Uterine contractions in labor result in a 60% reduction in uteroplacental perfusion, causing transient fetal and placental hypoxia. A healthy term fetus with a normally developed placenta is able to accommodate this transient hypoxia by activation of the peripheral chemoreflex, resulting in a reduction in oxygen consumption and a centralization of oxygenated blood to critical organs, namely the heart, brain, and adrenals. Providing there is adequate time for placental and fetal reperfusion between contractions, these fetuses will be able to withstand prolonged periods of intermittent hypoxia and avoid severe hypoxic injury. However, there exists a cohort of fetuses in whom abnormal placental development in the first half of pregnancy results in failure of endovascular invasion of the spiral arteries by the cytotrophoblastic cells and inadequate placental angiogenesis. This produces a high-resistance, low-flow circulation predisposing to hypoperfusion, hypoxia, reperfusion injury, and oxidative stress within the placenta. Furthermore, this renders the placenta susceptible to fluctuations and reduction in uteroplacental perfusion in response to external compression and stimuli (as occurs in labor), further reducing fetal capillary perfusion, placing the fetus at risk of inadequate gas/nutrient exchange. This placental dysfunction predisposes the fetus to intrapartum fetal compromise. In the absence of a rare catastrophic event, intrapartum fetal compromise occurs as a gradual process when there is an inability of the fetal heart to respond to the peripheral chemoreflex to maintain cardiac output. This may arise as a consequence of placental dysfunction reducing pre-labor myocardial glycogen stores necessary for anaerobic metabolism or due to an inadequate placental perfusion between contractions to restore fetal oxygen and nutrient exchange. If the hypoxic insult is severe enough and long enough, profound multiorgan injury and even death may occur. This review provides a detailed synopsis of the events that can result in placental dysfunction, how this may predispose to intrapartum fetal hypoxia, and what protective mechanisms are in place to avoid hypoxic injury.
In the majority of women, placental function is sufficient to allow appropriate growth of the fetus throughout pregnancy and to help shield it from the hypoxic stresses of labor. In some women, depending on the degree of placental dysfunction, fetal growth restriction may ensue or intrapartum fetal compromise may develop if the placenta is not able to meet the extra fetal demands required during the last few weeks of pregnancy or during labor and thereby predispose these infants to hypoxic insults. If severe enough, these can result in emergency operative birth, death, short-term morbidity, and significant long-term neurodevelopmental issues.
Globally, perinatal hypoxia remains a major contributor to stillbirth, hypoxic ischemic encephalopathy (HIE), and cerebral palsy; 10% of the global burden of disease is attributable to newborn conditions. It is estimated that worldwide, 23% of the 4 million neonatal deaths per year occur because of intrapartum complications. Of newborns who develop HIE, almost 1 million die in the first month of life and 25% of survivors have long-term sequelae such as cerebral palsy with intrapartum hypoxia, accounting for 1 in 5 cases in term infants. Indeed, the World Health Organization estimates that disability-adjusted life years due to neonatal encephalopathy, birth asphyxia, and birth trauma are comparable with congenital malformations, type II diabetes, and HIV/AIDS (54,400 million).
Although rare, unpredictable and acute catastrophic events such as placental abruption, uterine rupture, or cord prolapse are associated with a high risk of severe fetal hypoxia; 75% of cases in labor occur gradually as a result of uterine contractions. Uterine contractions reduce uteroplacental perfusion by as much as 60% and although most fetuses are able to tolerate this reduction in placental perfusion, there exists a cohort that are unable to and are at risk of hypoxic injury. Fetuses that are unable to withstand these periods of intermittent hypoxia manifest their compromise in a variety of ways, including heart rate abnormalities (decelerations, decrease in beat-to-beat variability etc) and passage of meconium in utero. In extreme cases, if the hypoxic insult is severe enough, brain injury or death can ensue. This review will cover the physiology of placental development, fetal response to intrapartum hypoxia at term, and some recent advances in the screening and identification of vulnerable fetuses.
Placental Development
Development of the placenta involves 2 complex but concurrent processes: endothelial invasion of the maternal spiral arteries by cytotrophoblast (CT) cells and development of the fetal vascular tree. Endothelial invasion initially results in the formation of a trophoblast “plug,” which results in a relatively hypoxic milieu (oxygen partial pressure [PaO 2 ] <20 mm Hg) within the intervillous space. This hypoxic environment is crucial for early regulation of CT proliferation and differentiation. The CT plug dissipates after 10 weeks of gestation, resulting in increased placental blood flow and PaO 2 . Endovascular invasion by the CTs into the wall of the spiral arteries involves de-differentiation of adhesion molecules and conversion from an epithelial to endothelial phenotype. By the late second trimester, the inner third of the myometrial spiral arteries are exclusively lined by CT endothelial cells, replacing the normal vascular smooth muscle with a noncontractile matrix that is nonresponsive to neuronal and hormonal stimuli. These remodeled vessels dilate 4-fold at their terminal portion, into low-resistance, high-capacitance channels capable of increasing the supply of oxygen and nutrients to the fetus to meet its growing demands through gestation. This process is mediated by the interplay of several paracrine factors, including vascular endothelial growth factor, soluble fms-like tyrosine kinase-1, and insulin-like growth factor peptides I and II, which are in turn modulated by pregnancy-associated plasma protein (PAPP-A) produced by trophoblast cells. Low PAPP-A levels are associated with decreased bioavailability of insulin-like growth factor peptides, limiting trophoblastic invasion and a range of pregnancy disorders associated with abnormal placentation.
Concurrent to trophoblast invasion is the development of new vessels within the placenta. This occurs through the processes of vasculogenesis (differentiation of mesenchymal-derived hemangioblasts into endothelial cells) and angiogenesis (remodeling and expansion of existing vessels either by branching or non-branching processes), which are modulated by vascular endothelial growth factor in first half of pregnancy and placental growth factor (PlGF) after 23 weeks gestation.
Failure of endovascular invasion of the spiral arteries produces a high-resistance, low-flow circulation that predisposes to hypoperfusion, hypoxia, reperfusion injury, and oxidative stress in the placenta. This affects placental function through several mechanisms—the persistent high-pressure flow through the intervillous space (2-3 m/s compared with 10 cm/s in normally dilated vessels) results in increased sheer stress and damage to the syncytiotrophoblasts lining the chorionic villi, thereby compromising the functional capacity of the villi for gas and nutrient exchange. Failure of spiral artery conversion renders these vessels susceptible to adrenergic stimulation and vasoconstriction, resulting in fluctuations in intervillous PaO 2 and placental hypoxia–reperfusion injury. Failure of CT endovascular invasion also results in retention of a “functional sphincter,” an innervated stricture point at the junction of the uterine mucosa, predisposing to hypoxia, reperfusion injury, and oxidative stress. Aberrant development of the fetal vascular tree within the placenta, as a consequence of dysregulation of the angiogenic and antiangiogenic factors at the maternal–fetal interface, further compromises placental function and has been implicated in the development of preeclampsia, fetal growth restriction (FGR), gestational diabetes mellitus, placental abruption, preterm labor, and intrapartum fetal compromise (IFC).
Effect of Uterine Contractions on Uteroplacental Perfusion
Uterine contractions during term labor result in a rise in intrauterine pressure of up to 25–70 mm Hg, compromising placental perfusion and oxygenation. Because of a reduction in uteroplacental perfusion, intermittent placental hypoxia, reperfusion injury, and oxidative stress occurs, with accelerated trophoblast apoptosis and enhanced endoplasmic reticulum stress. In vitro and in vivo studies have demonstrated that repeated ischemia–reperfusion events, as seen in labor, result in a substantial decline in placental mRNA and PlGF levels, which in turn further activates inflammatory cytokine pathways. Placentae that are at greatest risk of ischemia–reperfusion injury are those with aberrant conversion of spiral arteries, resulting in narrower vessels with luminal atherosclerotic deposits and retention of vascular innervation and vasoreactivity.
Impact of Labor on the Fetus
Although uterine contractions result in a decline in fetal PaO 2 by approximately 25%, the majority of appropriately grown, healthy, term fetuses are able to withstand the effects of this over a prolonged period of time. Indeed, normal fetuses can cope with a reduction in PaO 2 of up to 50% (from a PaO 2 above 20 mm Hg to as low as 10–12 mm Hg) because of their high myocardial glycogen stores, the presence of vascular shunts, the increased oxygen affinity of HbF, and near-maximal basal cardiac output. The cornerstone of the fetal defense against hypoxic injury lies in the peripheral chemoreflex, triggered in response to fetal hypoxia when uteroplacental perfusion declines by more than 50%. This has the sole aim of rapidly reducing oxygen consumption and centralizing blood flow to the critical organs needed for preservation of life and sensitive to hypoxic injury (the brain, heart, and adrenal glands). The fetal adaptations, including the peripheral chemoreflex, to transient hypoxia are summarized in Figure 1 .

Acute hypoxemia, detected by the carotid body chemoreceptors, stimulates the brain stem to increase both the parasympathetic and sympathetic outflow. Parasympathetic tone predominates, resulting in a decrease in the fetal heart rate (FHR), prolonging end-diastolic ventricular filling time, and subsequently end-diastolic volume. The greater ventricular stretch increases sarcomere length, tension, and contractility (Frank–Starling mechanism), maintaining fetal cardiac output despite the decline in FHR. In addition, the reduction in FHR reduces myocardial oxygen consumption as well as allowing increased transit time of red cells through tissue beds (including the placenta), thereby permitting increased oxygen extraction from HbF and transplacental oxygen transfer to the fetal circulation. Increased sympathetic outflow induces profound peripheral vasoconstriction, resulting in hypertension and centralization of blood flow to critical organs as well as balancing the parasympathetic effects with a positive chronotropic effect, leading to a partial recovery in FHR. Furthermore, peripheral vasoconstriction increases the descending aortic pressure, increasing right ventricular afterload that in turn encourages passage of blood from the right atrium, through the foramen ovale, into the left atrium and thereafter the left ventricle, causing an increase in blood flow into the ascending aorta and cerebral and coronary circulations.
The initial neuronal triggered peripheral vasoconstriction is then maintained by humoral factors, including adrenal catecholamines, arginine vasopressin, cortisol, angiotensin II, and neuropeptide Y, which prolong the redistribution of cardiac output, thus maintaining perfusion to critical organs.
In addition, acute hypoxia stimulates an increase in plasma adenosine and to a lesser extent nitric oxide and prostinoids. These potent vasodilators further increase cerebral blood flow independent of the centralization of cardiac output, with adenosine accounting for more than one-half of this increase.
With uterine relaxation, there is rapid return of efficient placental gas exchange, restoring fetal PaO 2 levels and removing lactate and carbon dioxide. In addition, the fetus is able to efficiently metabolize lactate (by oxidation and conversion to nonessential amino acids and lipids), rapidly reversing the metabolic acidosis that occurs during the preceding contraction. These processes allow the autonomic fetal response to be reactivated during the next contraction, ensuring protection from the repeated hypoxic episodes that characterize labor.
When and why does IFC occur?
It has now clear that fetal decompensation in labor occurs because of the infant’s inability to respond to the peripheral chemoreflex to maintain cardiac output. IFC may occur when there is inadequate reperfusion time between contractions (eg, during uterine hyperstimulation) or when there is appropriate reperfusion time but suboptimal placental function to allow adequate oxygen transfer to the fetus.
Impaired placental development and function renders the fetus vulnerable to intrapartum hypoxia through several mechanisms. First, a greater reduction in uteroplacental perfusion occurs for any rise in intrauterine pressure due to the smaller surface area of the unconverted spiral arteries. Consequently, the degree of fetal hypoxia is greater with each contraction than that which occurs in a fetus with a normally developed and functioning placenta. Furthermore, inadequate placental function is associated with the progressive development of severe fetal acidosis, even with adequate time between contractions, due to an inability to correct the impaired gas exchange between contractions.
Women with pre-labor placental dysfunction are more likely to develop IFC. These fetuses are less likely to withstand intrapartum hypoxia due to lower glycogen stores before the onset of labor, limiting their ability to transition to anaerobic metabolism. As even short periods of hypoxia can quickly deplete cerebral and cardiac glycogen by up to 80%, any limitation in placental transfer of nutrients and oxygen renders these fetuses vulnerable to IFC and injury. Progressive hypoxia in labor results in a further reduction of myocardial glycogen stores, impaired cardiac function, and, ultimately, profound systemic hypotension and irreversible multiorgan injury. Cardiac function is further aggravated by the associated systemic hypotension, limiting coronary blood flow and causing subendocardial myocardial injury. Jones et al recently demonstrated that infants with HIE have elevated troponin-T levels, a marker of myocardial cell death. As myocardial dysfunction progresses with worsening cerebral and in particular brain stem hypoxia, the fetal parasympathetic response may fail altogether.
The magnitude of the hypoxic insult correlates with the fetal response. Siristatidis et al demonstrated that the “brain-sparing” response is only activated when fetal PaO 2 falls below 37%. However, if the PaO 2 falls below 30% for more than 2 minutes, this response fails altogether and significantly increases the risk of adverse perinatal outcomes.
The impact of intrapartum hypoxic injury
The fetus adapts to hypoxia by preferentially redistributing its cardiac output, mediated by neuroendocrine mechanisms, to its coronary arteries, adrenal glands (for production of catecholamines critical for sustaining circulation), and brain. Occasionally, if the hypoxic insult is severe or prolonged enough, these protective mechanisms fail, and profound multiorgan injury or death can occur.
Hypoxic ischemic encephalopathy
The fetal brain is particularly sensitive to hypoxic injury and oxidative stress due to its high rate of oxygen consumption, lack of glucose stores (therefore all cellular events that are adenosine triphosphate and oxygen dependent rapidly fail), high lipid content (rendering neurons susceptible to lipid peroxidation in an autocatalytic fashion triggered by oxidative stress, leading to structural and function damage), and relatively low concentrations and activity of antioxidant enzymes. HIE is defined as neonatal encephalopathy (cerebral obtundation with disturbed neurological function) secondary to systemic hypoxia and reduced cerebral perfusion, resulting in ischemic injury that may be focal or diffuse. In one study, 51% of neonates with moderate or severe encephalopathy died or developed cerebral palsy).
The mechanisms of hypoxic cerebral damage are complex and not entirely mediated by the initial hypoxic insult but are compounded by injuries occurring during the reperfusion phase due to toxicity from reactive oxygen species and excessive stimulation of N-methyl-D-aspartate-type glutamate receptors. Indeed, the severity of the secondary injury occurring during the reperfusion phase correlates best with the severity of neurodevelopmental disability at ages 1 and 4 years. There is a strong association between hypotension and hypoxia with fetal injury, particularly neuronal damage and fetal death. During hypoxia, blood flow to the cerebral hemispheres is reduced, whereas perfusion to the basal ganglia, thalamus, and brainstem is increased. Animal studies have demonstrated that episodes of intermittent hypoxia accompanied by recurrent fetal hypotension cause neuronal loss in the “watershed” zones of the brain (the parasagittal sinus, cerebellar neocortex, and the dorsal horn of the hippocampus). By comparison, hypoxia without hypotension predisposes to cerebellar injury. Animal studies suggest that, even if the total duration of hypoxia is the same, repeated severe episodes cause greater neurologic injury than a single severe prolonged event. This is consistent with recent evidence showing that the FHR deceleration area of the cardiotocograph (ie, a composite of duration and severity) was most discriminative of acidemia in term infants. In addition, the pattern of neuronal damage is further influenced by the pre-labor metabolic and growth status of the fetus, as well as metabolic and nutritional status of the mother.
Noncerebral consequences of intrapartum hypoxia
After the brain, the renal system is the most common organ system to be injured following severe intrapartum hypoxia, with up to 64% of hypoxic term infants developing renal dysfunction usually secondary to acute tubular necrosis. Severely hypoxic infants often require dialysis to support renal function, with an associated mortality rate of between 14% and 70%.
Between 25% and 50% of hypoxic infants experience pulmonary complications and up to 20% require mechanical ventilation. Both hypoxia and hyperoxia (from oxygen therapy and ventilation in the neonatal period) impair alveolar development and predispose to persistent pulmonary hypertension and reactive airway disease, which may persist into adulthood.
Cardiac complications occur in up to 29% of infants with severe intrapartum hypoxia with evidence of myocardial ischemia on electrocardiogram seen in almost 1 in 5 cases. Other organ systems, including hepatic (35%) and gastrointestinal (5%), may be affected. More than one-half of infants with HIE have multiorgan dysfunction. There are many factors that influence the severity of neonatal morbidity following intrauterine hypoxia as well as our ability to predict the likelihood of hypoxia-related complications. Low et al demonstrated that although the range and severity of neonatal complications increased with the degree of metabolic acidosis, there was a great deal of heterogeneity between fetuses, a likely reflection of the nature and length of asphyxial event.
Pre-labor identification of the “at-risk” fetus
There is now evidence that some apparently normally grown fetuses have circulatory changes including cerebral redistribution similar to that seen in FGR. These fetuses are particularly vulnerable to intrapartum hypoxia and compromise.
Low birth weight at term is a major risk factor for emergency operative birth for IFC, perinatal death, and serious neonatal morbidity even in low-risk pregnancies, partly due to the proportion of small for gestational age (SGA) babies that are growth restricted. As not all growth-restricted fetuses are SGA, parameters other than standard biometry are required to identify these at-risk infants before birth. Cochrane reviews do not support the use of either routine late pregnancy ultrasound or umbilical artery Doppler assessment in low-risk populations. However, these reviews are limited by small sample size, use of surrogate outcomes, and most importantly, ultrasound scans that were often performed too remote from term, thereby potentially missing late-onset growth restriction.
More recently, the fetal cerebroplacental ratio (CPR) (ratio of the middle cerebral artery pulsatility index to the umbilical artery pulsatility index) has been proposed as a proxy for intrauterine growth with a low CPR indicative of suboptimal fetal growth. A low CPR (variably defined as <1.0, <5th centile, <10th centile, or <0.67 Multiples of Median [MoM]) is associated with a range of adverse perinatal outcomes, including neonatal intensive care admission, acidosis, and emergency operative birth for IFC. However, despite the broad association with adverse outcomes, the predictive utility of the CPR, particularly in a non-SGA cohort at term, remains modest, and caution should be exercised before incorporating its routine use into clinical care.
Biochemical markers
Low PAPP-A levels are indicative of failure of trophoblast invasion and placental dysfunction and associated with an increased risk of FGR, preeclampsia, intrauterine fetal demise, placental abruption, preterm birth, emergency cesarean delivery for IFC, and neonatal acidosis. Although low maternal PlGF levels in the third trimester have been shown to be associated with IFC and adverse neonatal outcomes, the evidence is conflicting with another recent study, suggesting that it did not predict cesarean birth for fetal compromise.
Identification of intrapartum fetal compromise
Despite concerted efforts to develop accurate techniques for the identification of intrapartum fetal hypoxia, to date all techniques currently in clinical practice have poor positive predictive values. Reviews of conventional antepartum cardiotocography (CTG), computerized FHR analysis, or intermittent auscultation have concluded that they do not result in improved perinatal outcomes. The CTG has poor characteristics as a test: interpretation is subjective, it has poor intra- and interobserver agreement, and lacks discriminatory power in identifying truly hypoxic fetuses. Despite these limitations, it remains the mainstay for intrapartum fetal monitoring despite having a false-positive rate as high as 99.8%. Although the use of intrapartum electronic FHR monitoring has resulted in a reduction in the incidence of neonatal seizures, there is no improvement in perinatal death or cerebral palsy rates despite an increase in operative births. The implementation of CTG monitoring use and interpretation guidelines has nevertheless reduced the incidence of HIE. However, the use of computerized CTG analysis software has failed to demonstrate any significant improvement in maternal or neonatal outcomes compared with standard FHR monitoring.
Assessment of fetal wellbeing using ST-segment waveform analysis from the fetal electrocardiogram has been proposed as an adjunct to conventional FHR monitoring. Westgate et al investigated the use of ST-segment analysis in animal models and demonstrated that although the T/QRS ratio indicated hypoxic stress, it correlated poorly with the severity of fetal hypoxia or hypotension. Several randomized controlled trials and meta-analyses have produced conflicting results, with the most recent randomized controlled trial not demonstrating any improvement in either neonatal outcomes or reduction in operative birth rates. A recent observational study showed that the implementation of intrapartum ST-segment waveform resulted in a substantial reduction in rates of umbilical artery metabolic acidosis as well as a significant reduction in operative birth rates, fetal blood sampling, and incidence of neonatal encephalopathy.
Fetal pulse oximetry (FPO) was developed as an adjunct to electronic FHR monitoring, particularly in the context of a nonreassuring FHR pattern. Fetal PO 2 saturations less than 30% are considered abnormal and corelate with hypoxic injury. In May 2000, the Food and Drug Administration granted approval for use of FPO as an adjunct to FHR monitoring based on a single randomized controlled trial that showed a significant reduction in cesarean section (CS) for IFC. However in 2006, a large, multicenter randomized controlled trial demonstrated that FPO as an adjunct to FHR monitoring did not reduce the rate of CS for any indication. An updated Cochrane review in 2013 of 7 published trials demonstrated that although FPO did not impact overall CS rates compared with FHR monitoring alone, there was a reduction in CS for IFC.
Intrapartum assessment of fetal scalp pH or lactate has not resulted in a decline in operative birth rates for IFC, neonatal acidosis, or adverse neonatal outcomes compared with the use of FHR monitoring alone.
Conclusion
Although the etiology of IFC is multifactorial, placental dysfunction and a mismatch between its functional ability and the metabolic requirements of fetus during labor is causative in the majority of cases. Labor is characterized by repeated transient episodes of fetal hypoxia that, under normal circumstances, an appropriately grown fetus with normal or minimal placental dysfunction is able to tolerate. However, when there is pre-existing placental dysfunction, inadequate uterine relaxation between contractions, or if the fetus is growth restricted with suboptimal metabolic reserves, intrapartum fetal hypoxia can ensue. In extreme cases, this can result in myocardial injury, systemic hypotension, cerebral hypoperfusion, and neuronal damage. Neonatal encephalopathy can have catastrophic consequences, with 1 in 2 infants either having significant neurodevelopmental delay, cerebral palsy, or death by the age of 2.
The challenges in this field are first, the development of a reliable pre-labor test that accurately identifies fetuses at risk of intrapartum compromise, second to develop new or refine current intrapartum techniques for fetal monitoring, and finally a prenatal or intrapartum treatment that can either prevent the development of intrapartum hypoxia or mitigate some of its perinatal complications. Accompanying these should be safe intrapartum care provided by trained caregivers.
J.T. and S.K. acknowledge research support by the Mater Foundation.
References
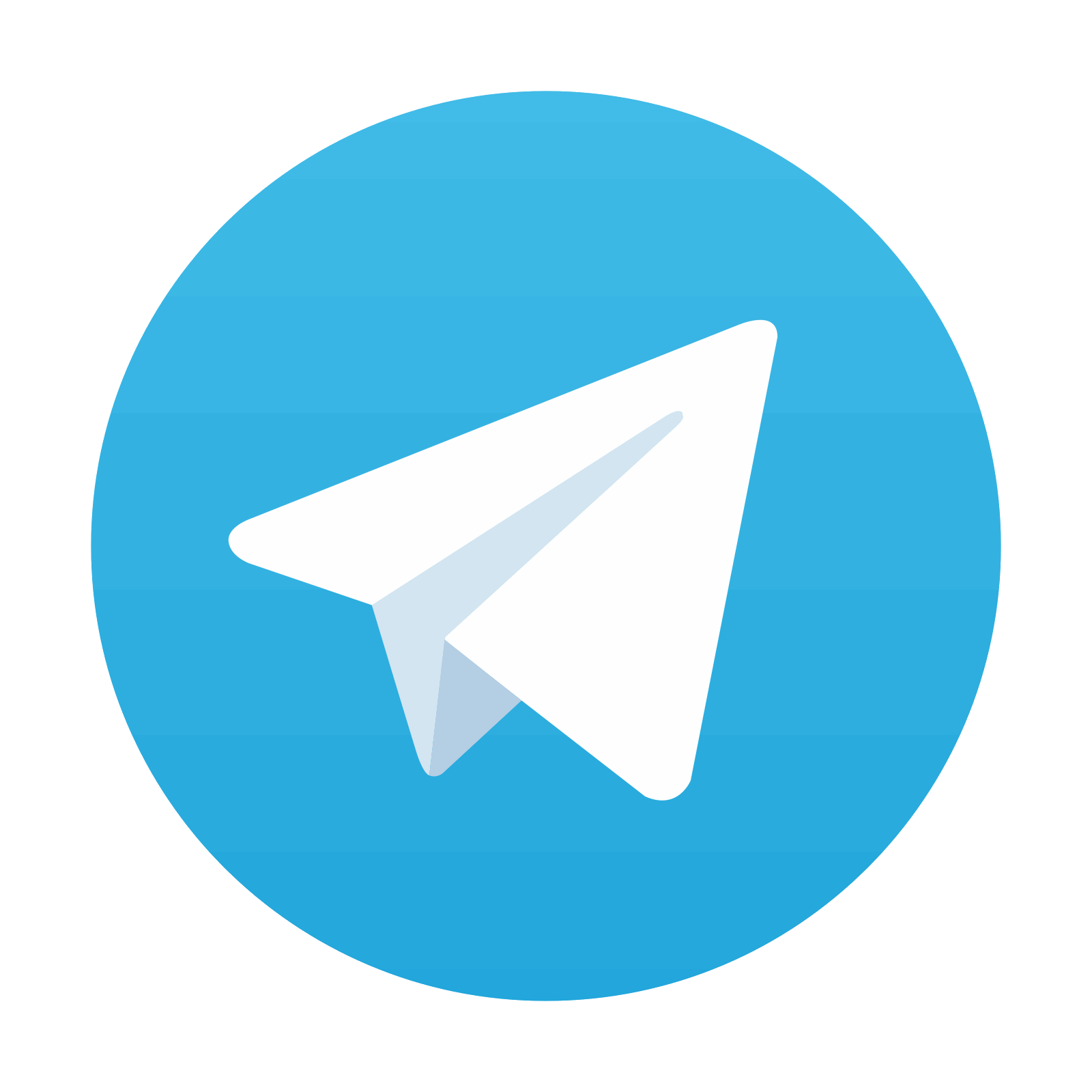
Stay updated, free articles. Join our Telegram channel

Full access? Get Clinical Tree
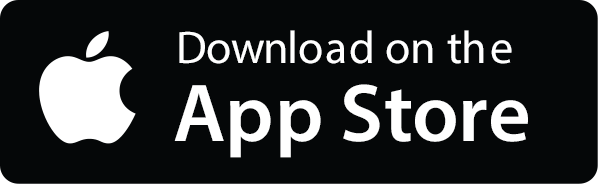
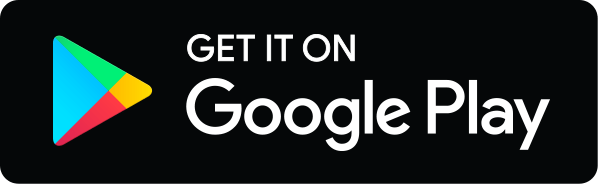
