of therapy relate to toxicities of intravenous gammaglobulin and adverse consequences of the supportive care of these gravely ill babies.
OUTCOMES AND PREVENTION
Approximately 75% of cases of neonatal enteroviral disease carry a benign outcome, with diagnosis and symptomatic treatment in nonintensive care unit settings.26 For the remainder of patients, more serious consequences can result from systemic enteroviral infection, including meningoencephalitis, cardiovascular collapse, myocarditis, or hepatitis.26 These last two organ-specific complications carry high mortality rates. More than 80% of babies with enteroviral sepsis syndrome involving the liver will die within 1 to 3 weeks of onset of symptoms, and mortality among infants with myocarditis alone is generally reported to be 30% to 50%.34
Given the ubiquity of enteroviruses in the environment during the summer and fall, specific measures designed to diminish transmission of virus to neonates are lacking. Careful hand-washing likely can have an impact on viral transmission to young babies and remains the best preventative method.
For further discussion of enterovirus see Chapter 306.
CYTOMEGALOVIRUS
CLINICAL FEATURES AND DIFFERENTIAL DIAGNOSIS
Cytomegalovirus (CMV) is a ubiquitous virus, and humans are its only reservoir. In populations with higher socioeconomic conditions, approximately 40% of adolescents are seropositive, with another 1% seroconverting annually thereafter.35 Approximately 70% of adults from higher socioeconomic strata and 90% from lower socioeconomic strata eventually become infected with CMV.
Transmission of CMV occurs by direct or indirect person-to-person contact. Sources of virus include urine, oropharyngeal secretions, cervical and vaginal secretions, semen, milk, tears, blood, and transplanted organs.36,37 The spread of infection requires close or intimate contact with infected secretions, including sexual contact.36,38,39 Other modes of transmission include intrauterine transmission,40 perinatal acquisition from breastfeeding,37 and spread among children in group childcare facilities.41 For the latter group, transmission is the result of horizontal transmission from child to child through saliva on hands and toys.42-44 This mode of viral transmission is so efficient that it is not unusual to find excretion rates as high as 20% to 40% in young toddlers attending daycare. Young children acquiring primary CMV infections at day-care facilities are at risk of transmitting to their seronegative parents, which in turn can lead to intrauterine transmission should a mother be pregnant at the time.45-47 Seronegative caretakers working in daycare centers are also at increased risk of acquisition of CMV infection.48
Intrauterine infection presumably follows maternal viremia and associated placental infection. CMV is the most common congenital infection in humans, with approximately 1% of all live births in the United States being infected with CMV (∼ 40,000 babies per year).49 CMV can be acquired in utero during any trimester of pregnancy. Congenital CMV infection is the most frequent known viral cause of mental retardation50 and is the leading nongenetic cause of neurosensory hearing loss in many countries including the United States.51-53 Of those fetuses infected, approximately 10% will be symptomatic at birth, and ∼20% of these patients will die in the neonatal period; of the survivors, 90% will have significant neurologic sequelae,40,54-58 including hearing deficits in 30% to 65% of patients.55,59
Children born with congenital CMV infection but without overt symptoms may nevertheless develop sequelae on follow-up, particularly sensorineural hearing loss. A recent systematic review of all prospective studies published worldwide reported that 13.5% of babies with congenital CMV infection develop sensorineural hearing loss on follow-up.60
DIAGNOSTIC EVALUATION
Detection of cytomegalovirus (CMV) by culture from urine or saliva within the first 2 to 4 weeks of life is diagnostic for congenital infection. Human fibroblasts are routinely used to support CMV replication in vitro. Cultures are observed at least twice weekly for the typical focal cytopathic effect (CPE) of CMV. Occasionally, urine samples from cases of congenital infection produce widespread CPE within 24 to 48 hours which resembles that of HSV. More usually, the CPE evolves only slowly, typically becoming apparent at 14 to 16 days so that cultures must be maintained for a minimum of 21 days before being reported as negative. To facilitate more rapid finalization of culture results, the shell vial assay utilizes monoclonal antibody detection of CMV antigens early in the culture process to confirm infection in a specimen. Given the large quantity of virus present in the urine or saliva of congenitally infected babies, either culture or shell vial assay should be used to confirm congenital CMV infection. More recently, as with the diagnosis of HSV, the application of PCR is supplanting tissue culture confirmation of CMV.
TREATMENT
Six weeks of intravenous ganciclovir therapy (6 mg/kg/dose administered intravenously every 12 hours) reduces the progression of hearing loss and may favorably impact the neurodevelopmental outcomes of babies with symptomatic congenital cytomegalovirus (CMV) disease involving the CNS.101 Specifically, 21% of symptomatic babies with CNS involvement who begin ganciclovir treatment within the first month of life have hearing deterioration at approximately 2 years of age, compared with 68% of untreated babies.101 Ganciclovir-treated babies with symptomatic congenital CMV disease involving the CNS also have fewer developmental delays at 6 and 12 months of age, as measured by the Denver developmental assessment tool.102 The NIAID CASG is now initiating a randomized, controlled trial comparing 6 weeks versus 6 months of valganciclovir oral solution to systematically assess efficacy and safety outcomes of longer-term antiviral treatment of symptomatic congenital CMV disease, utilizing the research grade of oral valganciclovir (www.casg.uab.edu/).
Two thirds of babies receiving intravenous ganciclovir for the treatment of symptomatic congenital CMV disease involving the CNS develop significant neutropenia.101 When it occurs, the neutropenia usually develops during the first 2 weeks of treatment. It usually can be managed with serial observations or dose modifications. Recent investigation of a research-grade formulation of oral valganciclovir in babies with symptomatic congenital CMV disease suggests that this formulation may result in fewer instances of neutropenia,103 although this must be verified in larger trials.
In some animal models, ganciclovir (and therefore valganciclovir) is carcinogenic and gonadotoxic. Such serious toxicities have not been seen to date in humans. There are no data on antiviral treatment of babies with asymptomatic congenital CMV infection. Given the toxicities of ganciclovir, antiviral therapy in this larger population cannot be recommended at this time.
OUTCOME AND PREVENTION
Approximately 20% of babies with symptomatic congenital CMV disease will die in the neonatal period. Of the survivors, 90% will have significant neurologic sequelae,40,54-58 including hearing deficits in 30% to 65% of patients.55,59 In at least 30% of untreated babies, their CMV-induced hearing loss will not be static but will progress over time.53,55,59,106,107 Even with ganciclovir treatment, progression of hearing loss can occur, albeit at a lower frequency.101 Thus, the majority of symptomatically infected babies will have sensorineural hearing loss, mental retardation, microcephaly, seizures, and/or paresis/paralysis. These impairments frequently result in spastic quad-riplegia requiring lifelong dependence on a wheelchair, along with cognitive and speech impairments that dramatically limit their ability to interact with and function in the world. Patients with this degree of neurologic impairment generally have a life expectancy of less than 10 to 15 years.
In 1999, the Institute of Medicine assessed the need for a cytomegalovirus (CMV) vaccine as the highest of all priorities.124 A glycoprotein B CMV vaccine demonstrated promise in a Phase II trial in women of childbearing age125 and is currently being investigated in adolescent girls. CMV hyperimmune globulin was shown in one study to protect against in utero transmission of CMV to the fetus.126 However, methodologic difficulties with the selection of subjects in this trial make it difficult to know the extent, if any, to which such an intervention is beneficial.
RUBELLA
Rubella is a highly contagious disease, and the incidence of rubella infection during an epidemic cycle approaches 100% of susceptible hosts in closed populations (ie, military recruits).61-63 Virtually all susceptible household contacts are infected during such outbreaks.64 At least half of all serologically confirmed childhood primary rubella infections result in clinically in apparent illness.62,65 Reinfection with rubella virus can occur following natural infection or vaccination but is usually asymptomatic.63,66,67 Up to 80% of persons previously vaccinated against rubella will be reinfected during an epidemic.63,66 Rubella reinfection during pregnancy can result in congenital rubella syndrome, although this is a rare event.68,69
Fetal infection can occur throughout pregnancy, with the risk of infection being greatest during the first trimester, decreasing during the second trimester, and then rising again as the fetus approaches term. The risk of congenital anomalies in live-borne children following fetal infection also varies according to the month of pregnancy in which maternal infection occurs, with over 85% of infants born to women infected with rubella virus during the first 8 weeks of pregnancy having anomalies detected during the first 4 years of life.70 This risk declines to 50% of infants born to mothers infected at 9 to 12 weeks gestation, to 15% of infants born to women infected at 13 to 20 weeks gestation, and to close to 0% in infants born to mothers infected beyond 20 weeks gestation.70-72
The clinical manifestations of congenitally acquired rubella are usually severe. The classic triad of congenital rubella consists of cataracts, cardiac abnormalities, and deafness. The consequences of in utero rubella infection can be considered broadly as belonging to one of three categories: signs and symptoms that are transiently apparent in affected infants, permanent manifestations that are noted within the first year of life, and manifestations of congenital rubella that are delayed in onset until later in life (2 years of age to adulthood).73 Transient findings include dermal erythropoiesis (“blueberry muffin” rash), chronic rash, thrombocytopenic purpura, hemolytic anemia, generalized lymphadenopathy, interstitial pneumonitis, hepatitis, hepatosplenomegaly, nephritis, myositis, myocarditis, bone radiolucencies, and meningoencephalitis.74 Among the more common of these findings are rash (petechial or blueberry muffin rash), hepatosplenomegaly, jaundice, pulmonary involvement, meningoencephalitis, and radiographic abnormalities.74 The majority of such infants are intrauterine growth restricted at delivery.73 Sensorineural hearing loss is the most common permanent manifestation of congenital rubella, with cardiovascular anomalies, ophthalmologic findings, and neurologic impairment also occurring commonly.
DIAGNOSTIC EVALUATION
Rubella infection is definitively diagnosed by isolation of rubella virus in tissue culture, using one of several cell lines and primary cell strains. Viral interference in African green monkey kidney cells is one common culture technique by which the presence of rubella virus is demonstrated. Infection in African green monkey kidney tissue culture by rubella virus is suggested by the failure of the typical enteroviral CPE to occur after challenge with echovirus 11 or other enteroviruses. The presence of rubella virus is then confirmed by an additional technique, such as neutralization or fluorescence with specific antirubella serum. Virus can be readily isolated from throat swabs in postnatal rubella infection for 6 days before and after the onset of rash.65,66 Virus can be isolated from specimens from the nasopharynx, conjunctivae, urine, blood buffy coat, and cerebrospinal fluid of patients with congenital rubella. In utero infection with rubella virus can be demonstrated by nucleic acid hybridization or by virus-specific antigen detection in specimens from the chorionic villus or fetus.94,95 Polymerase chain reaction assays have also been developed.96
Despite the definitive results afforded by direct viral isolation in tissue culture, the majority of rubella cases are diagnosed serologically. A 4-fold rise in rubella-specific IgG between acute and convalescent serum specimens confirms the diagnosis of postnatal rubella. Commercially available rubella virus IgG avidity assays are of variable sensitivity.97 Demonstration of the presence of rubella-specific IgM is also diagnostic for recent infection with rubella virus. Rarely, rubella-specific IgM can be detected with reinfection. Serologic diagnosis of congenital rubella can be demonstrated by the presence of rubella-specific IgM in neonatal serum. Confirmation of the diagnosis based solely upon the presence of IgG is difficult. In such cases, it is necessary to test sequential sera from the infant for rubella-specific IgG. In most cases, the IgG titer will decrease over several months if it is solely of maternal origin, whereas it will rise if congenital infection has occurred and the infant is producing rubella-specific IgG.
TREATMENT AND OUTCOMES
Interferon and amantadine have been used in individual cases of congenital rubella syndrome.104,105 Patients with congenital rubella require supportive care not only in the neonatal period but throughout life for such permanent impairments as deafness and heart defects. Prompt identification of such afflictions is of the utmost importance so that they can be managed with adjunct interventions.
Permanent sequelae from infancy onward include sensorineural hearing loss in 80% of congenitally infected patients,73 as well as cardiovascular anomalies, ophthalmologic findings, and neurologic impairment. Structural defects of the cardiovascular system occur in the majority of infants whose mothers acquired rubella during the first 2 months of gestation.74 Patent ductus arteriosus (PDA) is the most common of these cardiovascular sequelae, followed by pulmonary artery stenosis and pulmonary valvular stenosis. Two thirds of patients with PDAs will have other cardiovascular lesions present.74 Ophthalmologic findings include cataracts (bilateral or unilateral), retinopathy, and microphthalmia. The retinopathy results from pigmentary defects in the retina and usually does not interfere with vision. In contrast, a small number of patients have congenital glaucoma that, if undetected, can result in visual impairment. Permanent neurologic impairment can result from the active replication in the central nervous system (CNS) of rubella virus both in utero and following delivery. Indeed, such neurologic sequelae as mental retardation and motor disabilities correlate with the severity and persistence of the acute meningoencephalitis that is present at delivery in 10% to 20% of infants with congenital rubella syndrome.74 Movement and behavioral disorders can also be seen in surviving patients.
Sequelae of congenital rubella that develop in childhood or adulthood but are not present in infancy include endocrinopathies, deafness, ocular damage, vascular effects, and progressive rubella panencephalitis.108,109 Of these, the development of insulin-dependent diabetes mellitus (IDDM) occurs most frequently, with approximately 20% of patients being diagnosed with IDDM by the time they reach adulthood.73 Autoimmune-mediated thyroid dysfunction can also be seen.110
For counseling purposes, determination of the risk of congenital defects after confirmed maternal infection can be calculated by multiplying the rates of fetal infections by the rates of defects in infected infants. As such, the risks are 90% for maternal infection before the 11th week of gestation; 33% for infection occurring during weeks 11 and 12; 11% for infection from weeks 13 to 14; and 24% for infection between weeks 15 and 16.73
PREVENTION
Rubella and congenital rubella syndrome prevention are among the hallmark successes of the vaccination programs of the United States. By 2005, endemic transmission of rubella had been eliminated in the United States,127 and cases of rubella and of congenital rubella syndrome in the United States in 2007 were 99.9% and 99.3% lower, respectively, compared to the prevaccine era.128 Since 2001, fewer than 25 rubella cases have been reported each year in the United States, and vaccination coverage is at least 95% among school-aged children.127 An estimated 91% of the US population is immune to rubella.127 The remarkable success in decreasing the incidence of rubella in this country and others has led scientists and international organizations to consider the goal of rubella eradication.129-133
Further information on rubella is provided in Chapter 320.
NEONATAL BACTERIAL INFECTIONS
Intrauterine bacterial infection can result from overt or subclinical maternal infection, and sequelae depend on the infectious agent and timing of the infection in gestation. Congenital bacterial infection can cause spontaneous abortion, stillbirth, premature birth, and symptomatic neonatal infection. Intrapartum infection commonly is caused by bacteria that colonize the maternal genitourinary and gastrointestinal tracts; postpartum infection can occur by direct maternal contact or through exposure to organisms and other persons in the environment.
NEONATAL SEPSIS
Neonatal sepsis is a clinical syndrome characterized by systemic signs of infection in the first month of life. Neonatal systemic bacterial infections occur in 1 to 5 per 1000 live births. Over the past 20 years, the mortality rate due to neonatal bacterial infections has declined from 30% to 40% to 2% to 15%, in part due to enhanced awareness of maternal and infant risk factors and to earlier treatment (eTable 230.1). Early-onset and late-onset sepsis are two patterns of illness that characterize systemic bacterial infections during the first month of life (Table 230-2).
Neonates with early-onset disease have symptoms before 7 days of life, but the majority present with fulminant, systemic illness within 24 hours of birth. Infants who develop early-onset disease usually have a history of one or more risk factors associated with the pregnancy and delivery such as premature or prolonged rupture of maternal membranes, preterm onset of labor, preterm delivery, low birth weight, chorioamnionitis, peripartum maternal fever, septic or traumatic delivery, lack of prenatal care, and maternal urinary tract infection. Bacteria responsible for early-onset disease are acquired from the maternal genital tract. The typical clinical presentation often is of nonspecific signs and respiratory distress. The mortality rate varies from 3% to as high as 50% (eg, gram-negative pathogens).
Late-onset disease is variably defined as occurring after 72 hours in hospitalized infants to after 6 days in neonates in the community. Patients with late-onset infections can have a history of obstetric complications, but these are less characteristic than in early-onset sepsis. The bacteria causing late-onset infection can be from the maternal genital tract, the hospital environment, or the community. The mortality rate usually is lower than that in early-onset sepsis but varies between 2% and 40%, especially for gram-negative pathogens in very low (<1500 g) birth weight (VLBW) infants. The clinical presentation for late-onset disease also varies but often is focal and may manifest as pneumonia, meningitis, soft tissue infection, or other focal illness. With increasing survival of very low birth weight and extremely low birth weight (ELBW) (<1000 g) infants over the last decades, a third category of neonatal sepsis, very late-onset disease (>89 days), has emerged. These infants, because of their ongoing hospitalization and corrected gestational age of less than 28 weeks, are considered to have an extended period of time during which they are classified as neonates.
Table 230-2. Pathogens Causing Neonatal Septicemia
The most common pathogens causing early-onset bacterial infections are group B streptococci (GBS) and Escherichia coli as well as other gram-negative bacilli (Table 230-2). These organisms account for two thirds of all cases of early-onset disease and remain common as causes of late-onset sepsis. Other pathogens that cause early-onset sepsis include streptococci such as Enterococcus, αhemolytic streptococci, and, rarely, Streptococcus pneumoniae, Haemophilus influenzae, and other flora of the maternal genital tract.
Late-onset infection can be caused by the organisms listed above, but gram-negative pathogens also include those acquired in the health care setting that are potentially multi-drug resistant, such as Serratia marcescens, Klebsiella species, Pseudomonas aeruginosa, Citrobacter species, and Enterobacter species.
CLINICAL FEATURES
Clinical signs of bacterial sepsis in the neonate may be subtle and do not distinguish among organisms in most cases. Fever or hypothermia can be the sole examination finding, although it is absent in approximately half of infants with sepsis However, sustained fever for more than 1 hour generally indicates infection. Many infants will present with nonspecific findings such as irritability, lethargy, poor feeding, abdominal distention, vomiting, diarrhea, or exanthems. One third to one half of neonates with bacterial infection will have signs of respiratory distress, such as apnea, cyanosis, or bradycardia. In infants from birth to 8 weeks of age, the most reliable clinical signs of sepsis can include changes in affect, peripheral perfusion, and respiratory status. Alterations in feeding pattern, level of alertness, level of activity, and muscle tone, although often present, may be less sensitive indicators. Jaundice (direct hyperbilirubinemia) can indicate infection, especially when it occurs within the first 24 hours of life without Rh or ABO blood group incompatibility and when it is accompanied by an elevated direct bilirubin concentration. Infants with bacterial meningitis can present with seizures, bulging fontanelle, or nuchal rigidity in addition to manifesting the same nonspecific signs as those with septicemia.
In VLBW infants, the nonspecific and subtle nature of the signs of neonatal sepsis makes recognizing infection even more problematic. The clinical signs of late-onset sepsis in these infants can include increasing apnea and bradycardia episodes (55%), increasing oxygen requirement (48%), feeding intolerance, abdominal distention or guaiac-positive stools (46%), lethargy and hypotonia (37%), and temperature instability (10%). Unexplained metabolic acidosis and hypoglycemia can be present and are laboratory indicators of the metabolic derangement accompanying sepsis. In infants with nonfocal and nonspecific signs of illness, the differential diagnosis includes noninfectious etiologies such as hyaline membrane disease, intraventricular hemorrhage, congenital heart disease, metabolic disorders, and birth asphyxia.
Infants with sepsis can present with focal infection of any organ. However, excluding pneumonia and meningitis, focal infection in neonates occurs more often with late- rather than early-onset disease. Evaluation of infants with suspected septicemia should include a careful search for primary or secondary foci such as meningitis, pneumonia, urinary tract infection, peritonitis, otitis media, conjunctivitis, septic arthritis, osteomyelitis, or soft tissue infection.
COMMON BACTERIAL PATHOGENS IN THE NEONATE
Group B Streptococcus
Streptococcus agalactiae, or Lancefield group B Streptococcus (GBS), has been the most frequent organism causing invasive disease in neonates for nearly the past 4 decades. GBS is classified immunochemically into serotypes on the basis of its capsular polysaccharides. Nine capsular types Ia, Ib, II, III, IV, V, VI, VII, and VIII have been characterized. Types Ia, Ib, II, III, and V account for nearly all cases of early onset disease, and type III strains predominate among late-onset infections, especially those accompanied by meningitis.
GBS frequently is found in the lower genital and gastrointestinal tracts of up to 30% of healthy adult women and men and in the upper respiratory tracts and lower gastrointestinal tracts of neonates. The organism also has been isolated from various body fluids and sites including blood, pleural, or peritoneal fluids; CSF exudates; stool; urine; cervix; vagina; throat; skin; joints; bones; and wounds.
Early-onset disease caused by GBS presents as a multiorgan system illness, frequently characterized by severe respiratory distress, with rapid onset usually during the first day or two of life. The mortality rate is estimated at 3% but was as high as 50% in the 1970s. Late-onset neonatal sepsis frequently is accompanied by meningitis, and usually is more insidious in clinical presentation than early-onset disease. Many of the infants are products of normal term pregnancies and deliveries. In addition to sepsis and meningitis, other manifestations of neonatal disease caused by GBS include pneumonia, empyema, facial cellulitis, ethmoiditis, orbital cellulitis, conjunctivitis, necrotizing fasciitis, osteomyelitis, suppurative arthritis, and impetigo. Bacteremia without systemic or focal signs of sepsis can occur.
Group A Streptococcus
Streptococcal puerperal sepsis and obstetric infections have been recognized for centuries as causes of morbidity and mortality among parturient women and their newborns. Group A Streptococcus (GAS) now is an infrequent cause of neonatal sepsis but can occur rarely in nursery outbreaks. Additional information is given in Chapter 285.
Enterococci
Enterococccus faecalis is the most common cause of group D streptococcal sepsis in neonates, with a smaller number of cases caused by Enterococcus faecium. In most cases, the clinical presentation of enterococcal sepsis in neonates is similar to that of early-onset sepsis of any etiology. Prominent respiratory distress can be accompanied by chest radiograph demonstrating the hyaline membrane-appearing pattern of other etiologies such as group B Streptococcus. Septicemia may manifest as apnea, bradycardia, shock, respiratory failure, or other signs of illness. Meningitis and urinary tract infection also can occur. Nursery outbreaks have been reported. Recently, vancomycin-resistant enterococci have emerged as significant pathogens in hospitalized, usually premature neonates.
Staphylococci
Staphylococci have been an important cause of late-onset infections in neonates for 100 years. For most of the last century, methicillin-susceptible Staphylococcus aureus (MSSA) was the organism causing endemic and sporadic disease, in addition to staphylococcal epidemics occurring in 20- to 30-year cycles. In adults, nosocomial methicillin-resistant Staphylococcus aureus (MRSA) infections emerged in the 1980s. Strains of virulent, community-associated, methicillin-resistant Staphylococcus aureus (CA-MRSA), which are distinct from the typical nosocomial MRSA, have been reported since the late 1990s. Recently, methicillin-resistant Staphylococcus aureus (CA-MRSA) has emerged as a significant pathogen in neonatal intensive care units as a cause of late-onset sepsis. Infants with late-onset infection due to Staphyloccocus aureus can present with septic shock characterized most often by respiratory distress, temperature instability, and poor perfusion. Staphylococcal syndromes, such as toxic shock and toxic epidermal necrolysis, also have been reported. A distinctive feature of Staphylococcus aureus infection in the neonate is its ability to frequently and rapidly result in focal pyogenic complications in lungs, skin, soft tissues, bones, and joints. Prolonged bacteremia despite appropriate antimicrobial therapy can occur and suggests other foci of infection, such as endocarditis. Focal infection without bacteremia is less likely to cause fulminant disease but can cause significant morbidity, including cervical adenitis, impetigo, furunculosis, soft tissue abscesses, pneumonia, and pleural empyema. Outbreaks of MRSA skin infection among otherwise healthy, full-term newborns less than 30 days of age have been reported.
With the increased survival of very low birth weight and extremely low birth weight premature infants, coagulase-negative staphylococci (CoNS) have emerged as significant pathogens causing late-onset sepsis. Premature infants are at risk for infection with this commensal organism due to their developmentally immature immune systems and invasive procedures, such as placement of vascular access devices, that further compromise their already poor skin integrity. Other risk factors for CoNS infection include mechanical ventilation, intravenous intralipid use, and duration of total parenteral nutrition. Although CoNS is present on the skin and is usually considered a contaminant in immunocompetent individuals, in the premature infant or in those with vascular catheters, isolation of this organism from the bloodstream is indicative of infection. Clinical signs and features of late- and very-late-onset sepsis caused by CoNS can include apnea, bradycardia, hypoxia, hypotension, temperature instability, serum glucose alterations, soft tissue and joint abnormalities, and other findings. The mortality rate from CoNS infection is approximately 5%.
Listeria monocytogenes
Listeria monocytogenes is a ubiquitous organism and is an important cause of zoonoses. Listeriosis primarily is foodborne and can be found in animal products, including unpasteurized milk and soft cheeses, hot dogs, deli meat, pâté, and undercooked poultry, as well as on unwashed fresh fruits and vegetables. Most people exposed to Listeria do not develop illness, but maternal infection can result in miscarriage or stillbirth, and neonates can develop early or late-onset sepsis and meningitis. Infection in pregnant women occurs most frequently in the third trimester and is associated with an influenza-like illness accompanied by fever, headache, malaise, myalgias, and gastrointestinal tract symptoms in two thirds of infected women. Perinatal listeriosis results in stillbirth or neonatal death in approximately 22% of cases.
In infants with early-onset listeriosis, prematurity, pneumonia, and septicemia are common. Chorioamnionitis and brown-staining amniotic fluid can occur. Mothers can be asymptomatic. Granulomatosis infantisepticum, a diffuse erythematous nodular rash characterized by granulomas on histopathology, can occur in neonates with severe listerial disease. The lesions commonly occur in the liver and placenta but also can be found in the brain, lungs, adrenal glands, kidney, spleen, and gastrointestinal tract.
Late-onset disease caused by Listeria is less common than the early-onset form, but it usually occurs in the perinatal period in term infants and results in meningitis. Frequently, there is no history of pregnancy complications. Neonatal septicemia or meningitis caused by Listeria cannot be distinguished clinically from other infectious causes of sepsis. Patients with impaired cell-mediated immunity are at greatest risk of developing listeriosis.
Escherichia coli
Most infants are colonized with enteric bacilli in the lower gastrointestinal or respiratory tracts during or just before delivery. E coli has a complex antigenic structure with more than 145 different somatic (O), approximately 50 flagellar (H), and 80 different capsular (K) antigens. Despite this genetic diversity of human commensal isolates, a limited number of clones cause neonatal pathology.
E coli strains with the K1 capsular polysaccharide antigen are associated with 40% of cases of septicemia and 80% of cases of neonatal meningitis. The presence, amount, and persistence of K1 antigen in cerebrospinal fluid have been related to a more severe outcome in infants with meningitis.
Neonatal septicemia or meningitis caused by E coli and other gram-negative bacilli cannot be distinguished clinically from other infectious causes of sepsis. Signs of sepsis can include apnea, bradycardia, cyanosis, fever or temperature instability, poor perfusion, lethargy, irritability, poor feeding, vomiting, abdominal distension, diarrhea, jaundice, organomegaly, bulging fontanelle, seizure, or other nonspecific clinical findings.
Nosocomial Gram-Negative Pathogens
Both Klebsiella and Enterobacter species inhabit the gastrointestinal tracts of hospitalized infants and have emerged as significant nosocomial neonatal pathogens. These pathogens more commonly cause late-onset rather than early-onset sepsis. Infections of the bloodstream, central nervous system, lung, urinary tract, skin, and soft tissues can occur. Enterobacter species, especially E sakazakii, have been associated with a severe form of necrotizing meningitis with a high mortality rate (50%). Klebsiella species may account for 20% to 30% of late-onset sepsis, and the mortality rate can approach 30%. Risk factors for infection include prematurity, low birth weight, prolonged rupture of maternal membranes, and instrument or cesarean section delivery. Routine extended spectrum cephalosporin usage in some nurseries has resulted in the emergence of multiple-drug-resistant, in the form of extended spectrum beta-lactamase production, isolates of Enterobacter and Klebsiella species. Infections with these organisms are associated with increased morbidity and mortality.
Citrobacter and Serratia species, also occasional inhabitants of the gastrointestinal tracts of hospitalized infants, can cause sporadic and epidemic clusters of neonatal late-onset sepsis and meningitis. Clinical features can include septicemia, pneumonia, empyema, meningitis, urinary tract infection, skin and soft tissue infection, and bone infection. Like E sakazakii, Citrobacter koseri and Serratia marcescens are associated with necrotizing meningitis and the formation of brain abscesses. The mortality rate following meningitis due to either Citrobacter or Serratia species is estimated at 30%, and most survivors suffer significant neurodevelopmental morbidity. Risk factors for infection include prematurity, low birth weight, mechanical ventilation, indwelling vascular device, prior receipt of antibiotics, intraventricular hemorrhage, and necrotizing enterocolitis. Outbreaks of multi-drug-resistant Serratia marcescens infections in neonates have been reported.
Pseudomonas aeruginosa is a cause of late-onset neonatal sepsis, and infants usually are infected from their environment or their endogenous flora. The clinical presentation can be identical to other bacterial causes of neonatal infection, although infants with Pseudomonas sepsis frequently present with clinically fulminant disease. With rapid disease progression, mortality is approximately 50%. Risk factors for pseudomonas sepsis include prematurity, low birth weight, feeding intolerance, prolonged parenteral hyperalimentation, conjunctivitis, and necrotizing enterocolitis.
Rare Bacterial Etiologies Although non-typhi Salmonella is an uncommon cause of sepsis and meningitis in neonates, a significant number of cases of Salmonella meningitis occur in young infants. Meningitis can be complicated by subdural empyema and communicating hydrocephalus and can result in death.
Another uncommon cause of invasive bacterial infection in neonates is Neisseria meningitidis. This organism can colonize the maternal genital tract and infect the infant at the time of delivery, or intrauterine infection can occur as a result of maternal meningococcemia. Meningococcus can cause early- and late-onset sepsis in neonates. The clinical presentation can include septicemia, meningitis, and conjunctivitis. Purpura is rare. Serogroups B, C, Y, and nongroupable isolates have been reported.
Haemophilus influenzae type b disease in infants in the United States is rare since the introduction of H influenzae type b conjugate vaccines in 1988. In addition, invasive infections caused by nontypeable H influenzae in neonates remain uncommon. The clinical presentation of neonatal H influenzae disease can include septicemia, meningitis, pneumonia, soft tissue or joint infection, otitis media, and mastoiditis. Clinical and epidemiologic characteristics are similar to those of group B Streptococcus (GBS) neonatal disease, including early- and late-onset presentations and a high mortality rate.
Pneumococci are not usually isolated from cultures from the cervix or vagina of pregnant and nonpregnant women. However, S pneumoniae rarely can present as early-onset neonatal sepsis with clinical features similar to those of early-onset GBS infection. The illness can be associated with prolonged rupture of maternal membranes, respiratory distress, abnormal chest roentgenogram, poor peripheral perfusion, hypotension, leukopenia, and rapid clinical decline. Late-onset infection accompanied by meningitis also can occur.
Anaerobes have been isolated from the external genitalia or vaginae of pregnant and nonpregnant women, and newborns are colonized with these organisms at the time of birth. Anaerobes are thought to cause fewer than 5% of the cases of neonatal sepsis, but the true incidence is uncertain. The clinical presentation is indistinguishable from other bacterial causes of sepsis, but predisposing factors include premature rupture of membranes, preterm delivery, maternal amnionitis, sepsis following postoperative complications, and necrotizing enterocolitis. Intrauterine infection can cause septic abortion. Bacteroides and Clostridium species are the most frequent pathogens isolated from neonates with anerobic sepsis, and the mortality rate is estimated at 26%. Neonatal bacteremia caused by C perfringens can present with characteristics seen in adults such as active hemolysis, hyperbilirubinemia, and hemoglobinuria. In addition to causing sepsis and meningitis, Clostridium species can cause localized infection, such as omphalitis, cellulitis, and necrotizing fasciitis.
Miscellaneous Syndromic Infections
Pathogens Causing Conjunctivitis Eyelid edema, hyperemia of the conjunctivae, and purulent discharge are common manifestations of infectious conjunctivitis in neonates. The most common pathogen in infants not treated with topical silver nitrate is Chlamydia trachomatis. Various gram-negative and gram-positive organisms such as Haemophilus species, Neisseria gonorrhoeae, S aureus, enterococci, and S pneumoniae also can cause disease. In hospitalized and premature infants, bacteremia and meningitis can occur in association with conjunctivitis caused by Pseudomonas aeruginosa.
Pathogens Causing Otitis Media Infants with acute otitis media in the newborn period generally are not systemically ill. Signs of upper respiratory tract infection are common, but fever often is absent. The most common pathogens are the same as those found in older infants and children, such as S pneumoniae, M catarrhalis, and H influenzae. Tympanic aspiration reveals that approximately 40% of middle ear aspirates in this age group do not yield a pathogen. In hospitalized and premature infants with otitis media, systemic signs of illness such as fever, poor feeding, vomiting, diarrhea, and abdominal distention are more common than signs of upper respiratory tract infection. Pathogens causing very late onset sepsis, including staphylococci and enteric bacilli, are common.
DIAGNOSTIC EVALUATION FOR BACTERIAL PATHOGENS
The diagnosis of systemic infection in the newborn cannot be reliably established on the basis of clinical findings alone. A history of risk factors for neonatal sepsis often is associated with early-onset infection, but up to 20% of infants who develop early-onset disease are born without pregnancy or delivery complications. The development of late-onset sepsis in the term infant often is accompanied by only subtle signs of infection.
The isolation of a pathogenic microorganism from the blood is the “gold standard” and is the only definitive method of establishing the diagnosis of neonatal septicemia. The optimal volume of blood to obtain for culture is unknown, but 0.75 to 1.0 mL generally is recommended and yields the pathogenic organism in approximately 90% of cases. Multiple blood cultures are not necessary. In those 10% of cases in which the pathogen cannot be cultivated, sepsis can be presumed from the clinical course.
A lumbar puncture for CSF studies is recommended to evaluate for meningitis. A negative blood culture occurs in approximately 15% to 38% of neonates with meningitis. Therefore, in the neonate whose unstable condition precludes the performance of a lumbar puncture, CSF should be obtained and parameters evaluated for partially treated meningitis as soon as the infant’s condition permits.
Urine cultures generally are not useful in the evaluation of the neonate with early-onset sepsis but should be obtained by catheter or suprapubic aspiration when infants are evaluated for sepsis beyond the first few days of life. The yield is 7% to 10% for late-onset disease. Cultures and gram stain of foci of suspected infection are recommended, except in select clinical circumstances, such as brain abscess not amenable to surgery or lung abscess at high risk of fistula formation, where obtaining cultures can be associated with excessive morbidity or even mortality. In general, cultures from surface sites or mucous membranes reveal colonizing microorganisms and correlate poorly with invasive disease. Gastric aspirate and placental cultures provide information about exposure to possible pathogens and may be helpful in guiding empirical therapy in an infant with signs of or risk factors for sepsis.
Screening tests for neonatal sepsis (such as total white blood cell count, percentage of neutrophils or immature forms, platelet count, C-reactive protein level, serum procalcitonin and cytokine levels, etc) are variably useful adjuncts in the evaluation of those with clinical signs of illness. No one screening test or combination of tests is sufficiently sensitive or specific to limit the necessity of performing blood, cerebrospinal fluid, or other appropriate studies, such as chest roenterogram, in infants with signs of or risk factors for infection. Normal screening tests should not deter a clinician from fully evaluating an infant for sepsis; however, screening tests, clinical signs of illness, and risk factors for infection should be used together. A concern in any area should prompt immediate evaluation of the infant and initiation of appropriate antimicrobial therapy.
TREATMENT OF BACTERIAL PATHOGENS
Table 230-3 lists common antibacterial agents used for the treatment of neonatal infections. The choice of empirical antimicrobial therapy for the treatment of suspected sepsis is influenced by factors such as the prevalent organisms responsible for neonatal sepsis by age of onset, patterns of antimicrobial susceptibility of isolates in a particular hospital setting, penetration of an antimicrobial agent into the central nervous system, and potential toxicity of an agent in neonates or a particular neonate. For infants who develop clinical signs of illness during the first few days of life, initial therapy must include agents active against group B Streptococcus (GBS), streptococci, and other gram-positive cocci, Listeria monocyto-genes, and gram-negative enteric bacilli. Treatment of the infant with suspected late-onset sepsis who remains in the nursery must include therapy for nosocomial and commensal pathogens, such as S aureus, coagulase-negative staphylococci (especially in infants with intravascular catheters or cerebrospinal fluid shunts), and potentially multidrug resistant gram-negative bacilli. These infants also remain at risk for sepsis due to maternally acquired etiologic agents.
Empirical antimicrobial therapy for early-onset neonatal sepsis consists of ampicillin and gentamicin in order to provide bactericidal activity against GBS and other streptococci, enterococci, Listeria, E coli, and other gram-negative bacilli. If cerebrospinal fluid (CSF) parameters are concerning for meningitis, cefotaxime is added because of its ability to achieve bactericidal concentrations in the CSF. Gentamicin serum concentrations are not necessary unless therapy is continued beyond 72 hours, renal dysfunction is present, or the neonate is a VLBW infant.
As an initial choice of empirical antimicrobial therapy in infants with presumed septicemia, an aminoglycoside is recommended over a third-generation cephalosporin for the following reasons: third-generation cephalosporins are not active against Listeria and enterococci, superior efficacy with a third-generation cephalosporin has not been demonstrated, routine empirical use of third-generation cephalosporins for neonatal septicemia has been associated with sepsis due to multiple-drug-resistant organisms, and empirical cephalosporin use for treating early-onset neonatal sepsis has independently been shown to be associated with a higher case-fatality rate. Use of cefotaxime should be restricted to those with initial evidence of gram-negative sepsis or meningitis. Ceftazidime should be used if Pseudomonas sepsis is suspected. Continued cephalosporin therapy should be limited to those infants with gram-negative meningitis caused by susceptible organisms or those with ampicillin-resistant enteric infections. Unless it is the only agent effective against the pathogen causing disease, ceftriaxone is not recommended for use in neonates because it can displace bilirubin from serum albumin. The typical duration of therapy for early- or late-onset septicemia is 10 to 14 days with the least toxic, most effective, and preferentially most narrow-spectrum antimicrobial agent based on culture results.
Table 230-3. Initial Antibacterial Therapy for Neonatal Bacterial Infections
For neonates with early-onset meningitis, a third-generation cephalosporin should be administered in addition to ampicillin and gentamicin or to replace gentamicin because it provides concentrations of drug in the cerebrospinal fluid that greatly exceed the minimum inhibitory concentrations of susceptible pathogens, and there is no dose-related toxicity. If gram-negative meningitis is proven, combination therapy with a cephalosporin and an aminoglycoside generally is recommended for the first 7 to 14 days of therapy. Most experts recommend treating meningitis caused by enteric bacilli for a minimum of 21 days.
For suspected late-onset sepsis in the nursery or for sepsis associated with skin, soft tissue, bone or joint infection, initial antimicrobial therapy should provide coverage against commensal species such as coagulase-negative staphylococci (CoNS) and methicillin-resistant Staphylococcus aureus in addition to gram-negative pathogens and nonstaphylococcal gram-positive organisms. Beta-lactamase production is present in most species of CoNS, and they also are resistant to methicillin and cephalosporins. In addition, ampicillin has no activity against S aureus strains. In such cases, vancomycin is substituted for ampicillin and is administered with an aminoglycoside pending further culture data. Except in the cases of neurosurgery, indwelling devices, or intraventricular hemorrhage, staphylococci are rare causes of neonatal meningitis. For empirical therapy of nosocomial meningitis, a third-generation cephalosporin should be added to vancomycin and an aminoglycoside.
In infants in whom infection caused by anaerobic organisms is suspected, such as those with signs of omphalitis, peritonitis, or necrotizing enterocolitis, initiating therapy with a clinically appropriate agent such as clindamycin, metronidazole, piperacillin, or ticarcillin is indicated. Penicillin G is not considered adequate anaerobic coverage for most neonatal infections because B fragilis is usually resistant.
BACTERIAL PATHOGENS
Virtually all infants with neonatal sepsis died before the advent of antibiotics. In the present day, if the evaluation for bacterial sepsis and the initiation of antibacterial therapy are prompt, infants are not likely to experience long-term health problems associated with neonatal bacterial infection. However, if appropriate therapy is delayed because early signs are missed, the mortality rate increases. Cases of fulminant early-onset sepsis are associated with a high mortality rate of 14% to 20% to as much as 70%.
In the 1960s, mortality from neonatal sepsis was approximately 50% but decreased to 10% to 20% in the 1970s and 1980s. The mortality rate for premature infants is higher than that for term infants. A recent report indicated that in preterm infants with group B streptococcal sepsis, the mortality rate was 23% and 9% for early-onset and late-onset cases, respectively. In comparison, 4% of infants with early-onset disease and no infants with late-onset disease died during the same time period. Outcomes in neonates with meningitis is discussed in Chapter 231.
PREVENTION AND CONTROL OF BACTERIAL INFECTIONS
Improvement in the health of pregnant women, increased use of prenatal care facilities, the development of neonatal intensive care expertise and dedicated units, and adherence to proper hand hygiene and disinfection of materials involved in the care of neonates have improved infant outcomes. Improved care of the mother and infant may contribute to a decreased incidence of neonatal infection. The use of antibiotics to prevent neonatal infection can be valuable when a specific pathogen-directed approached is employed for a limited period of time. An example is the application of silver nitrate to the eye or intramuscular administration of ceftriaxone to prevent neonatal gonococcal ophthalmia. Intrapartum chemoprophylaxis of pregnant women with rectovaginal colonization of group B Streptococcus (GBS) has been shown to be a highly effective method of preventing early-onset neonatal GBS disease. Consensus guidelines developed for the Centers for Disease Control and Prevention (CDC) for intrapartum chemoprophylaxis were modified in 2002 and provide recommendations based on maternal screening cultures and risk factors [Centers for Disease Control and Prevention of Perinatal Group B Streptococcal Disease. MMWR 2002;51(No. RR-11):1-22]. The guidelines also state that routine administration of antibiotics to newborn infants who are born to mothers who have received intrapartum chemoprophylaxis is not recommended.
Breastfeeding has been recognized for many years to provide neonates a degree of protection against viral and bacterial pathogens. Breastfeeding can protect infants against neonatal sepsis and meningitis, urinary tract infection, respiratory illness, gastroenteritis, otitis media, and other infections. Uncommonly, breastfeeding can be the source of neonatal bacterial infection.
Infants are somewhat protected from bacterial infection by placental transfer of maternal immunoglobulin G. Adoption of immunization of pregnant women against pathogens causing severe infection in neonates has been studied and recommended on a limited basis. The immunization of pregnant women with tetanus toxoid is widely practiced and, in resource-limited countries, has markedly decreased the incidence of neonatal tetanus.
Numerous studies have explored whether the use of intravenous immune globulin or granulocyte colony-stimulating factor, especially in pre-term infants, can reduce the incidence of sepsis. Analysis of these studies and others reveals that there currently is insufficient evidence to support the routine use of intravenous immune globulin or granulocytes colony-stimulating factor preparations to prevent systemic bacterial infections and mortality in high-risk neonates.
REFERENCES
See references on DVD.
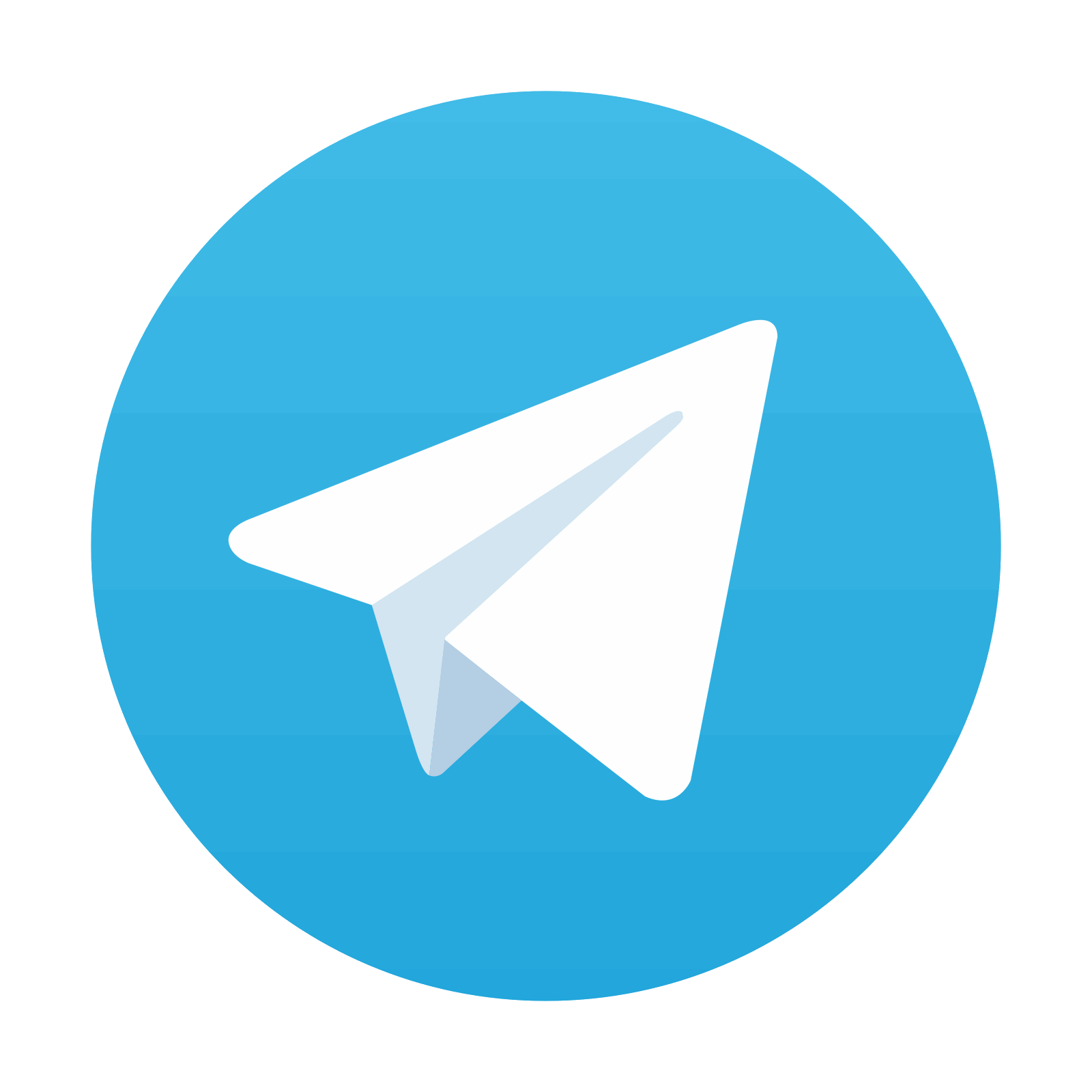
Stay updated, free articles. Join our Telegram channel

Full access? Get Clinical Tree
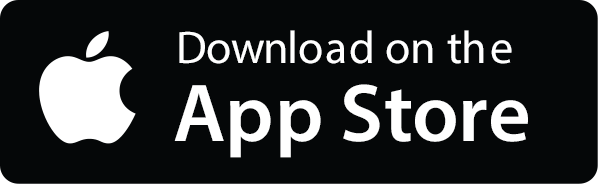
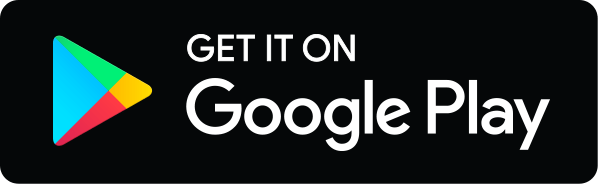