The Function, Phylogeny and Ontogeny of Sleep
Function of Sleep
The first question asked of a biological function is an explanation of its purpose, yet the exact function of sleep still remains elusive today. Historically, physicians have, seemingly wisely, recommended sleep for the treatment of many ailments. This inexpensive prescription has been based on the assumption that sleep must have a unique restorative purpose. However, no study documents that sleep does cure anything.1 Circadian rhythms of various biological processes, e.g., the immune system, appear to be modulated by sleep: lymphocyte functions are dramatically altered at sleep onset and during sleep.2 Specific pokeweek mitogen response and natural killer cell activity are altered with sleep in healthy young men. Interlukin-1-like activities are followed by interlukin-2-like activities during sleep and interlukins-1 and -2 are disrupted with sleep deprivation.3 Narcoleptic patients present disordered diurnal patterns of immune function.4 However, what clinical effect these changes produce or how they may be therapeutically modified is unknown. The relationship between the immune system and sleep is obviously important, attractive, and possesses many clinical implications.
Restoration Theory
Sherington suggested in 1946 that sleep was a state required for enhanced tissue growth and repair.5 This theory holds that certain somatic and/or cerebral deficits occur as a result of wakefulness and sleep either allows or promotes physiological processes to repair or restore these deficits. This will, in turn, allow normal daytime functioning.6–8 Special focus has been placed on both restoration of somatic function and the central nervous system function. NREM sleep is thought to function in reparation of body tissue and REM sleep in restoration of brain tissue. Supporting evidence, however, is empirical and indirect. The theoretical role of NREM sleep in repair of somatic tissue comes from investigations that have shown the following:
1. Slow-wave sleep (SWS) increases following sleep deprivation.9
2. The percentage of SWS is increased during developmental years.10
3. Total sleep duration increases with body mass.11
4. Release of growth hormone occurs at sleep onset and peak levels occur during SWS in prepubertal children.12
5. The release of many endogenous anabolic steroids occurs in relation to a sleep-dependent cycle (prolactin, testosterone, and luteinizing hormone).13,14
6. The nadir of catabolic steroid release, such as corticosteroids, occurs during the first hours of sleep, coincident with the largest percentage of SWS.15
7. Increased mitosis of lymphocytes and increased rate of bone growth occur during sleep.16
8. There is a gradual increase of SWS percentage of total sleep time in response to a graded increase of physical exercise.17
However, contrary and conflicting observations exist. For example, while peak rates of cell division occur during sleep, it appears not to be due to sleep itself. Increased mitosis is demonstrable after a night without sleep, is positively influenced by oral glucose load, and negatively influenced by cortisol secretion.18 Similarly, in adolescents and adults, somatomedin levels are highest during waking, but not during sleep, as it is in prepubertal children.19
REM sleep, on the other hand, has been thought to function in restoration of central nervous system function. This state is characterized by intense CNS activation. REM sleep may have evolved in order to ‘reprogram’ innate behaviors and to incorporate learned behaviors and knowledge acquired during wakefulness.20 Synthesis of CNS proteins is increased during REM sleep.21 REM sleep also appears in significantly higher proportions in the fetus and newborn, gradually decreasing over the first few years of life. Increased protein synthesis during this sleep state may be critical in the development of the central nervous system.
Evolutionary and Adaptive Theories
Development of many physiological functions follows an orderly progression which mirrors phylogenetic development. It has been suggested that the development of sleep in the human organism also follows this same phylogenetic pattern. Evidence for this theory is scant. Animals sleep in many different ways, often more influenced by the environment and life-style than by evolution of the species.22 SWS and REM sleep rebound are characteristic features seen after sleep deprivation in the dog, cat, rabbit, and human.23 Definitive REM sleep, however, has never been documented in the dolphin. Dolphins do not have a pulmonary reflex to hypoxemia and have, therefore, complete voluntary control of breathing, and presumably sleep would be associated with impaired respiratory neural control. Actually, dolphins appear to exhibit hemispheric sleep. That is to say that when dolphins appear to sleep, slow-wave patterns are seen over a single hemisphere at a time, while the other hemisphere shows waking rhythm.24 If the evolutionary theory is true, animals with highly complex central nervous system function, such as the dolphin, should follow this pattern. It stands to reason that if the dolphin sleeps in the same manner as the dog, cat, and human, survival in its aquatic environment would be impossible. Skeletal muscle atonia during REM sleep (as currently understood) would result in drowning. Therefore, the life-style and environment of the dolphin play a much more significant role in the pattern of sleep development in these species than does phylogeny.
In some species, sleep may function to enhance survival. Animals that graze for food tend to sleep in bursts over a short period of time, a behavior which may provide time needed for sufficient food-seeking while protecting the animal from predators.25 Carnivorous animals that do not require large amounts of time for foraging and who are relatively safe from predation tend to sleep for long periods of time.
Sleep may also be an instinctive behavior, a patterned response to stimuli which conserves energy, prevents maladaptive behaviors and promotes survival.26 According to the evolutionary theory of sleep, REM sleep cortical activation may perform additional survival functions.27
Energy Conservation Theory
Sleep may function to conserve energy. Mammal species exhibit a high correlation between metabolic rate and total sleep time.28 This view states that energy reduction is greater during sleep than during periods of quiet wakefulness and sleep provides periods of enforced rest, barring the animal from activity for extended periods of time. Endothermic animals exhibit slow-wave sleep. During NREM sleep, endogenous thermoregulation continues, though functioning at levels below that of wakefulness. Poikilothermic species, on the other hand, do not exhibit clear SWS patterns. It is doubtful, however, that this theory explains the function of sleep in humans. Reduction in metabolism, which occurs during sleep, is minimal. Though the hypothesis is intriguing, energy conservation theory has been disputed and evidence exists that increase in sleep time does not correlate with increased metabolic rate.29,30 It has been shown that there is only approximately an 8% to 10% reduction in metabolic rate during sleep when compared with relaxed wakefulness. This would be insignificant when considering an adult human’s basal metabolic expenditure.
Learning Theory
A particularly interesting theory of the function of sleep centers on the role of sleep in the process of learning and memory. A significant body of knowledge exists which suggests that retention of new information depends on activation of some brain function which occurs at a critical period after the registration of this information.31,32 Two pivotal phases appear to exist. The first is one of ‘consolidation.’ Medication which causes stimulation of the reticular activating system and cortical excitation during the first 90 seconds after acquisition of new information appears to enhance memory and increase retention. Though the consolidation phase of learning is important, it cannot be considered definitive for fixation of information since processing continues for a long period of time.
Over the past 50 years, beneficial effects of sleep on the retention of memories acquired during wakefulness have been documented.33,34 REM sleep appears to hold special significance. Despite evidence from animal and human studies, the exact function of REM sleep in childhood development and learning remains unknown. Diverse reasons have been proposed for children’s learning difficulties, but no single factor appears to be consistent for all individuals. Most diagnostic and treatment protocols have empirically focused on the child’s daytime capabilities.
Minor neurologic and electroencephalographic (EEG) abnormalities have been described in children with hyperactivity syndrome.35 These abnormalities have been associated with specific or global learning difficulties and the syndrome had previously been described as ‘minimal cerebral dysfunction.’ Neurologic and EEG abnormalities associated with this ‘hyperactivity’ syndrome, however, have been shown to be non-specific and variable,36 resulting in a change of the name of the syndrome to ‘attention deficit hyperactivity disorder.’
It is noteworthy that of 15 reading-disabled (dyslexic) children studied by Levinson, 97% revealed evidence of cerebellar–vestibular (CV) dysfunction. Ninety-six percent of 22 blinded neurological examinations and 90% of 70 completed electronystagmograms indicated similar CV dysfunction.37 Ottenbacher et al. explored the relationship between vestibular function as measured by duration of postrotatory nystagmus and human figure-drawing ability in 40 children labeled as learning-disabled.38 Chronological age and postrotatory nystagmus durations shared significant amounts of variance with human figure-drawing. The variables of IQ and sex were non-significant. DeQuiros and Schrager have also identified vestibular dysfunction in some learning-disabled children39 and described another related syndrome termed ‘vestibular–oculomotor split,’ which results in impaired ocular fixation, scanning ability, and poor eye–head coordination.
Despite the evidence that some children with learning disabilities display soft or non-focal neurologic signs40 and low scores on tests of visual–motor integration, reading achievement, and ocular scanning,41 contrary evidence of normal vestibular responses to rotation in dyslexic children has been published. Brown et al. measured eye movements provoked by sinusoidal rotation of the subjects at low frequencies.42 Gain, phase, and asymmetry of the responses were calculated from the eye velocity and stimulus velocity wave forms. There were no differences between the groups in any of the measurements. These results led to the conclusion that ‘there are no clinically measurable differences in this aspect of vestibular function’ in their carefully selected population of dyslexic and control children. Their conclusions, however, were based on evidence obtained during the waking state. Vestibular nuclei play a major role in the control of eye movements when awake and asleep. If these nuclei are destroyed, eye movements during REM sleep are absent. In a pilot study of four reading-disabled children, a significant difference was found in the mean angular velocity of eye movements during REM sleep when compared with three normally reading controls.43
Correlates exist which associate the phasic events of rapid eye movements with CV control. Pompeiano et al. have shown that lesions of the medial and descending vestibular nuclei in the cat eliminated all phasic inhibition of sensory input, spinal reflexes, and all motor output associated with phasic REM bursts, including eye movements themselves.44,45 They have also demonstrated that intense spontaneous discharges from neurons of the vestibular nuclei occur synchronous with the ocular activity of REM sleep. Nystagmus evoked by rotation can be most readily induced during sleep at the time of phasic events of REM sleep46 and in Wernicke–Korsakoff’s disease, where the vestibular nuclei are often damaged, eye movements are absent during REM sleep.47 These observations partially confirm the influences of vestibular mechanisms of the phasic activity of REM sleep.
An age-related development of phasic inhibition of auditory evoked potentials during the ocular activity of REM sleep, and an age-related increase in the duration of the REM burst, have been described in normal subjects.48 It seems that central vestibular influences underlie these events, and that vestibular control of phasic activity follows a developmental maturation schedule.
Significant literature exists, however, supporting a relationship between REM sleep, phasic REM activity, and learning. Sleep patterns in hyperkinetic children and normal children were studied by Busby, Firestone and Pivik.49 Analysis of sleep pattern variables revealed a significantly longer REM onset latency and a greater absolute and relative amount of movement time for the hyperkinetic group relative to controls. No other sleep parameter differentiated the groups. Clinical observations of autistic children have suggested that fundamental symptoms of the syndrome of childhood autism involve disturbances of motility and perception. The nature of these disturbances indicates a maturational delay in the development of complex motor patterns and the modulation of sensory input.48 Sleep studies have provided some evidence for a maturational delay in the differentiation of REM sleep patterns and the development of phasic excitatory and inhibitory mechanisms during REM sleep in these children. These findings implicate a failure of central vestibular control over sensory transmission and motor output during REM sleep. The notation that there is a dysfunction of central vestibular mechanisms underlying the delayed organization and differentiation of the REM sleep state is supported by observations of altered vestibular nystagmus in the waking state in autistic children.48
Studies in animals and humans support the importance of REM sleep in learning. Lucero conducted experiments which showed a significant increase in REM sleep duration with respect to controls, a non-significant increase in total sleep time, and no changes in slow-wave sleep duration in animals subjected to consecutive learning experiences.50 Increase in REM sleep time observed after incremented learning suggests that REM sleep might be involved in the processing of information acquired during wakefulness. It has been postulated that such processing might consist of the transformation of a ‘labile program’ acquired in the learning session into a more ‘stable program’ devoid of superfluous information.
Major evidence of the importance of REM sleep in facilitating recall of complex associative information has been documented by Scrima.51 The beneficial effect of isolated REM and isolated NREM sleep on recall was tested in 10 narcoleptic subjects. The results for complex associative tasks indicated significant differences between three conditions for free recall. Recall was significantly better after isolated REM than after isolated NREM sleep or wakefulness and was significantly better after NREM sleep than after wakefulness. It was concluded that the results were consistent with the proposed neuronal activity correlates theory of Emmons and Simon52 that REM sleep actively consolidates and/or integrates complex associative information and that NREM sleep passively prevents retroactive interference of recently acquired complex associative information.
Newborn animals and human infants show a greater proportion of REM sleep with respect to total sleep time than adults,53,54 and a progressive decrease in that proportion as growing continues, which is paralleled by a decrease in learning ability.24 Fishbein has shown that REM sleep deprivation, both prior to and following learning, disrupts primarily long-term memory processes.55 Evidence has been provided that learning induces a protracted augmentation of paradoxical sleep time, lasting for at least 24 hours.56 This work, together with previous works, suggest that REM sleep augmentation may be a neurobiological expression of the long-term process of memory consolidation. Fishbein was able to augment REM sleep using behavioral techniques of learning. Therefore, one psychobiological function of REM sleep may be to process and maintain information during wakefulness.
Results obtained from non-deprivation studies of animals provide consistent support for the hypothesis that REM sleep is functionally related to learning. Results of studies that have employed multiple training sessions may be interpreted to suggest either that prior REM sleep prepares the organism for subsequent learning, or that REM sleep facilitates consolidation and retrieval of prior learning. Given the equivocality of prior REM deprivation literature, the second interpretation seems more reasonable.57
Impaired cognitive functioning has been documented in studies conducted on sleep-deprived physicians. In one investigation, cognitive functioning in acutely and chronically sleep-deprived house officers was evaluated.58 Analysis of data revealed significant deficits in primary mental tasks involving basic rote memory, language and numeric skills, as well as in tasks requiring high-order cognitive functioning and intellectual abilities.
Acquisition of many simple learning tasks in animals is followed by augmentation of REM sleep without any modification of NREM sleep.31 Augmentation of REM sleep after learning has also been described in human infants.59 Sleep may be particularly important for RNA and DNA synthesis linked to memory processes. There is some evidence that during sleep RNA is more actively synthesized, less rapidly degraded, or more slowly transported into the cytoplasm.60
As in infants, there is some evidence that REM sleep may increase following learning in older children and adults. Hartman has demonstrated an increase of REM sleep time occurring after days of increased learning, mental stress, and especially demanding events.61 If learning does cause an increase in REM sleep, brain-damaged patients who are improving should have a higher proportion of REM sleep than patients who show no improvement. Following up a group of nine patients with severe traumatic brain damage, Ron et al. found a correlation between cognition and REM sleep improvement in seven patients.62 Greenberg and Dewan compared the percentage of REM sleep in improving and non-improving aphasic patients and found that the latter groups did, in fact, have lower levels.63 In 32 patients with Down syndrome, phenylketonuria, and other forms of brain damage, Feinberg found a positive relation between the amount of eye movement during REM sleep and estimates of intellectual function,10 while in a comparison of 38 normal individuals and 15 brain-damaged subjects it was shown that mentally retarded patients had less REM sleep.64 Linkage between REM sleep and development of the visual system has been further supported by a recent study by Oksenberg and co-workers.65 They have reported significant anatomical changes in the microscopic anatomy of the visual cortex in REM sleep-deprived cats.
Unlearning Theory
An antithetical hypothesis for the function of REM sleep in learning and memory involves a process of unlearning. No single memory center appears to exist in the brain.66 Many parts of the central nervous system participate in the representation of a single event. However, localization of memory of a single event generally involves a limited number of neural pathways, and those collections of neurons within which a memory is equivalently represented probably contains a ‘set’ of no more than a thousand neurons. These interconnected assemblies of cells could store associations.67,68 If the cells involved in the ‘memory’ of an event form mutual synapses, when part of that event is encountered again, regeneration of the activity of the entire neuronal set would occur. Crick and Mitchison proposed that the function of REM sleep, therefore, is to remove certain undesirable modes of interaction in networks of cells in the cerebral cortex.69 This would be accomplished during REM sleep by a ‘reverse learning’ mechanism, so that the trace in the brain of the unconscious dream is weakened, rather than strengthened, by the activity of dreaming. A mathematical and computer model of a network of 30 to 1000 neurons has been developed by Hopfield, Fenistein, and Palmer.70 Their model network has a content-addressable memory or ‘associative memory’ which allows it to learn and store many memories. A particular memory can be evoked in its entirety when the network is stimulated by an adequately sized subpart of the information of that memory. When memories are learned, spurious information is created and can also be evoked. Applying an ‘unlearning’ process, similar to the learning process, but with a reversed sign and starting from a noise input, enhanced the performance of the network in accessing real memories and minimizing spurious ones.
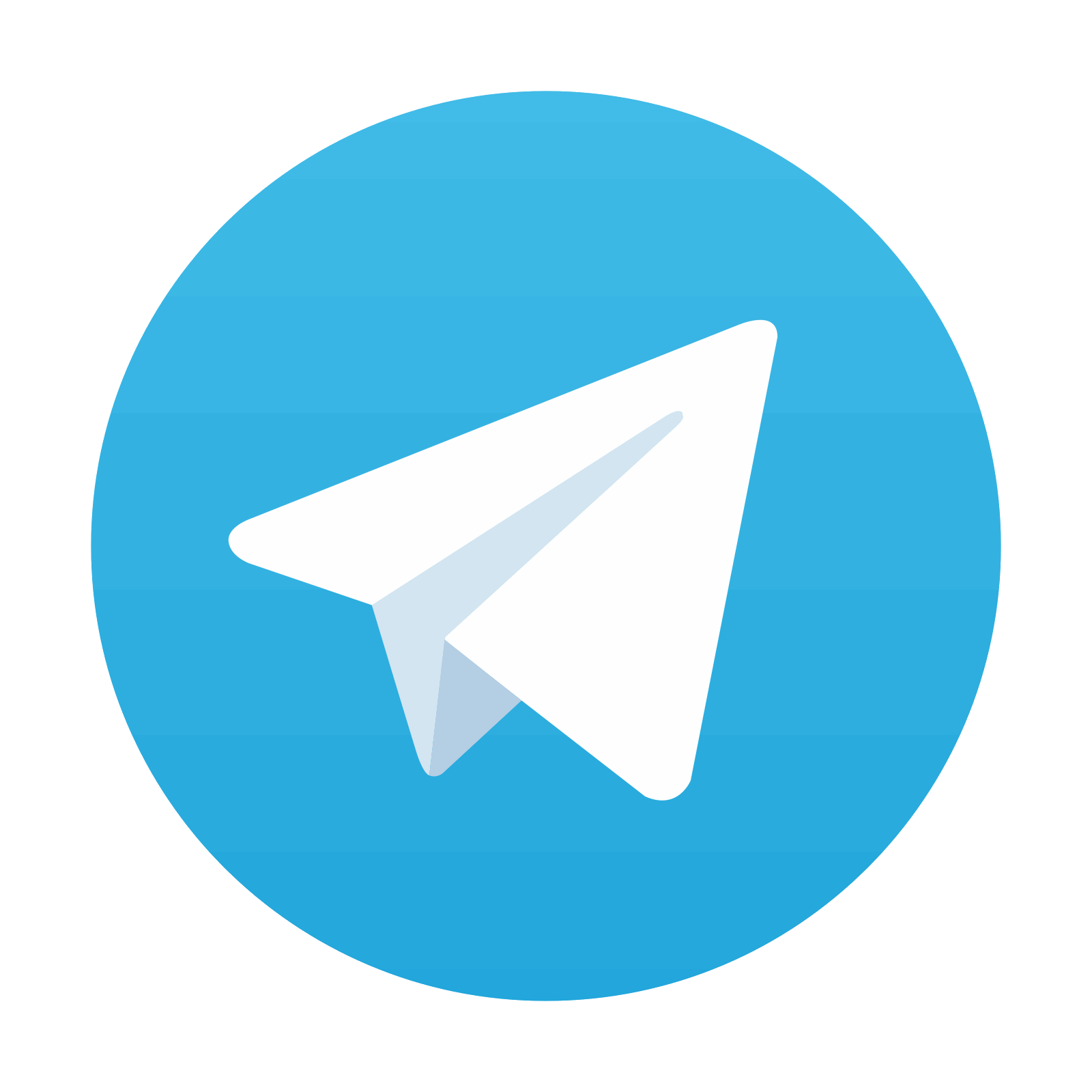
Stay updated, free articles. Join our Telegram channel

Full access? Get Clinical Tree
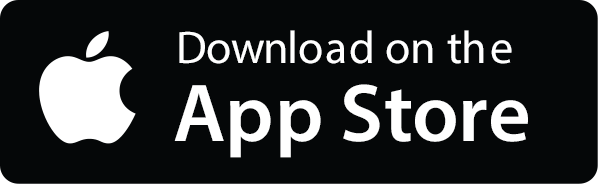
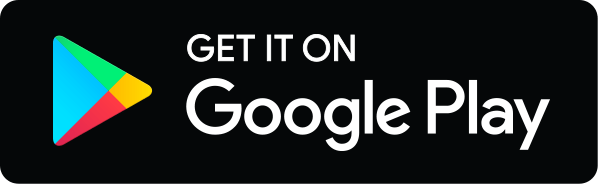