Fig. 23.1
CT scans—apex, hilum, and base—in ARDS from sepsis. Images were taken at 5 cm H2O end-expiratory pressure. The CT scans show heterogeneous disease and both the craniocaudal and sternovertebral gradients (From Gattinoni et al. (2001))
Prone positioning may have a variable effect on improving oxygenation depending on the underlying recruitment status of the patient’s alveolar units. Recruitment normally progresses from ventral to dorsal and from cephalad to caudal (Puybasset et al. 1998). In light of this, one might imagine that prone positioning is less likely to have the same benefit in a derecruited lung as it might in a recruited lung. One study recently examined the effect of prone positioning on alveolar recruitment and oxygenation in a cohort of adult patients with ARDS (Guerin et al. 1999). The investigators found a correlation between change in oxygenation and the recruited lung volume. They hypothesized that one of the mechanisms by which prone positioning improved oxygenation was recruitment. Another study examined the effect of prone positioning on enhancing the outcome of recruitment maneuvers in patients with ARDS. The authors concluded that cyclical sustained inflations during ventilation in the prone position may produce optimal lung recruitment (Pelosi et al. 2003b). Oczenski and colleagues demonstrated a prolonged improvement in oxygenation after performing a recruitment maneuver in patients who were maintained in the prone position for 6 h (Oczenski et al. 2005). These studies support the hypothesis that redistribution of ventilation and enhanced alveolar recruitment is the mechanism of improvement in oxygenation in patients with ARDS. This may have important implications in patients with extrapulmonary ARDS, whose lungs may be more responsive to standard recruitment measures (Lim et al. 2003; Pelosi et al. 2003a). Because patients with secondary ARDS may have more recruitable lungs than those with primary ARDS, they are more likely to respond to prone positioning.
In supine patients, the anterior chest wall is normally more compliant than the posterior chest wall as there is little impediment to anterior movement of the rib cage. When patients are transitioned to the supine position, this anterior chest wall compliance is impeded by the patient’s bed, and the posterior chest wall has a relatively improved compliance. In a study of 16 adults with ARDS, Pelosi and colleagues proposed that these relative differences in chest wall compliance explain the improved alveolar ventilation of dependent lung areas when patients are supine (Pelosi et al. 1998).
Another potential benefit of utilizing the prone position in ARDS is that of limiting ventilator-induced lung injury (VILI). VILI involves various mechanisms, including overdistention through excessive volume delivery (“volutrauma”) or transpulmonary pressure (“barotrauma”) and the phenomenon of alveolar collapse and reinflation (“atelectrauma”), which may lead to a local and systemic inflammatory reaction (Slutsky 1999). In a recent animal model, Valenza and colleagues demonstrated a delay in the progression of VILI with prone positioning (Valenza et al. 2005). Recent animal studies with preinjured lungs ventilated with large tidal volumes demonstrated less histopathologic injury in the prone versus the supine position (Broccard et al. 1997, 2000). In addition, they found that in the supine position, lung injury was primarily located in the dependent lung areas, while in the prone position, lung injury was more homogeneously distributed. These preclinical studies uniformly suggest that the prone position may act to protect the lung from developing VILI, as well as to slow the progression of existing lung injury.
23.4 Effectiveness and Outcome
Most of the large clinical trials of prone positioning in ARDS have been performed in the adult population. In the neonatal and pediatric populations, several investigators have examined small cohorts of patients. These studies as well as a recently published randomized, controlled trial in pediatric patients are summarized in Table 23.1. The initial reports of prone positioning in pediatrics were limited by nonrandom assignment of prone positioning for variable time periods in a heterogeneous population. These limited reports, along with a lack of uniform guidelines to perform prone positioning safely and effectively, led to reluctance among pediatricians to perform this maneuver. Yet, these initial reports did describe dramatic improvements in oxygenation soon after children with ALI/ARDS were placed in the prone position. The improvement in oxygenation and the resultant potential to reduce inspired oxygen concentrations as well as airway pressures have spurred continued study of prone positioning in pediatrics.
Table 23.1
Pediatric studies: prone position
Reference | Year | Level of evidence | Type of study | # of pts | Entry criteria | Timing > lung injury (mean) | Frequency of PP (# of cycles) | % of time in PP | Duration in PP | Duration in SP | Responders | Adverse effects | Outcomes measured |
---|---|---|---|---|---|---|---|---|---|---|---|---|---|
Murdoch and Storman (1994) | 1994 | 3b | 1-center Prospective case series | 7 | ARDS | 4.5 days | 1 | – | 0.5 h | 0.5 h | 100 % | – | CO, HD variables, DO2 |
Numa et al. (1997) | 1997 | 3b | 1-center Prospective case series | 30 | Intubated pts Control = 10 Obstructive = 10 Restrictive = 10 | 12 days | 1 | – | 70 min | Rest of the day | -NA- | – | ABG, FRC, CRS, RRS |
Curley et al. (2000) | 2000 | 3b | 1-center Prospective case series | 25 | ALI/ARDS | <19 h | 1–12 | 47 % | 20 h/day | 4 h/day | 84 % | St II Skin ulcers in 24 % | PaO2 to FiO2 ratio, mortality (study, 28-day), adverse effects, COMFORT score |
Kornecki et al. (2001) | 2001 | 3b | 1-center Prospective case series | 10 | ARF OI >12 FiO2 >0.5 For 12 h | 1 | 12 h/day | 12 h/day | 90 % | Facial edema in 30 % | HD, OI, Static C RS and R RS | ||
Casado-Flores et al. (2002) | 2002 | 3b | 1-center Prospective case series | 23 | ALI/ARDS | 56 h | 2–14 | 36 % | 8 h | 8 h | 78 % | – | PaO2 to FiO2 ratio |
Haefner et al. (2003) | 2003 | 4 | 1-center Retrospective cohort | 63 | ECMO for resp failure | – | 15 position changes | – | – | – | -NA- | Adverse effects recorded | |
Relvas et al. (2003) | 2003 | 4 | 1-center Retrospective chart review | 40 | ARDS | 107 h | Variable | – | >20 h (n = 37) | – | – | – | OI, PaO2 to FiO2 ratio, |
Curley et al. (2005) | 2005 | 1b | Randomized, multicenter study | 102 | ALI/ARDS | <48 h | Up to 7 | 20 h/day | 4 h/day | Ventilator-free days, mortality, time to recovery from lung injury, organ-failure-free days, functional outcome |
Key components to consider in the studies on pediatric prone positioning to date include patient selection, the timing and duration of prone positioning, and outcome measurement. Patient selection for prone positioning is challenging and may be integral to understanding the true benefit and correct application of the intervention. One important factor in patient selection is that acute lung injury from different etiologies does not share the same morphological, mechanical, and clinical characteristics. For years, investigators of adult ALI and ARDS have debated the clinical significance between pulmonary and extrapulmonary etiologies of lung injury (Callister and Evans 2002; Pelosi and Gattinoni 2001). Some assert that the two conditions are different entities, with different pathophysiology, radiographic appearance, and respiratory mechanics (Pelosi et al. 2003a). The two entities may also have different responses to prone positioning. Indeed, Pelosi and colleagues have reported that secondary ARDS, with associated diffuse atelectasis, may be more responsive to prone positioning (Pelosi et al. 2002). Primary ARDS, characterized by altered lung elastance, may not benefit from strategies such as increased PEEP, lung recruitment, and prone positioning, compared to patients with secondary, or extrapulmonary, ARDS, which may be characterized more by altered chest wall elastance. It is important to note, however, that the difference in the two conditions in terms of the impact of different therapies is unknown. Indeed, a recent study revealed no difference in lung functional assessment 6 months after hospital discharge in adult patients with pulmonary versus extrapulmonary ARDS (Suntharalingam et al. 2001).
The overall length of mechanical ventilation is important when considering patient selection in studies on prone positioning. In 1997, Numa and colleagues examined the effect of prone position on functional residual capacity (FRC), oxygenation, and respiratory mechanics in mechanically ventilated children with restrictive and obstructive lung disease and in controls (Numa et al. 1997). The investigators found a pattern of increased FRC in the prone position in each of the three subgroups, though this improvement did not reach statistical significance. There was no significant improvement in oxygenation in any of the groups. One important caveat to the study is that the authors enrolled patients after prolonged periods of mechanical ventilation (up to 2 weeks), and this may have influenced the physiologic effects that prone positioning had on this population.
Outcome measures are important to consider when evaluating studies on ARDS. Traditionally, the degree of oxygenation has been the main surrogate of the severity of lung injury in studies on ARDS. This holds true in studies evaluating the role of prone positioning. Pediatric studies evaluating the role of prone positioning in ARDS have used indices of oxygenation such as the oxygenation index (OI) and the PaO2/FiO2 (P/F) ratio as outcome measures (Casado-Flores et al. 2002; Curley et al. 2000; Kornecki et al. 2001). In a prospective case series of 25 pediatric patients with ARDS, an immediate and cumulative improvement in oxygenation was demonstrated after prone positioning (Curley et al. 2000). The study included patients from 2 months to 17 years that were enrolled within 19 h of meeting entry criteria. Patients were placed prone for 20 h each day and returned to the supine position for 4 h, accounting for 47 % of the time on mechanical ventilation. Indices of oxygenation, including P/F ratios and OI, are shown in Fig. 23.2 at four time points in the first 24 h of prone positioning. Both P/F ratio and OI significantly improved at both 1 h and up to 19 h after prone positioning. Based on the response to prone positioning in the first 24 h, the investigators then classified patients as immediate responders (n = 11, 44 %) and immediate nonresponders (n = 14, 56 %). In the immediate responders, oxygenation improves early and is sustained during the prone period (Fig. 23.3). Even after this subgroup of patients (immediate responders) returned to the supine position, they exhibited preserved improvement in oxygenation. Nonresponders were found to have a delayed and non-sustained improvement in oxygenation. The authors reported an overall 84 % rate of response to prone positioning in this population of children. Casado-Flores and colleagues also showed an improvement in oxygenation with prone positioning in a population of 23 children with ARDS (Casado-Flores et al. 2002). In contrast to the previously summarized study, they showed that the improvement in oxygenation was not sustained after patients returned to the supine position. Overall, studies using oxygenation as an outcome for response to prone positioning have shown variability in response and an inability to predict which patients might respond to the intervention.
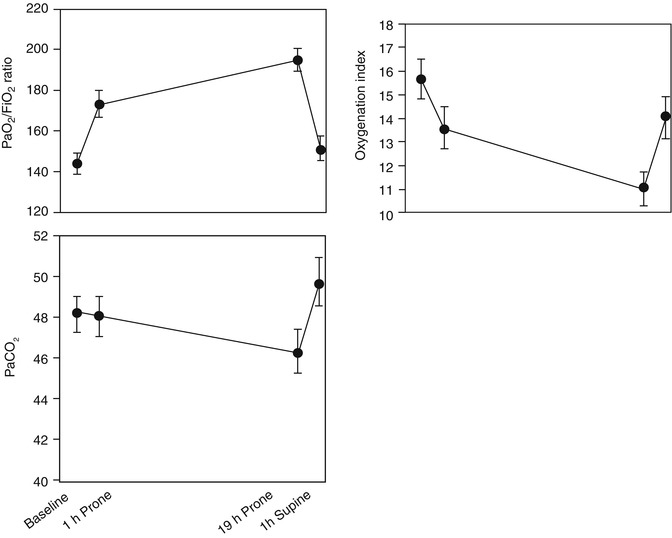
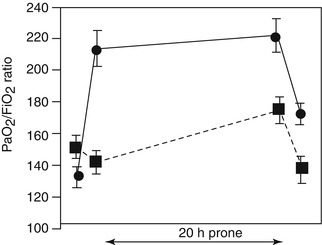
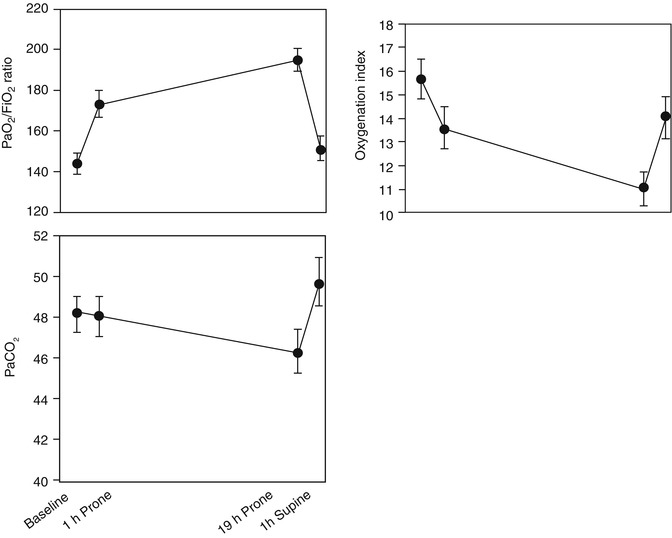
Fig. 23.2
P/F ratio, OI, and PaCO2 at four time points in first 24 h of prone positioning. Day 1 PaO2/FiO2 ratio, OI, and PaCO2. Top: The PaO2/FiO2 ratios among the four data collection points were significantly different (p = 0.006). The PaO2/FiO2 ratio significantly increased (p = 0.04) from 143 ± 10 mmHg at baseline in the supine position to 173 ± 14 mmHg after 1 h in the prone position. It continued to increase significantly (p = 0.005) to 194 ± 15 after 19 h in the prone position. The PaO2/FiO2 ratio at hour 21 in the supine position (150 ± 11 mmHg) was not significantly different from that at baseline. Middle: The OIs among the four data collection points were significantly different (p = 0.01). The OI significantly decreased (p = 0.05) from a baseline value in the supine position of 15.7 ± 1.7–13.6 ± 1.6 after 1 h in the prone position and continued to decrease significantly (p = 0.008) to 10.9 ± 1.5 after 19 h in the prone position. The OI at hour 21 in the supine position (14 ± 1.9) was not significantly different from that at baseline. Bottom: PaCO2 values were not significantly different among the four data collection points (From Curley et al. (2000))
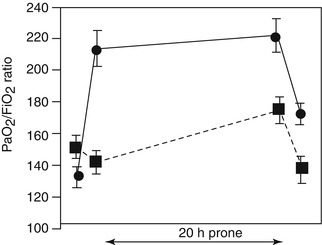
Fig. 23.3
The PaO2/FiO2 ratio was significantly different between the two subgroups (p = 0.05) over the four data collection points (p = 0.003) and between the two subgroups over the four data collection points (p = 0.03). Immediate responder group (•): The PaO2/FiO2 ratio significantly increased (p = 0.003) from a baseline value in the supine position of 134 ± 11–213 ± 21 mmHg after 1 h in the prone position. Significant (p = 0.02) but not cumulative increases in oxygenation were seen after 19 h in the prone position (220 ± 25 mmHg). The PaO2/FiO2 ratio at hour 21 in the supine position (170 ± 12) was significantly better than that at baseline in the supine position (p = 0.02). Immediate nonresponder group (■): The PaO2/FiO2 ratio did not significantly increase from a baseline value in the supine position of 152 ± 16–141 ± 13 mmHg after 1 h in the prone position. Significant (p = 0.02) and cumulative increases in oxygenation were seen after 19 h in the prone position (173 ± 15 mmHg). The PaO2/FiO2 ratio at hour 21 in the supine position (135 ± 15 mmHg) was not significantly better than that at baseline in the supine position. Note that with the exception of increasing the FiO2 to keep Spo2 at >85 %, ventilator settings were held constant during the 1-h supine-to-prone and prone-to-supine repositioning (From Curley et al. (2000))
It has been demonstrated in a number of clinical trials in adults with ARDS that improved oxygenation is not associated with improved clinical outcomes (ARDSnet 2000; Brower et al. 2004; Doyle et al. 1995). The only randomized, controlled trial of the prone position in pediatric patients that evaluated relevant clinical outcomes as well as gas exchange was published in 2005 (Curley 2005). The study population included 102 patients, aged 2 weeks to 18 years, from seven pediatric intensive care units who were enrolled within 48 h of meeting criteria for ALI or ARDS. To ensure uniform co-interventions among the patients, the two groups were managed using identical ventilator protocols, extubation readiness testing, sedation protocols, and guidelines related to hemodynamics, nutrition, and skin care. Patients in the prone positioning group spent 20 h/day in the prone position, for a maximum of 7 days. The primary outcome was ventilator-free days, and the secondary outcomes included mortality, time to recovery from lung injury, and organ-failure-free days. The authors did not demonstrate significant differences in either the primary outcome or any of the secondary outcome variables. The study was designed to enroll patients early in their presentation and to follow them throughout the acute phase, with patients placed prone on average of 28 h after fulfilling eligibility requirements and being treated for 79 % of the acute phase of the illness. Consistent with an earlier study on the prone position (Curley et al. 2006), this study demonstrated that 90 % of patients managed in the prone position were categorized as responders, defined by an improvement in P/F ratio or OI. Despite a robust study design, which included early enrollment, algorithms for all relevant interventions likely to affect the primary outcome, and a high rate of compliance with all treatment protocols, the study failed to show a clinically relevant benefit of prone position in the treatment of pediatric ARDS or ALI.
A recent meta-analysis of studies on prone positioning in both adult and pediatric ARDS revealed no significant improvement in mortality (Abroug et al. 2008). A meta-analysis of adult trials also demonstrated no significant overall improvement in mortality, though there was a significantly improved mortality in patients with higher severity of illness (Alsaghir and Martin 2008). No studies have demonstrated that prone positioning in patients with ARDS improves mortality, though one recent trial has shown a trend toward improved 60-day survival in adults (Fernandez et al. 2008).
Another important consideration when evaluating pediatric studies on prone positioning is the duration of prone positioning. The timing of prone positioning ranges from a single 30-min prone maneuver in one study to other studies that place patients prone from 8 to 24 h a day (Murdoch and Storman 1994). Some studies report early improvement in hypoxemia in patients who respond to prone positioning. Relvas and colleagues performed a retrospective analysis of patients with ARDS placed in the prone position and compared the OI prior to the intervention to OI values after brief (6–10 h) and prolonged (18– 24 h) periods of prone ventilation (Relvas et al. 2003). The patients placed in a prone position for a prolonged period of time demonstrated a more pronounced and stable reduction in OI than did patients placed prone for brief periods (Curley et al. 2006; Kornecki et al. 2001).
23.5 Indications and Contraindications
Because prone positioning has not been shown to have a positive impact on clinical outcomes, it should not routinely be used in the care of pediatric patients with ALI and ARDS. Prone positioning may be used in patients with ARDS and refractory hypoxemia as a recruitment maneuver. Particularly in patients with extrapulmonary ARDS, whose lungs may be more “recruitable,” prone positioning may prove helpful. The duration of prone positioning may be limited by practical considerations that include patient size and clinical status. Patients who do not show an immediate oxygenation response may not be good candidates for continued prone positioning.
In some patients, prone positioning may not be a practical intervention. This may include patients who are unable to be positioned due to body habitus (e.g., extreme obesity or recent abdominal surgery), patients unable to tolerate prone positioning (e.g., neurologic trauma), and patients undergoing therapies that make the changes in position challenging (e.g., extracorporeal membranous oxygenation or continuous venovenous hemofiltration). Compared to adults, pediatric patients may be more safely placed in the prone position due to their size (Fineman et al. 2006).
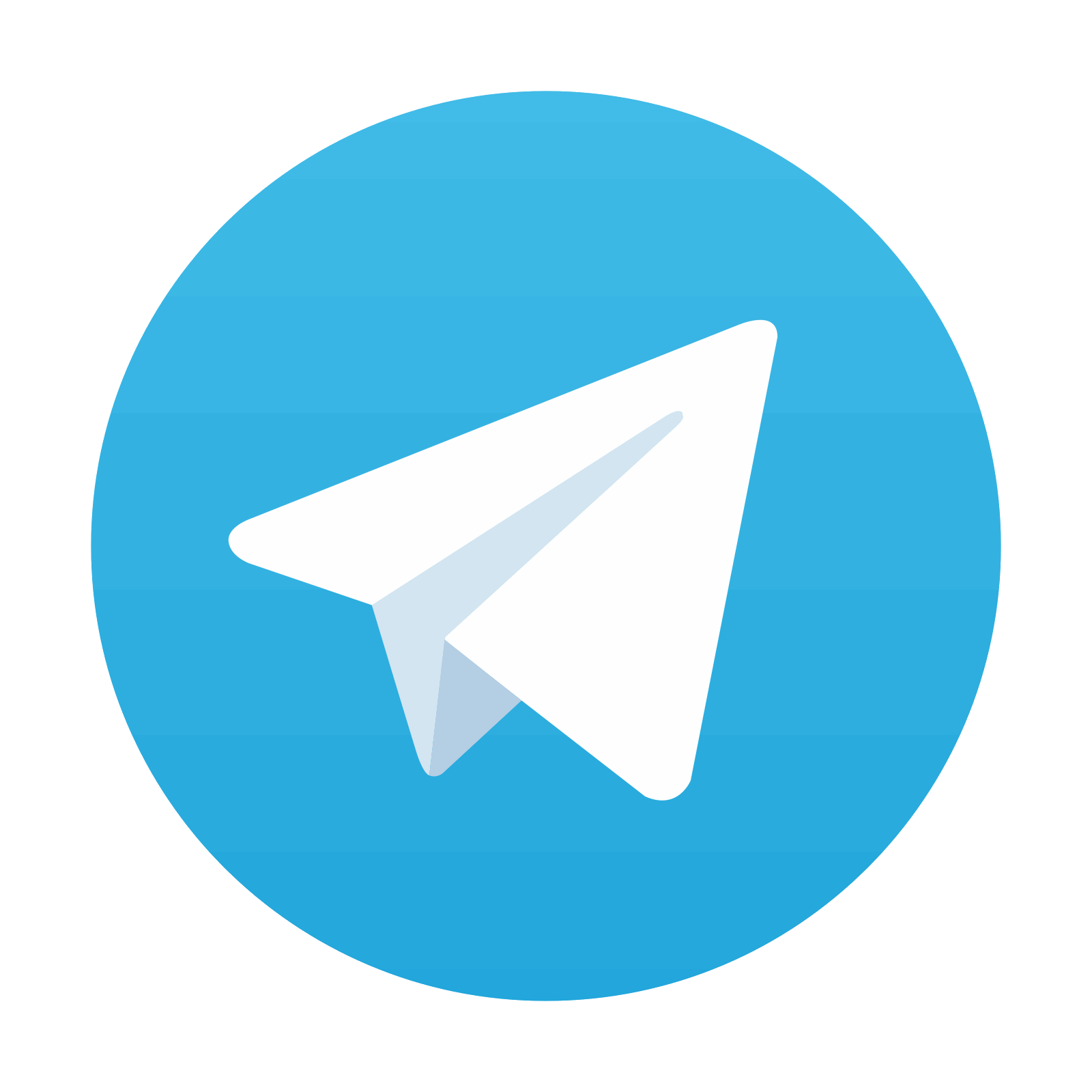
Stay updated, free articles. Join our Telegram channel

Full access? Get Clinical Tree
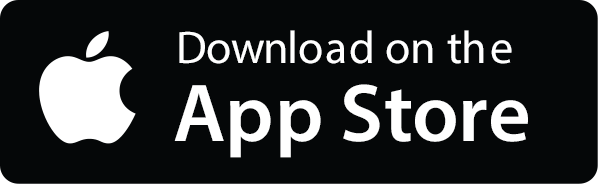
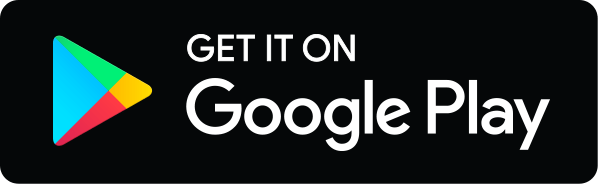