Zika virus is an emerging mosquito-borne ( Aedes genus) arbovirus of the Flaviviridae family. Following epidemics in Micronesia and French Polynesia during the past decade, more recent Zika virus infection outbreaks were first reported in South America as early as May 2013 and spread to now 50 countries throughout the Americas. Although no other flavivirus has previously been known to cause major fetal malformations following perinatal infection, reports of a causal link between Zika virus and microcephaly, brain and ocular malformations, and fetal loss emerged from hard-hit regions of Brazil by October 2015. Among the minority of infected women with symptoms, clinical manifestations of Zika virus infection may include fever, headache, arthralgia, myalgia, and maculopapular rash; however, only 1 of every 4–5 people who are infected have any symptoms. Thus, clinical symptom reporting is an ineffective screening tool for the relative risk assessment of Zika virus infection in the majority of patients. As previously occurred with other largely asymptomatic viral infections posing perinatal transmission risk (such as HIV or cytomegalovirus), we must develop and implement rapid, sensitive, and specific screening and diagnostic testing for both viral detection and estimation of timing of exposure. Unfortunately, despite an unprecedented surge in attempts to rapidly advance perinatal clinical testing for a previously obscure arbovirus, there are several ongoing hindrances to molecular- and sonographic-based screening and diagnosis of congenital Zika virus infection. These include the following: (1) difficulty in estimating the timing of exposure for women living in endemic areas and thus limited interpretability of immunoglobulin M serologies; (2) cross-reaction of immunoglobulin serologies with other endemic flaviruses, such as dengue; (3) persistent viremia and viruria in pregnancy weeks to months after primary exposure; and (4) fetal brain malformations and anomalies preceding the sonographic detection of microcephaly. In this commentary, we discuss screening and diagnostic considerations that are grounded not only in the realities of current obstetrical practice in a largely global population but also in basic immunology and virology. We review recent epidemiological data pertaining to the risk of congenital Zika virus malformations based on trimester of exposure and consider side by side with emerging data demonstrating replication of Zika virus in placental and fetal tissue throughout gestation. We discuss limitations to ultrasound based strategies that rely largely or solely on the detection of microcephaly and provide alternative neurosonographic approaches for the detection of malformations that may precede or occur independent of a small head circumference. This expert review provides information that is of value for the following: (1) obstetrician, maternal-fetal medicine specialist, midwife, patient, and family in cases of suspected Zika virus infection; (2) review of the methodology for laboratory testing to explore the presence of the virus and the immune response; (3) ultrasound-based assessment of the fetus suspected to be exposed to Zika virus with particular emphasis on the central nervous system; and (4) identification of areas ready for development.
Click Supplemental Materials under article title in Contents at ajog.org
Human infection with Zika virus (ZIKV), an emerging mosquito-borne flavivirus ( Flaviviridae family, Flavivirus genus), has reached pandemic levels in the Americas, with at least 50 countries or territories, including Puerto Rico, Florida, and Texas, reporting infection over the interval from May 2015 through November 2016 ( Figure 1 ). The recently confirmed causal link between ZIKV and fetal microcephaly now places ZIKV among the relatively small list of infections in pregnant women that lead to congenital anomalies. Coupled with the recent pandemic, this has led to unprecedented numbers of pregnant women at risk for having fetuses with severe abnormalities.
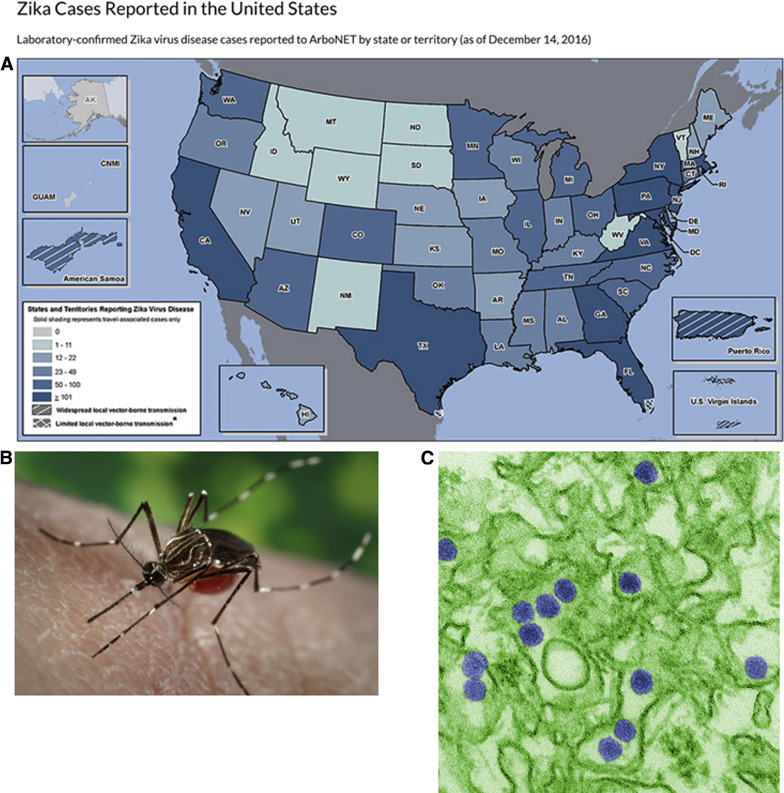
Epidemiology and estimates of congenital ZIKV infection
Previous outbreaks of ZIKV were largely sporadic across Southeast Asia and equatorial African belts but later spread east, resulting in an outbreak in Yap Island in 2007, followed by epidemics in French Polynesia, New Caledonia, the Cook Islands, and Easter Island in 2013 and 2014.
Until recently ZIKV illness was thought to be self-limiting, resembling a mild version of dengue virus (DENV) or chikungunya virus. ZIKV is transmitted via Aedes spp of mosquitos and is spread via sexual transmission and vertical (mother to child) and blood transfusions ( Figure 1 ). Clinical manifestations of ZIKV infection include fever, headache, arthralgia, myalgia, and maculopapular rash, although only 1 of every 4–5 people who are infected manifest symptoms. Thus, clinical symptoms are not an effective screening tool for the diagnosis or relative risk of ZIKV infection because approximately 80% are likely asymptomatic.
Based on the initial reports of relatively mild symptoms accompanying infection, ZIKV was not thought to lead to severe consequences. Exceptions included rare cases of Guillian-Barre (73 of 28,000 cases) and even rarer instances of perinatal transmission (2 initial cases, with potentially as great as 1% by later estimates) first reported during the French Polynesia outbreak. However, as ZIKV spread to the Americas in far higher volume (1.3 million autochthonous cases by December 2015), an approximate 20-fold increase in congenital cases of microcephaly with brain and ocular malformations was reported throughout northeast and southeast Brazil. Although no other flavivirus is known to cause disseminated fetal neural malformations in humans, worldwide concern for latent viral disease was raised following several case reports demonstrating ZIKV RNA in the amniotic fluid, placenta, and fetal neural tissue weeks to months after the initial maternal infection ( Figure 2 ).
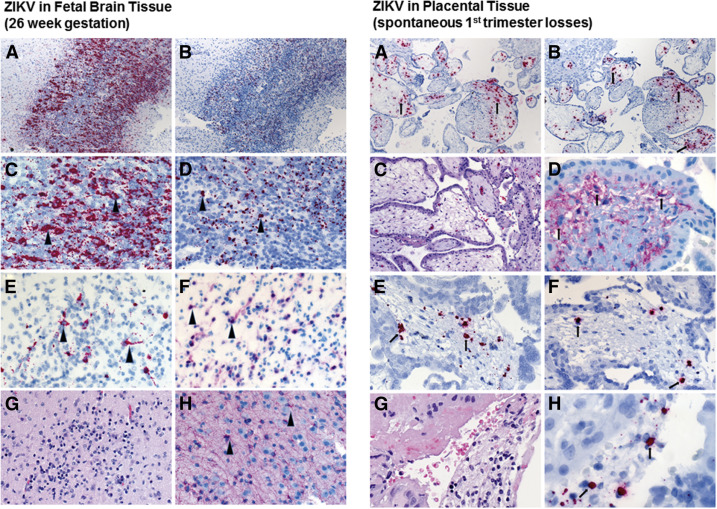
More recently, however, several national and regional cohorts or registries have provided further evidence, suggesting that there are multiple clinical manifestations of ZIKV infection in pregnancy. The first of these studies was published as a preliminary description of a prospective cohort of 88 symptomatic gravidae from Rio de Janeiro, Brazil, that had been followed up throughout gestation, with a further expanded description published in late December 2016 and inclusive of 134 ZIKV positive women.
In the preliminary report, Brasil et al described that 42 of the 72 symptomatic gravidae who tested positive for ZIKV underwent ultrasound examination, with 29% (12 of 42 ZIKV) demonstrating variable findings on ultrasound, ranging in presumptive severity from central nervous system (CNS) lesions with microcephaly to isolated findings suggestive of placental insufficiency such as fetal growth restriction or abnormal umbilical artery Doppler velocimetry or amniotic fluid volume.
Fortunately, Brasil et al published an expanded recent follow-up from this prospective cohort with detailed pregnancy and infant outcomes. Inclusion into this expanded study cohort of 345 women was limited to those presenting to a single clinic in Rio de Janeiro with a rash, and all positive ZIKV cases were defined by testing positive within 5 days of rash development for ZIKV viral nucleic acid by polymerase chain reaction (PCR; QuantiTect Probe real-time reverse transcriptase polymerase chain reaction [rRT-PCR] ) on blood specimens, urine specimens, or both. Of the 345 gravidae with a rash enrolled, 182 were PCR positive for ZIKV and 163 were PCR negative. Of the 182 ZIKV-positive, 125 of 134 had delivery and follow-up data; of the 163 ZIKV negative, 61 were followed up through delivery.
Because only gravidae presenting to the clinic with a rash were enrolled in this cohort study, it is not surprising that ZIKV-negative women were more likely to have positive Chikungunya virus immunoglobulin (Ig) M or PCR results (41.7%, or 25 of 60, vs 2.8%, or 3 of 106 tested, P < .001). Interestingly, more than half of ZIKV-positive women presented with acute infection in the second trimester, and ZIKV-negative women were more likely to have used insect repellent (80% vs 60%, P = .0006).
They reported adverse pregnancy outcomes in 46.4% (58 of 125 after 9 of the 134 gravidae were lost to follow-up), including a 7% risk of fetal loss (9 of 125; 6 of 9 miscarriages and 3 of 9 stillbirths) and 41.9% (49 of 117 live births) with congenital anomalies noted by the first month of life (49 of 117 ). The rate of adverse pregnancy outcomes including fetal loss with laboratory-documented ZIKV infection was similar and did not significantly vary by trimester of exposure (55%, or 11 of 20 of pregnancies in the first trimester, 52%, or 37 of 72 in the second trimester, and in 29%, or 10 of 34 of those in third trimester ).
In a comparative analysis with the ZIKV-negative pregnancies, a statistically significant difference was observed for the elevated risk of adverse pregnancy outcomes in any trimester with ZIKV-documented infection (46.4% vs 11.5%, P < .001), emergency cesarean delivery (23.5% vs 2.5%, P = .003), and evidence of abnormal neonatal and infant findings (41.9% vs 5.3%, P < .001 ).
Interestingly, Brasil et al described that almost all of the neonatal and infant abnormalities detected in ZIKV-positive gravidae affected the CNS but were not accompanied by microcephaly per se. Positive postnatal findings included microcephaly, cerebral calcifications, cerebral atrophy, ventricular enlargement, hypoplasia of cerebral structures, parenchymal brain hemorrhages, and gross findings on postnatal examination. In fact, among ZIKV-positive gravidae, a total of 31 of 49 (63%) infants available for follow-up displayed 1 or more of the following: hypertonus, spasticity, limb contractures, seizures, persistent cortical thumb sign or clenched fists, redundant scalp skin (even among normocephalic infants), and abnormal funduscopic or audiology examinations.
Of note, although the data provided are descriptive, the authors found that a number of infants with normal clinical assessments in early infancy had abnormal nonspecific postnatal magnetic resonance imaging (MRI) findings. These included diffuse T2 hypersignaling in in the peritrigonal posterior areas and less evident in the frontal parietal white matter, with diffusion sequence hyposignaling. These findings are abnormal in infants and raise concern for cortical tract dysfunction.
Although the reports of Brasil et al are limited to symptomatic women from a single pregnancy clinic in Rio de Janeiro, a similar spectrum of congenital findings have been described among women exposed in other regions of the Americas. Honein et al have provided recent estimates from a US Centers for Disease Control and Prevention (CDC) and health department registry (US Zika Pregnancy Registry) of 442 completed and registered pregnancies.
These US-based investigators reported that 6% (26 of 442, 95% confidence interval [CI], 4–8%) of maternal ZIKV infections result in congenital birth defects and reach 11% (9 of 85, 95% CI, 6–19%) when exposure was documented exclusively in the first trimester or preconception; no cases of microcephaly or brain malformations were detected unless first-trimester exposure was documented to occur. However, gestational age at infections was unknown for 2 of 26 fetuses or infants with birth defects and 27 of the 442 total completed pregnancies; nearly half of all women in the US. Zika registry had exposure during multiple trimesters of pregnancy.
Of the 26 affected fetuses or infants, 4 had microcephaly but no reported neuroimaging, 14 had microcephaly and brain abnormalities, and 4 had brain abnormalities without microcephaly. Malformations noted included intracranial calcifications, abnormalities of the corpus callosum and cortical formation, cerebral atrophy, ventriculomegaly, hydrocephaly, and cerebellar abnormalities.
The rate of congenital ZIKV among reported pregnancies did not differ by symptom occurrence or severity (6% in both groups), and asymptomatic registry subjects were just as likely to have an affected infant or fetus as symptomatic subjects (16 of 271 symptomatic, 95% CI, 4–9%, vs 10 of 167, 95% CI, 3–11% ). Interestingly, of the 442 women, 61% (271 of 442) were asymptomatic and 38% (167 of 442) were symptomatic.
There are several comments pertaining to the US registry report of Honein et al that are worth considering prior to implementing their findings as part of obstetrical clinical counseling or risk assessment. First, registered subjects represented exposure resulting from travel of the subject or their partner to endemic regions in addition to only Brazil and include Barbados, Belize, Colombia, the Dominican Republic, El Salvador, Guatemala, Haiti, Honduras, Mexico, Marshall Islands, and Venezuela.
Second, registry inclusion necessitated laboratory evidence of possible ZIKV infection and required either physician or public health reporting of positive cases. As with any voluntary registry, there is a strong risk of ascertainment bias. Both performance of testing for exposure to ZIKV and entry into the registry may be biased by either symptoms or ultrasound-based detection of anomalies. Similarly, a positive ZIKV test may have precluded additional genomic testing, including chromosomal microarray and karyotypic analysis.
In addition, access to and availability of testing likely limited the population denominator in this study, and the human factors related to reporting may have overrepresented the numerator. This possibility is best realized by the reported rate of 61% of registry subjects reporting symptoms, which is 2–3 times the anticipated rate.
Finally, although no birth defects were reported among the pregnancies with maternal symptoms or exposure only in the second trimester (0 of 76) or third trimester (0 of 31), ongoing follow-up of infants was not reported, and there was insufficient data to adequately estimate the purported affected during the latter 2 trimesters. Because there are multiple reports of normocephalic neonates at birth after second- and third-trimester ZIKV exposure who subsequently display postnatal brain malformations, case affect rates arising solely from the current registry ought to be considered preliminary estimates.
In summary of both the Brazilian symptomatic cohort of Brasil et al and the US-based registry of Honein et al, tremendous gratitude should be extended to these investigative teams for their Herculean efforts aimed at collecting, characterizing, and detailing broad and robust perinatal clinical outcomes in the year since the association between ZIKV and fetal malformations was first largely recognized.
However, these high-impact reports are limited by hindrances inherent to descriptive cohorts and estimates of relative or absolute risk of congenital ZIKV malformations in any trimester of pregnancy remain preliminary. All counseling should be accordingly framed with an appropriate degrees of uncertainty. This would include acknowledging a persistent but not currently quantifiable attributable risk for congenital ZIKV malformations following infection at any point during gestation and a need for postnatal follow-up. Moreover, until population-based studies are completed with universal testing of both asymptomatic and symptomatic women at risk of exposure, the true attributable risk estimate cannot be determined with any degree of confidence.
Given the challenges in estimating the likelihood of congenital malformation following perinatal ZIKV exposure, we are left to question to what extent the current ZIKV pandemic will affect the global health burden in the coming decades. In the absence of ZIKV exposure in the population, microcephaly occurs in approximately 7 per 10,000 births. Assuming an estimated attack rate of ZIKV as high as 73% (as occurred on the island of Yap ), the burden to both individual families and society in the face of a burgeoning pandemic is potentially staggering, even if the risk of microcephaly or congenital brain malformation is as low as 0.1–11% among symptomatic gravidae. In light of such a potential burden and the need for accurate risk estimates, the importance of accurate and predictive diagnosis is readily evident.
Who should be tested and how?
The first step in potentially diagnosing congenital ZIKV infection is the recognition of who should be tested. Because the majority of infected patients will have no or mild symptoms, clinical screening is not a reliable tool. There are 2 key risk factors that make pregnant women eligible for testing: those either with a personal history of exposure risk (either traveling to or residing in an endemic area) or sexual contact with a partner bearing the same exposure risk. Recognition of those at risk for ZIKV is therefore predicated on screening patients for regional travel and residence and sexual history, 2 areas commonly neglected in standard medical visits. Use of prompts within the electronic medical record may aid in screening.
For those women who have resided in or traveled to a Zika endemic area, the CDC recommends testing inclusive of 8 weeks prior to conception and throughout gestation. This recommendation is based on a doubling of the maximal incubation time in nonpregnant women (3–14 days) plus intervals of viremia (2–10 days ) and viruria (up to 14 days). These rough estimations were empirically demonstrated in a nonpregnant subject case report from our group documenting viral shedding in the vaginal mucosa for 2 months after symptomatic infection.
In addition, women whose male sexual partners have traveled to areas with active ZIKV transmission have a risk of viral acquisition for 6 months. However, the exact duration of potential transmission via sexual exposure is currently unknown, although ZIKV RNA has been discovered in semen for up to 188 days after illness onset. Important pitfalls to these guidelines include the potential for prolonged viremia and viruria noted in pregnant women.
Who should be tested and how?
The first step in potentially diagnosing congenital ZIKV infection is the recognition of who should be tested. Because the majority of infected patients will have no or mild symptoms, clinical screening is not a reliable tool. There are 2 key risk factors that make pregnant women eligible for testing: those either with a personal history of exposure risk (either traveling to or residing in an endemic area) or sexual contact with a partner bearing the same exposure risk. Recognition of those at risk for ZIKV is therefore predicated on screening patients for regional travel and residence and sexual history, 2 areas commonly neglected in standard medical visits. Use of prompts within the electronic medical record may aid in screening.
For those women who have resided in or traveled to a Zika endemic area, the CDC recommends testing inclusive of 8 weeks prior to conception and throughout gestation. This recommendation is based on a doubling of the maximal incubation time in nonpregnant women (3–14 days) plus intervals of viremia (2–10 days ) and viruria (up to 14 days). These rough estimations were empirically demonstrated in a nonpregnant subject case report from our group documenting viral shedding in the vaginal mucosa for 2 months after symptomatic infection.
In addition, women whose male sexual partners have traveled to areas with active ZIKV transmission have a risk of viral acquisition for 6 months. However, the exact duration of potential transmission via sexual exposure is currently unknown, although ZIKV RNA has been discovered in semen for up to 188 days after illness onset. Important pitfalls to these guidelines include the potential for prolonged viremia and viruria noted in pregnant women.
Why should we test and not just screen for microcephaly with ultrasound? Molecular diagnostics for viral pathogens and estimation of risk
There are 2 primary reasons that screening for microcephaly is insufficient. First, there are multiple malformations that may not entail microcephaly, including intracranial calcifications, abnormalities of the corpus callosum, and cerebellar abnormalities. Second, in instances of abnormal cortical formation, cerebral atrophy, and cortical neuronal agenesis, neuronal death will occur over time and microcephaly will be observed with age.
Fortunately, over the past 2 decades, clinical laboratory medicine has witnessed an unprecedented capacity in the ability to rapidly test for infectious pathogens. It was not long ago when diagnosis relied on weeks of viral cultures, which were often low or nil yield, whereas today’s current clinical laboratory medicine and pathology practice allows for highly sensitive and specific rapid molecular diagnostic testing. Ergo, we are notably handicapped when we cannot reliably identify a potential viral pathogen or establish exposure and immunity with serological testing. In many circumstances diagnostic testing is utterly and fundamentally necessary to both personalized clinical management and public health control measures.
This is no truer than when a viral pathogen will result in a devastating perinatal transmission, yielding a potentially severely compromised infant faced with lifelong disability. In the short term, diagnoses are important for decisions of pregnancy continuation. However, in the longer term, accurate and early diagnosis becomes the cornerstone of developing targeted and efficacious interventions and estimating the natural history of an infection to give truly informed risk estimates.
Currently available laboratory-based testing for ZIKV
There are several limitations to currently available testing for ZIKV. To illustrate these limitations, consider the following hypothetical clinical scenario. Ms Jones is a 35 year old G2P0010 who resides in Texas but works part time in Brazil. Her husband resides and works full time in Brazil. She comes to see you at 16 weeks’ gestation, after she completed a 4 month interval working in Rio de Janeiro with frequent travel to the northern provinces. You have performed an ultrasound, which shows borderline bilateral ventriculomegaly at 11 and 12 mm, with an overall estimated fetal weight at the 24th centile and a head circumference and biparietal diameter at the 15th centile. Per the CDC algorithm, she should be eligible for IgM testing up to 12 weeks from entry back into the United States. However, what if she were actually infected at 4 weeks’ gestation? Her IgM might be negative or indeterminate as a result of natural titer waning. In the absence of an IgG isotype serological test, a negative or indeterminate IgM titer would potentially be interpreted falsely as negative.
Knowing these limitations, what are the testing options for this patient? Would rRT-PCR testing be helpful? When should each test be performed? How should we interpret our testing results, and what are the current limitations to these interpretations? In the following sections, we discuss testing options; Tables 1 and 2 provide summary guidance.
Test category | Specimen sources | Timing of first positive test a | Duration of positive test a | Limitations | Interpretations of positive tests | |
---|---|---|---|---|---|---|
Viral serology | IgM | Serum CSF | 4 d in symptomatic 7-14 d in asymptomatic Unknown | 12 wks Unknown |
|
|
IgG | Serum | 7-14 d b | >12 wks |
|
| |
PRNT | Serum | With positive serological testing |
|
See Table 2 | ||
Viral nucleic acid testing | rRT-PCR | Serum Blood Urine CSF Tissue Amniotic fluid | 0-7 d 0-7 d 0-14 d Unknown Unknown Unknown | 5-14 d c 5-14 d c 14 d c Unknown Unknown Unknown |
|
Recent Zika infection |
Ultrasound | Amniotic fluid Biometry Neurological Extremities | Variable | Once present, appears progressive |
|
When performed in isolation, cannot distinguish anomaly cause. When performed in conjunction with amniocentesis with chromosomal microarray or other infectious pathogen testing, improved specificity; see Table 3 . |
a Based on current information
b Extrapolated from West Nile IgG
c Prolonged viremia and viruria noted in pregnant women and neonates.
IgM ELISA testing | Result | Interpretation |
---|---|---|
ZIKV IgM | Detected | No evidence of Zika or Dengue infection, false-positive IgM |
ZIKV PRNT | Not detected | |
DENV PRNT | Not detected | |
ZIKV IgM | Positive or equivocal | Recent Zika virus infection |
DENV IgM | Positive or equivocal | |
ZIKV PRNT | ≥10 | |
DENV PRNT | <10 | |
ZIKV IgM | Positive or equivocal | Recent Dengue virus infection |
DENV IgM | Positive or equivocal | |
ZIKV PRNT | <10 | |
DENV PRNT | ≥10 | |
ZIKV IgM | Inconclusive in one assay and inconclusive or negative in the other | Recent flavivirus infection; specific virus cannot be determined |
DENV IgM | ||
ZIKV PRNT | ≥10 | |
DENV PRNT | ≥10 | |
ZIKV IgM | Inconclusive in one assay and inconclusive or negative in the other | Evidence of Zika virus; timing cannot be determined |
DENV IgM | ||
ZIKV PRNT | ≥10 | |
DENV PRNT | <10 | |
ZIKV IgM | Inconclusive in one assay and inconclusive or negative in the other | Evidence of Dengue virus; timing cannot be determined |
DENV IgM | ||
ZIKV PRNT | <10 | |
DENV PRNT | ≥10 | |
ZIKV IgM | Inconclusive in one assay and inconclusive or negative in the other | Evidence of flavivirus infection; specific virus and timing of infection cannot be determined |
DENV IgM | ||
ZIKV PRNT | ≥10 | |
DENV PRNT | ≥10 |
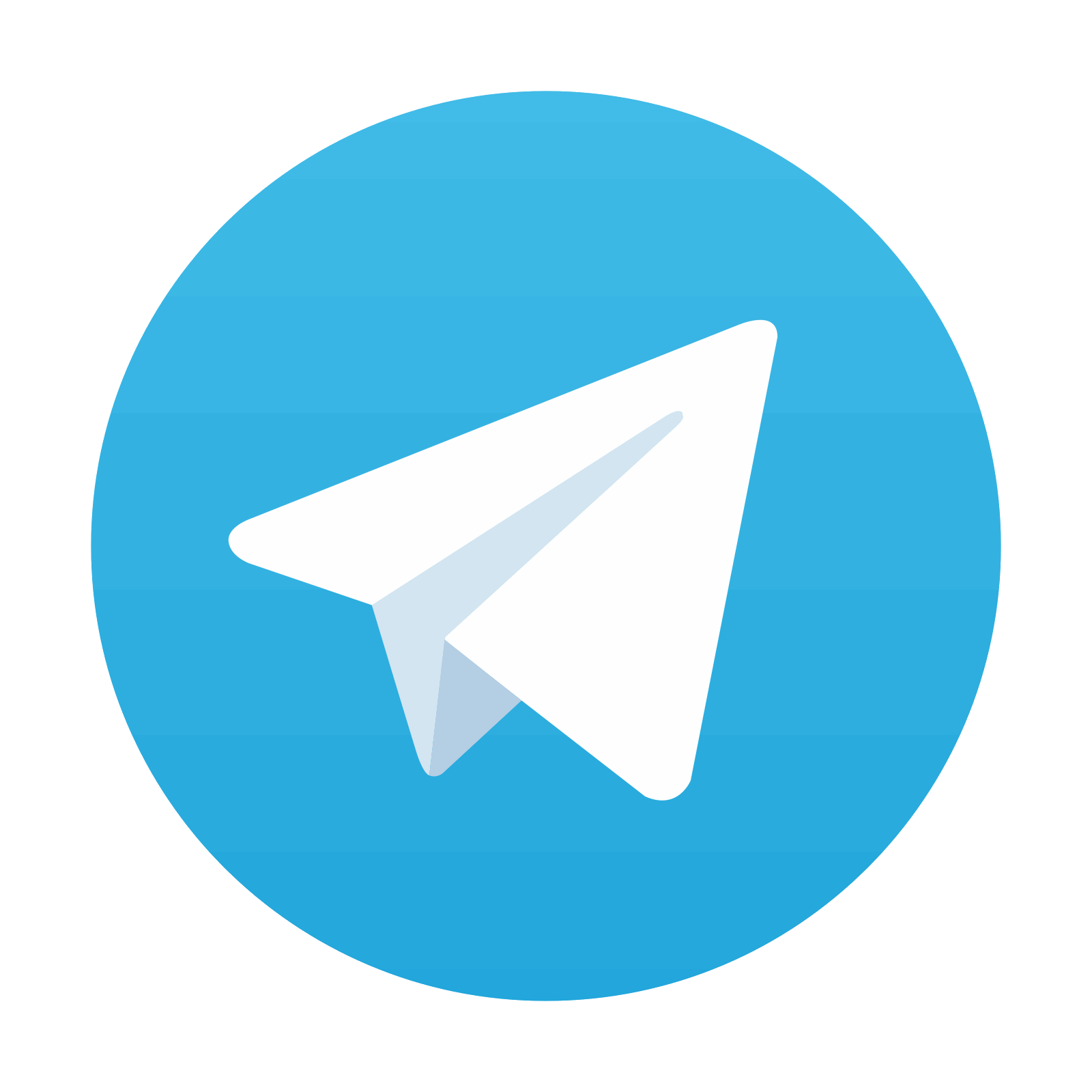
Stay updated, free articles. Join our Telegram channel

Full access? Get Clinical Tree
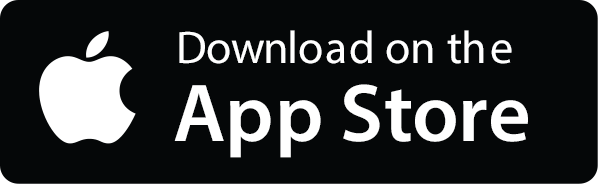
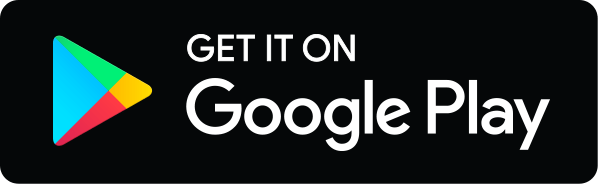