Chapter 54
Substance Abuse
Lisa R. Leffert MD
Chapter Outline
Abuse of licit and illicit substances by pregnant women can pose a significant risk to maternal and fetal health. Estimates of the prevalence of substance abuse in pregnant women vary depending on the particular licit or illicit substance, the maternal age group, and the data source.1–3 The rate of illicit drug use during pregnancy as reported in the National Survey on Drug Use and Health from 2009 to 2010 was 16.2% among pregnant women aged 15 to 17 years, 7.4% among pregnant women aged 18 to 25 years, and 1.9% among pregnant women aged 26 to 44 years. During the same time period, among pregnant women aged 15 to 44 years, 4.4% were current illicit drug users. This 4.4% rate was lower than the rate among nonpregnant women (10.9%).1
Depending on the particular substance ingested, pregnant women may experience little to no acute and chronic adverse effects, or alternatively, manifest one or more of the following: (1) cardiovascular, pulmonary, and neurologic complications; (2) obstetric complications (e.g., decreased intrauterine fetal growth, preterm labor, placental abruption, fetal death); and (3) heightened sensitivity to pain.4–12 Anesthesia providers often care for these patients during the provision of analgesia for labor and anesthesia for cesarean delivery as well as during other obstetric procedures before and after delivery. Patients with substance abuse may have altered pain sensitivity, and anesthesia providers are often consulted for help with management of acute postoperative pain.
Drug Detection
Optimal care requires developing a therapeutic bond with these patients and identifying what substances have been ingested.13 The anesthesia provider should ask questions in a respectful and nonjudgmental manner. It is vital to respect patient confidentiality, and it may be necessary to speak to the patient without family or friends present. Self-reporting typically underrepresents the true incidence of substance abuse.14–16 Therefore, health care providers should be familiar with the characteristic signs and symptoms associated with acute and chronic intoxication. The myriad of methods available to test pregnant patients and their infants for the presence of illicit drugs include analysis of urine, blood, saliva, hair, meconium, and umbilical cord tissue. Amniotic fluid and neonatal gastric aspirate have also been analyzed (Tables 54-1 and 54-2).14–19 It is vital to understand which compounds a particular drug test identifies before interpreting the results. Caregivers should be aware that the immunoassays most commonly used in drug testing can have false-positive or false-negative results in the presence of structurally related drugs or additives. Gas chromatography with mass spectrometry ideally should be used to provide confirmation of positive results.16
TABLE 54-1
Drug Detection: Overview
Specimen | Advantages | Limitations |
Urine | Detection of diverse group of illicit substances (except volatile alcohols) Specimen and test readily available Short turnaround time (30 minutes at point of care; 2 hours for laboratory specimens) More sensitive test (compared with meconium and hair) for cannabis | Underrepresents most illicit drug use Significant false-positive rate for phencyclidine (PCP) Narrow detection window compared with that for meconium and hair Specimen can more easily be adulterated |
Blood | Most commonly used for volatile alcohols (can detect other illicit substances) Specimen and test readily available | Invasive Narrow detection window compared with that for urine, meconium, and hair |
Meconium | Highly sensitive (compared with urine testing) for cocaine and opioids Wide detection window No false-positive results for cocaine | Report may be delayed (days) Low sensitivity and specificity for detecting cannabinoids and amphetamines via immunoassay |
Hair | Highly sensitive test for detecting cocaine (three times that of urine) and opioids Wide detection window (reflects chronic cumulative use) Samples can be stored at room temperature Samples can be analyzed remote from collection | Multiple hairs required; harvested close to scalp Environmental contamination may cause false-positive result Low sensitivity for detecting tetrahydrocannabinol |
Umbilical cord blood | Comparable to meconium with more rapid results May reflect a wide window of detection | Specimen not available before delivery |
Oral fluid | Highly sensitive for methamphetamine and other basic drugs Easy, noninvasive Primarily detects parent compound | If mouth is dry, salivary stimulation may be associated with a decreased drug concentration in oral fluid |
Data from references 3, 14, 15, 17, and 165.
TABLE 54-2
Drug Detection Window in Urine*
Drug† | Analyte | Detection Window |
Tobacco | Cotinine Nicotine | 19 h (urine T1/2) 2 h (urine T1/2) |
Cocaine | Cocaine Benzoylecgonine | 3-6 h IV use: 1-2 days Intranasal use: 2-3 days |
Amphetamines | Amphetamine Methamphetamine | 1-3 days Smoked: 60 h |
Methylenedioxymethamphetamine (MDMA, ecstasy) | MDMA | 1-3 days |
Marijuana (cannabis) | Tetrahydrocannabinol (THC) THCCOOH | Smoked: 10 h Up to 25 days |
Lysergic acid diethylamide (LSD) | LSD 2-Oxo-3-OH-LSD | 24 h 96 h |
Heroin | 6-Acetyl morphine Morphine | IV use: 2-4.5 h 19-54 h |
Benzodiazepines | Flunitrazepam: 7-aminoflunitrazepam | < 72 h Chronic use: 4-6 wk |
γ-Hydroxybutyric acid (GHB) | Rapidly metabolized to CO2 and H2O | < 12 h |
* Average values based on recent use; precise values may vary according to method of ingestion, assay employed, and duration of use.
† Detection of methadone, buprenorphine, oxycodone, and oxymorphone typically requires an additional screening test.
IV, intravenously; T1/2, half-life.
Data from references 5, 19, 74, 120, and 166.
Licit Drugs
Alcohol
Epidemiology
Alcohol (ethanol) abuse represents a significant problem for pregnant women and their developing fetus. Since 1981, official advisories have warned against the use of alcohol by pregnant women or women considering pregnancy.20 Yet the 2010 National Survey on Drug Use and Health noted that 10.8% of pregnant women 15 to 44 years old reported current alcohol use, 3.7% reported binge drinking, and 1.0% reported heavy drinking. These rates are significantly lower than those of nonpregnant women in the same age brackets (54.7%, 24.6%, and 5.4%, respectively). Survey data indicate that among heavy drinkers, approximately 1.8% also use illicit drugs.1
Pharmacology
Alcohol, usually ingested orally, is absorbed through the gastrointestinal tract, primarily within the small intestine. Ingested alcohol is metabolized by alcohol and acetaldehyde dehydrogenases. This process leads to the production of acetaldehyde and the reduction of nicotinamide adenine dinucleotide (NAD) to its reduced form NADH. The excess reduced form relative to NAD results in metabolic derangements. A small residual amount (2% to 8%) of alcohol is excreted via the lungs, urine, and sweat.21–23
Systemic Effects
According to legal statutes, the definition of “intoxication” requires a blood alcohol level of at least 80 to 100 mg/dL, although behavioral, cognitive, and psychomotor changes can occur at levels of 20 to 30 mg/dL (e.g., after one to two drinks) (Table 54-3).21–23
Alcohol has complex effects on the central nervous system (CNS); it acts as both a depressant and a stimulant through a variety of neurotransmitter pathways.23 When alcohol is consumed in conjunction with barbiturates or benzodiazepines, these effects can be compounded. Endogenous opioids interact with alcohol to “reinforce” further alcohol use; this effect is blunted by opioid antagonists.23
Alcohol and its metabolites (e.g., acetaldehyde) can be directly toxic to brain tissue.21,22 Chronic alcoholism is associated with brain atrophy that results in impairment of memory, abstract problem-solving, verbal learning, and visual-spatial processing.21,22 Additional adverse neurologic effects result from vitamin (e.g., thiamine, vitamin B12) deficiencies.21,22
Heavy alcohol consumption also damages other organs. Over time, hepatic cirrhosis can develop, which, in turn, can lead to encephalopathy, coagulopathy, and esophageal varices (Table 54-4). Gastrointestinal mucosa injury, pancreatitis, and cardiomyopathy may also occur.9,10,22,24
Women who abuse alcohol are at increased risk for depression, suicide, and accidents.7 Symptoms of acute alcohol withdrawal (e.g., nausea, vomiting, tachycardia, hypertension, arrhythmias, tremor, hallucinations, agitation, seizures) usually occur within 6 to 48 hours after cessation of alcohol consumption (Table 54-5).9,10,25 Pharmacologic therapy to minimize the signs and symptoms of alcohol withdrawal includes the use of benzodiazepines and α2-adrenergic agonists (e.g., clonidine).9 The most severe form of withdrawal symptoms, delirium tremens, manifests as agitation, disorientation, hallucinations, and fever combined with autonomic instability. Delirium tremens occurs rarely in pregnant women, but it can lead to maternal and fetal death if untreated.6, 26
Effects on Pregnancy and the Fetus
Intrauterine alcohol exposure is the leading cause of preventable birth defects in the United States.27,28 No safe level of alcohol consumption by pregnant women has been identified.26,27 In contrast to other investigations of high-risk populations, a 2010 population-based cohort study found no association between low or moderate prenatal alcohol exposure before or during pregnancy and the occurrence of birth defects.29 However, the authors recognized that the incidence of birth defects is low and the sample size may not be sufficient to draw definitive conclusions. Fetal alcohol syndrome is defined as the presence of particular neonatal facial features (e.g., small palpebral fissures, flat midface with a short upturned nose, thin upper lip) and significant impairment in neurodevelopment and physical growth.9,10,27,30 Fetal alcohol spectrum disorders refer to the wide range of possible adverse effects of fetal exposure to alcohol.27 The extent to which an individual fetus is affected is related to the characteristics of the exposure, genetic variables, and the intrauterine environment.31
Alcohol exposure is also associated with pregnancy loss. Harlap and Shiono32 reported an increased risk for second-trimester fetal loss in pregnant women who consumed alcohol early in pregnancy (relative risk, 1.98 for those consuming one to two drinks daily and 3.53 for those consuming more than three drinks daily, compared with nondrinkers). Identification of mothers at risk can facilitate treatment and perhaps improve pregnancy outcomes.27
Anesthetic Management
Alcohol-intoxicated parturients are at increased risk for behavioral problems, electrolyte abnormalities, greater gastric acid secretion, and co-intoxication with other substances.9,10,26 Determining whether the patient can protect her airway is of paramount importance because acute intoxication increases the risk for pulmonary aspiration of gastric contents. In addition, these patients may have intravascular volume depletion secondary to vomiting, inadequate oral intake, diuresis, and hypoalbuminemia. Significant alcohol ingestion in the setting of poor oral intake may also manifest as severe hypoglycemia.22,24,33
Neuraxial analgesia or anesthesia can be safely administered for labor or cesarean delivery provided that (1) the patient is cooperative, (2) there is no evidence of coagulopathy (as a result of liver disease), (3) the patient is volume replete, and (4) baseline neurologic deficits (e.g., peripheral neuropathy, cognitive deficits) are assessed and documented.9
If emergency delivery is required and the patient is either uncooperative or too sedated to protect her airway, general anesthesia will be necessary. The patient should receive pharmacologic aspiration prophylaxis (e.g., nonparticulate antacid, histamine-2 (H2)-receptor antagonist, metoclopramide) and should undergo a rapid-sequence induction of general anesthesia.34
Evidence from published reports is inconclusive about predictable differences in anesthetic requirements in patients with acute and chronic alcohol use.35 Acute alcohol intoxication is believed to decrease a patient’s anesthetic requirements, in part because of the additive effect of alcohol and other CNS depressants. The notion that chronic alcoholics require more anesthesia than their non–alcohol-using counterparts is based primarily on data from an abstract published by Han in 1969,36 who demonstrated that the mean minimum alveolar concentration (MAC) for halothane in six chronic alcoholic patients who had been heavy drinkers for more than 10 years was significantly greater than that for six healthy adults. Subsequently, Swerdlow et al.37 assessed the response to thiopental in 11 nonpregnant, chronic alcohol users. After eliminating potential confounders such as acute intoxication, withdrawal, polysubstance abuse, and end-organ dysfunction, they found that chronic alcohol intake did not alter thiopental dose requirements, pharmacokinetics, or pharmacodynamics. No large population studies have assessed dose requirements for volatile anesthetic agents or hypnotic agents in patients who chronically abuse alcohol.
Short-term consumption of alcohol inhibits the metabolism of drugs by the liver (through competition for cytochrome P450), which results in higher plasma concentrations of drugs metabolized by the liver. Long-term consumption of alcohol increases the levels of cytochrome P450, resulting in decreased levels of medications such as diazepam and labetalol, and increased levels of toxic metabolites that occur from hepatic degradation of illicit drugs such as cocaine.22 Both pregnancy and liver disease can lead to decreased plasma concentrations of pseudocholinesterase; however, this decrease does not seem to have a clinically significant effect on the degradation of succinylcholine and ester local anesthetics.35
Parturients who regularly consume large amounts of alcohol and undergo general anesthesia for cesarean delivery may be at high risk for awareness under anesthesia.38 The high doses of volatile anesthetics often recommended in nonpregnant, chronic alcohol-using patients can lead to significant uterine atony and potential increased blood loss. Therefore, a balanced anesthetic technique that combines induction with generous doses of the hypnotic agent with succinylcholine (if not otherwise contraindicated), followed by maintenance with a volatile anesthetic agent (limited to 0.5 to 0.8 MAC after delivery to prevent uterine atony), nitrous oxide (up to 67%), and an opioid and a benzodiazepine (for analgesia and amnesia), should be employed. Withholding additional muscle relaxation after induction and adding a brain function monitor if time and circumstance permits39 may help to identify patients who could benefit from additional anesthesia.
Tobacco
Epidemiology
Cigarette smoking is the most common form of substance abuse during pregnancy. As public awareness has grown regarding the hazards of smoking during pregnancy, the prevalence of cigarette smoking during pregnancy has declined over the past 40 years. An estimated 18% of pregnant women reported smoking (in the past month) in 2003,40 compared with 16% in 2010.1 Although the overall smoking rate in pregnant women is lower than in nonpregnant women, the rate among pregnant teens aged 15 to 17 years is actually higher than that among nonpregnant teens (22.7% versus 13.4%).1 Among the approximately 40% of patients who stop smoking when they discover that they are pregnant, 60% to 80% return to smoking by 6 months postpartum.41
Pharmacology
More than 4000 chemicals are found in tobacco, including nicotine, carbon monoxide, and cyanides.23,42 Tobacco is most often smoked, but it can also be chewed or sniffed. Nicotine, the principal drug of abuse in tobacco, acts at peripheral and central nicotinic (acetylcholine) receptors throughout the body to affect the release of catecholamines. Nicotine’s effects begin immediately on exposure; it is then rapidly metabolized in the liver and the lungs and excreted by the kidneys. The half-life is typically a few hours. The duration of the acute effects of nicotine is shorter in heavy smokers than in light smokers.23
Carbon monoxide, another constituent of cigarette smoke, interferes with oxygen delivery to the cells by competitively binding to hemoglobin, thereby decreasing the oxygen-binding capacity and shifting the oxyhemoglobin dissociation curve to the left.43 Depending on the extent of smoke inhalation, carbon monoxide may occupy 3% to 15% (or more) of the oxygen-carrying capacity of the blood.44
Systemic Effects
Smoking alters maternal physiology through both the acute pharmacologic actions of tobacco’s chemical constituents and their contribution to comorbid disease. Peripherally, nicotine increases sympathetic tone, thereby increasing maternal heart rate, blood pressure, and cardiac work (see Table 54-3).45 Nicotine affects neurotransmitter release in different areas of the brain, producing feelings of alertness, euphoria, and, ultimately, dependence.23,45
Increased production of carboxyhemoglobin is thought to be a major factor in the impaired wound healing observed in smokers.43 Smoking also promotes atherosclerosis. The effects of tobacco smoking on the lungs include changes in the volume and composition of mucus, impaired mucociliary clearance, and an increased incidence of bronchitis and chronic obstructive pulmonary disease (see Table 54-4).45
Tobacco is addictive, and cessation of its use produces withdrawal symptoms, including cravings, irritability, headache, cough, and insomnia (see Table 54-5). Smoking cessation interventions include counseling and therapy, hypnosis, acupuncture, and pharmacologic therapy. The use of nicotine replacement therapy (e.g., nicotine gum, patch, inhaler) has not undergone sufficient evaluation of safety during pregnancy; thus, the American College of Obstetricians and Gynecologists (ACOG) has recommended that nicotine replacement therapy be used only when nonpharmacologic interventions have failed.40 The physiologic benefits of smoking cessation are progressive. Even brief smoke-free intervals can result in a reduction in the carboxyhemoglobin concentration, improved ciliary function, and decreased small airway obstruction.46
Effects on Pregnancy and the Fetus
Nicotine has a low molecular weight and readily crosses the placenta.4 Smoking may result in decreased fetal oxygenation as a result of increased concentrations of carboxyhemoglobin and reduced uteroplacental perfusion. It also leads to decreased uptake of nourishing amino acids by the placenta.42 Smoking is associated with a higher incidence of ectopic pregnancy, spontaneous fetal loss, placental abruption, and sudden infant death syndrome (SIDS).32,40,47 Smoking may protect against the occurrence of preeclampsia (see Chapter 36).48
Smoking adversely affects fetal growth.42,49,50 Low birth weight (LBW) is associated with increased neonatal and infant mortality.42,50 Salihu et al.50 documented that infant mortality was 40% higher in the offspring of women who smoked than in the offspring of nonsmoking women; this risk increased in a dose-dependent fashion for the infants who were small for gestational age (SGA). Smoking cessation before the third trimester ameliorates the smoking-associated reduction in birth weight.40
Intrauterine exposure to smoking (tobacco and/or marijuana) may also have long-term effects. Fried et al.51 examined the cognitive performance in 145 adolescents (13 to 16 years of age) from a low-risk, middle-class population. After controlling data for socioeconomic status and polysubstance abuse, they found that measures of overall intelligence and auditory memory were negatively related to prenatal maternal smoking in a dose-related fashion.
Anesthetic Management
Smoking is a risk factor for several perioperative complications, including respiratory sequelae and impaired wound healing.43,45 Smoking results in increased airway secretions, decreased ciliary motility, and impaired gas exchange.45 Smoking is also associated with an increase in nonspecific airway reactivity, and tracheal intubation may provoke bronchospasm. Wilkes et al.52 observed that coughing after exposure to desflurane was more pronounced in smokers. By contrast, Kim and Bishop53 observed that although most patients coughed as they awakened from general anesthesia maintained with isoflurane, smokers were not more likely to cough than nonsmokers.
Six months of abstinence may be required before the function of alveolar macrophages and pulmonary cytokines during and after general anesthesia in former smokers is similar to that of nonsmokers.46 Administration of neuraxial anesthesia avoids airway manipulation and is typically preferred in parturients who smoke.
Caffeine
Epidemiology
On a daily basis, 80% to 98% of women drink caffeine-containing beverages.9,54 The prevalence of consumption of caffeine-containing beverages during pregnancy is unknown.
Pharmacology
Caffeine (1,3,7-trimethylxanthine) is a naturally occurring alkaloid found in coffee, tea, cocoa, and some soft drinks and medicines.9,54,55 The primary sources of caffeine in the adult diet are coffee (56 to 100 mg/100 mL if brewed) and tea (20 to 73 mg/100 mL). Caffeine is readily absorbed through the gastrointestinal tract, and maximum blood concentrations of caffeine are attained 1 to 1.5 hours after ingestion. Caffeine undergoes hepatic metabolism and is then excreted in the urine.54,55 The elimination half-life of caffeine is 3 to 7 hours. In pregnancy, the half-life increases from 4 hours in the first trimester to 18 hours by the third trimester. Caffeine crosses the placenta and can also be found in breast milk.54,56 Habitual use of caffeine at levels greater than 500 to 600 mg/day is defined as abuse.54,56
The half-life of caffeine in the neonate is prolonged in comparison with that in children and nonpregnant women.54
Systemic Effects
Caffeine acts as an antagonist at the adenosine receptor. In the absence of the inhibitory effects of adenosine, the neurotransmitters norepinephrine, dopamine, and serotonin are released in increased concentrations.54,56 Systemic effects of caffeine include CNS stimulation, changes in blood pressure and metabolic rate, and diuresis (see Table 54-3).55 The side effects commonly attributed to caffeine vary among individuals, in part related to the doses ingested and the degree of chronic use. Studies of the effects of caffeine on alertness, vigilance, mood, and memory have produced inconsistent results.54
Moderate caffeine intake (≤ 400 mg/day or ≤ 4 cups of coffee/day) does not seem to negatively affect cardiovascular health in most people. Although some people who ingest caffeine report tachycardia and palpitations, doses lower than 450 mg/day do not appear to increase significant cardiac arrhythmias in either healthy patients or in those with ischemia or ventricular ectopy. Caffeine doses as low as 250 mg have been reported to have a hypertensive effect after acute ingestion (an increase in systolic blood pressure of 5 to 15 mm Hg and an increase in diastolic blood pressure of 5 to 10 mm Hg), particularly in caffeine-naive individuals; however, epidemiologic studies have produced inconsistent results. In general, people who ingest caffeine on a long-term and frequent basis are less likely than occasional users to have difficulty sleeping.54
Caffeine appears to affect bladder function in women. Moderate caffeine intake may exacerbate preexisting bladder symptoms, and excessive intake (> 400 mg/day) increases the risk for bladder dysfunction.54
Evidence suggests that caffeine is not a human carcinogen.55 The lethal dose of caffeine in humans has been estimated to be 10 g; however, only a few such cases have been reported.54
Caffeine withdrawal is associated with headache, anxiety, depressed mood, and fatigue (see Table 54-5). Typically, symptoms begin 12 to 24 hours after cessation of use, peak at 20 to 48 hours, and last up to 7 days. The severity and likelihood of symptoms are not predictable.54
Effects on Pregnancy and the Fetus
Caffeine readily crosses the placenta. Whereas animal studies have shown that very high doses can have a teratogenic effect, moderate doses do not appear to result in teratogenesis in humans.54,57,58 There is some evidence that caffeine at doses greater than 300 mg/day may result in fetal growth restriction (also known as intrauterine growth restriction) and decreased birth weight, particularly in women who also smoke or drink significant amounts of alcohol.54 Although previous studies56 have not shown an association between low or moderate caffeine intake (< 300 mg/day) and greater risk for spontaneous abortion or preterm delivery, Weng et al.59 found that caffeine consumption greater than 200 mg/day was associated with an increased risk for miscarriage, particularly among pregnant women who did not have a previous history of miscarriage. Moderate intake of caffeine in lactating women does not adversely affect postnatal development.54,55
Two studies have suggested that moderate caffeine intake during pregnancy may actually have beneficial effects on the mother and the fetus. Adeney et al.60 found that women who consumed caffeinated coffee before conception had a significantly lower risk for development of gestational diabetes mellitus than women who did not consume coffee. No reduction in risk was associated with ingestion of tea or soda. Back et al.61 showed that neonatal mice exposed to caffeine and subsequently subjected to hypoxia seemed to have less neurologic injury (i.e., less ventriculomegaly, less disruption in myelination) than non–caffeine-exposed mice.
Anesthetic Management
Caffeine may enhance the side effects of beta-adrenergic receptor agonists such as epinephrine and albuterol. Caffeine may also increase the risk for a hypertensive crisis in patients taking monoamine oxidase (MAO) inhibitors. Caffeine slows the elimination of theophylline and acetaminophen, resulting in higher drug concentrations in the blood. In contrast, serum concentrations of lithium may be decreased secondary to caffeine-enhanced elimination.55
Perhaps of greatest significance to the anesthesia provider is the potential for caffeine withdrawal headache in parturients who abruptly decrease their usual caffeine intake during labor and delivery. Caffeine withdrawal should be considered in a postpartum patient who has a nonspecific, nonpositional headache without associated lateralizing neurologic findings (see Chapter 31). Evidence for the efficacy of caffeine in the treatment of post–dural puncture headache is scant (see Chapter 31).62 Resumption of caffeine ingestion should be considered for treatment of caffeine withdrawal headache.
Illicit Drugs
Marijuana (Cannabis)
Use of marijuana for medical and recreational purposes can be traced back thousands of years.63 In 2010, approximately 2.4 million people in the United States used marijuana for the first time.1 Marijuana was the illicit drug with the largest number of new users aged 12 or older. Estimates of marijuana use in the pregnant population vary from 6% to 7%.17,64
Pharmacology
Marijuana contains more than 400 compounds, including 60 cannabinoids. Most of the psychotropic effects are caused by 9-tetrahydrocannabinol (THC).63,65 Noncannabinoid constituents of marijuana are similar to those in tobacco without the nicotine. Inhaled THC is absorbed through the lungs and reaches the brain within minutes. Oral ingestion of marijuana results in blood THC concentrations that are 25% to 30% of those obtained by smoking, but with a delayed onset of up to 2 hours. Cannabinoids are highly lipid-soluble compounds that are sequestered in fatty tissues and are gradually released into other tissues. Thus, a single ingestion can have an elimination half-life of up to 7 days; complete elimination of the inactive metabolite 11-nor-9-carboxy-delta-9-tetrahydrocannabinol (THCCOOH) takes as long as 25 to 30 days. Metabolism occurs in the liver, with excretion in the urine. Measured concentrations of THC and other cannabinoid metabolites in the blood and urine correlate poorly with the degree of intoxication.65
Systemic Effects
Cannabis interacts with specific cannabinoid receptors in the brain and peripheral nerves. Psychoactive effects include anxiolysis, analgesia, appetite stimulation, euphoria, and, sometimes, dysphoria (see Table 54-3).65 Marijuana intoxication impairs cognitive and psychomotor function. Impairment of memory, attention, and the ability to process complex information can occur with long-time heavy use (see Table 54-4). It is unclear whether these effects are reversible.66
Acute intoxication with marijuana appears to have a biphasic effect on the autonomic nervous system; low doses cause tachycardia and higher cardiac output due to increased sympathetic and decreased parasympathetic tone. In contrast, high doses produce sympathetic inhibition and parasympathetic stimulation, resulting in bradycardia and hypotension.5,63 Although ventricular ectopy can occur, life-threatening arrhythmias in patients without preexisting cardiac disease are rare and autonomic disturbances are generally well tolerated. Reversible ST-segment and T-wave abnormalities may occur, perhaps as a result of the higher heart rate associated with marijuana use.5
As with tobacco, the respiratory consequences of smoking marijuana include mucociliary dysfunction, increased susceptibility to bronchitis, and chronic obstructive pulmonary disease (see Table 54-4). Acute intoxication may cause conjunctival vasodilation and visible reddening of the eyes. Although high doses of marijuana can cause hallucinations and psychosis, fatal overdose has not been documented.6,65
Withdrawal from long-time marijuana use may produce a mild abstinence syndrome that includes headache, restlessness, tremor, anxiety, and autonomic effects, similar to those of withdrawal from benzodiazepines and hypnotic drugs (see Table 54-5).5,66
Effects on Pregnancy and the Fetus
It is difficult to ascertain specific effects of marijuana on pregnancy, because women who use marijuana often engage in polysubstance abuse. In a prospective study of more than 3800 pregnancies, Hatch and Bracken66 showed that regular marijuana use by white women was associated with an increased risk for delivering an LBW and/or SGA infant, but occasional use was not. The risk for these adverse outcomes in nonwhite marijuana-using women was not elevated above their baseline level of increased risk. In a prospective multicenter study of more than 7000 multiethnic pregnant women, marijuana use was not associated with increased risk for LBW, preterm delivery, or placental abruption.67
Intrauterine exposure to marijuana has not been proven to be teratogenic, but some research suggests that it is associated with subtle postnatal neurologic derangements. Fried et al.51 did not find an association between maternal marijuana use and overall intelligence quotient (IQ) and verbal memory in exposed offspring; however, certain aspects of cognition, such as memory at 4 years of age, attention at 6 years, attention and visually related cognition at 9 to 12 years, and stability of attention at 13 to 16 years, were negatively associated with prenatal marijuana exposure.
Anesthetic Management
Neuraxial analgesia is typically preferred during labor and delivery and can be safely performed in patients who use marijuana, in the absence of other contraindications. Drug-related changes in heart rate and blood pressure are usually well tolerated in otherwise healthy patients who are acutely intoxicated. However, the administration of atropine, pancuronium, and ketamine can exacerbate existing tachycardia.63
Long-time marijuana smokers are at risk for many of the same respiratory complications during and after general anesthesia as are tobacco cigarette smokers; these patients may have increased airway secretions, impaired mucociliary clearance, and potentially increased airway reactivity.45,65 Persistent postextubation laryngospasm in a patient with a history of heavy marijuana smoking has been reported.68
Acute intoxication with marijuana can have additive effects with those of sedative agents and volatile anesthetic agents, including myocardial depression24,63; careful titration to clinical effect is recommended.
The effect of marijuana on pain perception has been explored. Wallace et al.69 presented a model of human experimental pain in which smoked marijuana had a differential effect on pain scores; a low dose had no effect, a medium dose reduced pain, and a high dose significantly increased pain. The clinical implications of these findings are unclear.
Cocaine
The use of cocaine, extracted from the leaf of the South American Erythroxylum coca bush, can be dated as far back as 600 ad.70 Cocaine was introduced into clinical practice as a local anesthetic in the 1880s. During this period, Sigmund Freud also experimented with cocaine’s ability to combat hunger and fatigue and opiate addiction.70,71 Cocaine has both vasoconstrictive and local anesthetic properties as a result of its ability to block sodium channels during depolarization.
Epidemiology
The prevalence of cocaine use during pregnancy is difficult to estimate. Studies using a variety of detection techniques have reported rates that range from 1.8% to greater than 10% depending on the method and the population studied.70 Survey data demonstrate that cocaine abuse in pregnancy is distributed broadly across ethnic and socioeconomic groups. In addition, an estimated 60% to 90% of pregnant cocaine users engage in polysubstance abuse, including the use of tobacco.72,73 As with other illicit substances, the self-reporting of cocaine use results in identification of fewer drug users than urine testing and use of other biologic markers.3 There is an association between cocaine use and an increased risk for sexually transmitted diseases, preterm labor, and failure to obtain prenatal care.3
Pharmacology
Cocaine (benzoylmethylecgonine) is an ester of benzoic acid and the base ecgonine, which is the parent compound of atropine and scopolamine.71,74 Cocaine is consumed in a variety of forms. When dissolved in hydrochloric acid to form a water-soluble powder (cocaine hydrochloride), it can be chewed, administered intravenously (“mainlined”), or taken intranasally (“snorted”). Intrarectal and intravaginal use has also been reported.75 When cocaine is processed with either sodium bicarbonate (“crack”) or ammonia and ether (“free base”), the resulting cocaine alkaloid can be smoked.71,74,76 The amount (dose) and duration of exposure are more important determinants of the effects of cocaine than is the chemical formulation. Smoked cocaine (“crack” or “free base”) is rapidly absorbed through the lungs and reaches the brain in 6 to 8 seconds, intravenous cocaine reaches the brain in 12 to 16 seconds, and snorted cocaine reaches the brain in 3 to 5 minutes.77 The typical half-life of cocaine is 30 to 90 minutes, although its effects can last as long as 6 hours.71
Cocaine is metabolized to ecgonine esters and benzoylecgonine (biologically inactive) by plasma and hepatic cholinesterases and to norcocaine (biologically active) by nonenzymatic hydrolysis. Only small amounts of cocaine are excreted unchanged in the urine. In the presence of alcohol, cocaine is transesterified to cocaethylene, which has a longer half-life and greater physiologic effects than cocaine.76,77
Systemic Effects
Cocaine has complex actions on the central and peripheral nervous systems and on nerve conduction. The powerful sympathomimetic effects of cocaine are due to the drug’s inhibition of the reuptake of norepinephrine, dopamine, and serotonin, which allows these neurotransmitters to accumulate at the synaptic clefts and produce sustained stimulation.70,76–78 This process can result in as much as a fivefold increase in circulating concentrations of catecholamines,5 which, in turn, may lead to feelings of euphoria, increased energy, and decreased fear.70,77 Repetitive use of cocaine eventually leads to depletion of neurotransmitter stores, up-regulation of receptors, and a higher dose requirement to achieve the desired euphoric effects.71
The peripheral nervous system effects of cocaine and its derivatives result from binding to tissue receptors involved in monoamine reuptake, which results in hypertension and/or labile blood pressure and tachycardia. Cocaine produces widespread small and large vessel occlusion through vasospasm, thrombosis, and endothelial injury, which may result in significant end-organ damage.71
Cocaine has profound effects on the cardiovascular system, and pregnancy appears to enhance them. Acute administration of cocaine increases peripheral vascular resistance, cardiac contractility, and myocardial oxygen demand (see Table 54-3).71,79 Coronary vasoconstriction also occurs; a greater effect occurs in diseased vessel segments than in nondiseased segments.76 Studies in vitro have indicated that cocaine can have a procoagulant effect in small and large vessels.5 This may lead to thrombus formation and coronary plaque rupture in the setting of cocaine-induced hypertension.71,80
Cocaine-induced chest pain is a common complaint among young people presenting to the emergency department.81 Mittleman et al.80 found that cocaine use was associated with a significant, abrupt, and transient increase in the risk for acute myocardial infarction in patients who were otherwise at low risk.82 The occurrence of cocaine-induced electrocardiographic changes is fairly common and is not necessarily associated with true ischemia.76
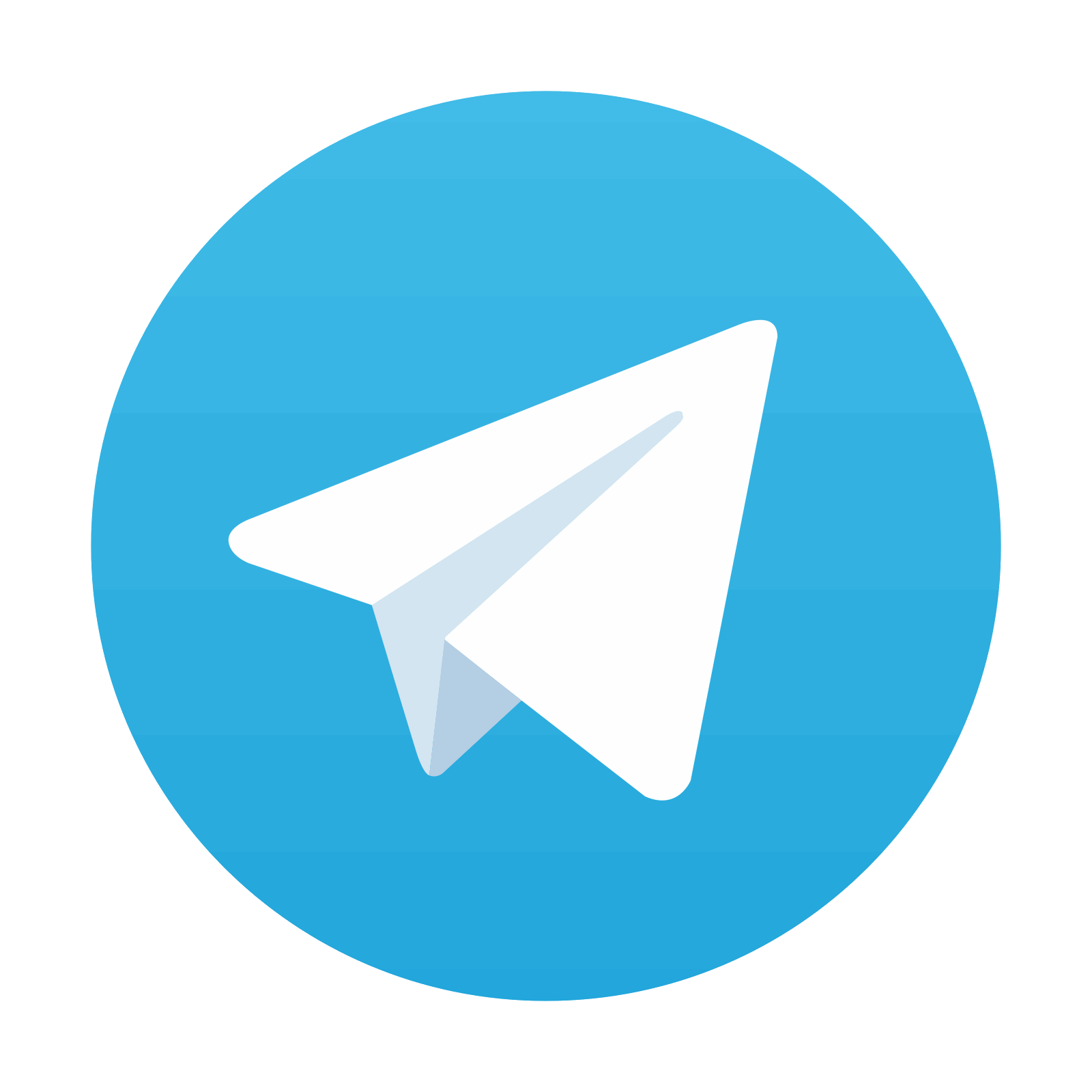
Stay updated, free articles. Join our Telegram channel

Full access? Get Clinical Tree
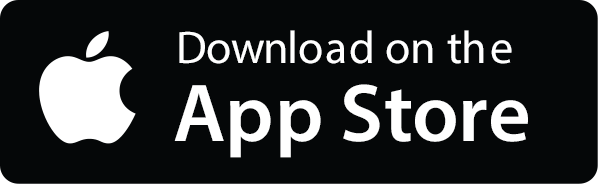
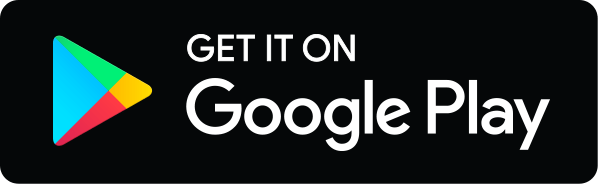
