Solid Organ Transplantation in Children
Liver Transplantation
Indications for Transplantation
Table 45-1 reviews the leading diagnoses that lead to LT. These disease entities define the bimodal age distribution of pediatric transplant recipients. Infants and children with biliary atresia and, occasionally, rapidly progressive hepatic failure secondary to metabolic abnormalities, such as neonatal tyrosinemia, hemochromatosis, or neonatal hepatic vascular tumors, are the patients who require transplantation early in life. Patients with hepatic tumors, metabolic disturbances, fulminant hepatic failure, and cirrhosis present as older children and adolescents requiring LT.
TABLE 45-1
Indications for Liver Transplantation at Cincinnati Children’s Hospital Medical Center, 1986–2007
Biliary Atresia
Children with extrahepatic biliary atresia constitute at least 50% of the pediatric LT population. Successful biliary drainage achieving an anicteric state following the Kasai portoenterostomy is the most important factor affecting preservation of liver function and long-term survival.1 Primary transplantation without portoenterostomy is not recommended in patients with biliary atresia unless the initial presentation is greater than 120 days of age and the liver biopsy shows advanced cirrhosis.2,3 We believe that the Kasai portoenterostomy should be the primary surgical intervention for all other infants with extrahepatic biliary atresia. Patients with progressive disease following a Kasai procedure should be offered early orthotopic liver transplantation (OLT). The sequential use of these two procedures optimizes overall survival and organ use.3
Patients with extrahepatic biliary atresia who are seen for transplantation form several cohorts. Infants with a failed Kasai have recurrent cholangitis, ascites, rapidly progressive portal hypertension, malnutrition, and progressive hepatic synthetic failure, and often require OLT within the first two years of life. Children with the successful establishment of biliary drainage have an improved prognosis, but this alone does not preclude the development of cirrhosis and portal hypertension leading to hypersplenism, variceal hemorrhage, ascites, and occasionally hepatopulmonary syndrome. These patients may require LT later in childhood. Patients with mild hepatocellular enzyme and bilirubin elevation, and mild portal hypertension can be observed with ongoing medical therapy. Approximately 20% of all patients with biliary atresia will not require OLT at some point in their life.4,5
Alagille Syndrome
Alagille syndrome (angiohepatic dysplasia) is an autosomal dominant genetic disorder that manifests as bile duct paucity which leads to progressive cholestasis and pruritus, xanthomas, malnutrition, and growth failure. Liver failure occurs late, if at all. Specific criteria for LT are difficult to quantify. Preoperative evaluation must include assessment for congenital cardiac disease and renal insufficiency, both of which are associated with this syndrome. Hepatocellular carcinoma (HCC) has also been seen in occasional patients.6,7
Experience using external biliary diversion or internal ileal bypass accompanied by ursodeoxycholic acid therapy has demonstrated a significant decrease in both pruritus and complications of hypercholesterolemia.8 Both of these procedures may ameliorate or decrease the rate of ongoing parenchymal destruction and cirrhosis, obviating the need for LT. Quality of life issues such as intractable pruritus, hypercholesterolemia, severe growth retardation, and intractable bone disease are criteria for consideration for LT.9–11
Metabolic Disease
An important indication for hepatic transplantation in older children is hepatic-based metabolic disease. In these patients, LT is not only lifesaving but also accomplishes phenotypic and functional cure. A review of these diseases and their mode of presentation are given in Box 45-1 and Table 45-2.
TABLE 45-2
aClassification of inherited metabolic disorders according to clinical modes of presentation.
Reprinted from Balistreri WF, Ohi R, Todani T, et al. Hepatobiliary, Pancreatic and Splenic Disease in Children: Medical and Surgical Management. Amsterdam: Elsevier Science; 1997. p. 395–9.
Hepatic replacement to correct the metabolic defect should be considered before other organ systems are affected, and before complications develop that would preclude transplantation, such as in patients with tyrosinemia, in whom there is a high risk of HCC.12 Although results of transplantation are excellent in the metabolic disease subgroup, replacement of the entire liver in order to correct single enzyme deficiencies is an inefficient, but presently necessary procedure. Current research centers around hepatocyte transplantation and gene therapy.13–17 These efforts may better serve this patient population in the future. Patients with primarily extrahepatic manifestations of their disease, such as cystic fibrosis, are occasionally helped by LT, although their prognosis is most often determined by their primary illness.18
Fulminant Hepatic Failure
Patients with fulminant hepatic failure without recognized antecedent liver disease present diagnostic and prognostic difficulties. Rapid clinical deterioration frequently makes establishment of a definitive diagnosis impossible before there is an urgent need for transplantation. Acute viral hepatitis of undefined etiology makes up the largest group, followed by drug toxicity and toxin exposure. Previously unrecognized metabolic disease must also be considered. Recently, an immune-based defect has been recognized as a cause of fulminant liver failure.19 This population needs to be identified as these children may require a combination of bone marrow and LT to achieve long-term survival. When acceptable clinical and metabolic stability make liver biopsy safe, diagnostic information allowing directed treatment of the primary liver disease is helpful. The presence of ongoing coagulopathy often dictates the need for an open approach to biopsy.
The prognosis of patients with fulminant liver failure is difficult to predict, and neurologic outcome is potentially suboptimal.16,20,21 Use of intracranial pressure (ICP) monitoring in patients with progressive encephalopathy has allowed early recognition and treatment for increased ICP. Monitoring should be instituted for patients with advancing grade III encephalopathy, and in all patients with grade IV encephalopathy. Intracranial monitoring is continued intraoperatively and for 24 to 48 hours after OLT, because significant increases in ICP can develop postoperatively. Failure to maintain a cerebral perfusion pressure greater than 50 mmHg and an ICP less than 20 mmHg has been associated with very poor neurologic outcomes.21 Also, survival following LT is significantly decreased in patients who reach grade IV encephalopathy. Efforts to identify and perform LT in children before this deterioration occurs are of utmost importance. When candidates are identified before they develop irreversible neurologic abnormalities, the results of transplantation are dramatically improved.
Liver Tumors
Transplantation for hepatoblastoma is recommended for individuals who, after the administration of several cycles of chemotherapy, have a neoplasm confined to the liver that is unresectable.22,23 Children who had prior isolated metastasis that disappeared while undergoing preoperative chemotherapy can be considered in select instances.24 A favorable response to pretransplant chemotherapy suggests a more favorable long-term outcome.25 In the current Children’s Oncology Group (COG) trial AHEP 0731, early referral for transplant evaluation is being evaluated for children who present with large lesions that appear unresectable.
Transplantation for HCC is complicated by less successful chemotherapy options and frequent extrahepatic involvement. The reported two-year survival rates are only 20–30%.26 Most deaths are due to recurrent HCC within the allograft or to extrahepatic tumor involvement. When primary HCC is discovered incidentally within the cirrhotic native liver at the time of hepatectomy, the overall prognosis is unaffected by the tumor.27
Donor Considerations
Donor Options
The single factor limiting the availability of LT is the supply of donor organs. The number of patients awaiting LT has increased by eleven fold since 1991.28,29 Available donor resources have not kept pace. As a consequence, the waiting time to transplant for all pediatric age groups has increased significantly, with young children and infants most affected (Figs 45-1 and 45-2). This severely limited supply of available donor organs has driven the advancement of many innovative liver transplant surgical procedures. The development of reduced-size LT allowed significant expansion of the donor pool for infants and small children. This not only improved the availability of donor organs but also allowed access to donors with improved stability and organ function. Evolution of these operative techniques has resulted in the development of both split-LT and live donor (LD) transplantation.
FIGURE 45-1 Graph depicting the mortality rate for adults and children on the liver transplant waiting list from 1999–2010. Note the largest number of deaths occur in infants less than 1 year of age. The numbers represent the age of the patient (in years). (Source: Scientific Registry Transplant Recipients—2011 Annual Report).
FIGURE 45-2 This diagram describes what has happened to children who have been listed for liver transplantation. Fortunately, most of the patients have undergone either live donor (LD) or deceased donor (DD) transplantation, although about 5% of patients are still on the transplant list after 36 months. (Source: Scientific Registry Transplant Recipients—2011 Annual Report).
In the hands of experienced transplant teams, these procedures all have similar success to whole organ transplantation. Furthermore, access to these donor options has reduced the waiting list mortality rate to less than 5%.
Organ Allocation
In 1998, the ‘final rule’ established by the Health Resources and Service Administration (HRSA) mandated the formation of a system for candidate stratification based on a continuous severity score reflecting 90 day waiting list mortality, i.e., outcome.30 The system for pediatric patients, the Pediatric End-Stage Liver Disease (PELD) score, was created using an analysis of the prospective registry of children listed for transplantation by the consortium Studies of Pediatric Liver Transplantation (SPLIT).31 The parameters selected included total bilirubin, international normalized ratio (INR), albumin, age <1 year, and evidence of failure to thrive (Box 45-2). The primary function of PELD is the stratification of candidates for LT by risk of 90 day waiting list mortality, allowing optimal use of donor organs. When death rates for all children listed were analyzed, PELD was an accurate predictor of mortality risk and demonstrated progressive risk until high scores were reached (>35) (Fig. 45-3).32
FIGURE 45-3 Pediatric End-Stage Liver Disease score predictive of survival after transplantation. (Redrawn from Barshes NR, Lee TC, Udell IW, et al. The PELD model as a predictor of survival benefit and of post transplant survival in pediatric liver transplant recipients. Liver Transpl 2006;12:475–80.)
Donor Selection
Assessment of donor organ suitability is undertaken by evaluating clinical information, static biochemical tests, and dynamic tests of hepatocellular function. Static biochemical tests identify preexisting functional abnormalities or organ trauma, but do not serve as good benchmarks to differentiate among acceptable and poor donor allografts. Donor liver biopsy is helpful in questionable cases to identify preexisting liver disease or donor liver steatosis. The shortage of donor organs has led to expanded efforts to use individuals of advanced age and marginal stability, termed ‘extended criteria donors’ (ECD).33 The evolving donor risk index (DRI) is used as a guide that quantifies relative risk of graft failure.34 In the future, organ allocation may be based on maximal life years gained, an approach being utilized in kidney allocation.35
Anatomic replacement of the native liver in the orthotopic position requires selection or surgical preparation of the donor liver to fill, but not exceed available space in the recipient. When using full-sized allografts, a donor weight range from 50–125% of the recipient weight is usually appropriate, taking into consideration body habitus and factors that would increase the abdominal size in the recipient such as ascites and hepatosplenomegaly. The right lobe graft, using segments 5 to 8, and the right trisegmentectomy graft using segments 4 to 8 can be accommodated when the weight difference is no greater than 2 : 1 between the donor-to-recipient (D:R). The thickness of the right lobe makes this allograft of limited usefulness in small recipients. Right lobe grafts from LDs have become widely used in adults. The left lobe, using segments 1 to 4, is applicable with a D:R disparity from 2.5 : 1 to 5 : 1, and a left lateral segment (segments 2 and 3) can be used with up to a 10 : 1 D:R weight difference.
The successful experience with in situ division of the living donor left lateral segment is a basis for the in situ split procedure. Two variations of the procedure are utilized depending on the needs of the recipients, a right–left lobe split or a right trisegmentectomy–left lateral segment split. For the right trisegmentectomy–left lateral segment split, the left lateral segment is prepared similar to a living related donor graft. The viability of segment 4 can be examined at the time of the division and is usually incorporated with the right lobe graft to increase the cellular mass of the allograft. For a left-right lobe graft, the parenchymal resection follows the anatomic lobar plane through the gallbladder fossa to the vena cava.36,37 A crush and tie technique is preferred to achieve good closure of the vascular and biliary structures. The bile duct, portal vein, and hepatic artery are divided at the right or left confluence. The vena cava is left incorporated with the allograft in both right and left lobe preparation. Vena caval reduction by posterior caval wall resection and closure is occasionally necessary. Resection of the inferior protruding portion of the caudate lobe is necessary during left lobe preparation to reduce the likelihood of arterial angulation, which can result in arterial thrombosis. This also facilitates shortening of the inferior vena cava to fit in a small recipient.38 When using left lateral segment (LLS) allografts, the parenchymal dissection follows the right margin of the falciform ligament with preservation of the left hilar structures. Direct implantation of the left hepatic vein into the combined orifice of the right and middle/left hepatic veins in the recipient vena cava is preferred; the donor vena cava is not retained with this segmental allograft. Further reduction of the LLS graft to a monosegmental graft may be necessary in very small recipients. Resection of the distal LLS is technically easier than an anatomic segment II/III division. Because this procedure adds considerably to the donor procurement time, and the necessary skill of the donor team, it is more demanding and occasionally difficult to successfully orchestrate. This technique is, however, despite these considerations, the preferred method for split-liver donor preparation.39,40
The benefits of split-liver transplantation are best achieved when ideal donors are selected. Strict restrictions on age, vasopressor administration, pre-donation hepatic function, and limited donor hospitalization have been used to select optimal donor candidates. When these donors are selected, the results from both in situ and ex-situ techniques are similar, with both techniques now having patient survival for both allografts of 90–93% and graft survival rates of 86–89%.41
The use of LDs has increased as the safety and success of this approach has been demonstrated (Fig. 45-4).42–44 One of the critical elements of LD transplantation is the proper selection of a donor, usually a parent or relative. This procedure is performed on the assumption that donor safety can be assured and that the donor’s liver function is normal. Careful attention to proper living donor consent is important. Parental concerns to help their ill child make true informed consent are a challenge. A dedicated ‘donor advocate’ not directly associated with the transplant team should assist with this process. Independent medical assessment of the donor is essential. United Network Organ Sharing (UNOS) has recently established clear criteria for this process.45 After a satisfactory medical and psychological examination by a physician not directly involved with the transplant program, computed tomography (CT) scanning is used to measure the volume of the potential donor segment to assure that it will meet the metabolic needs, but not exceed the space available in the recipient. If acceptable, CT angiography or arteriography is undertaken to assess the hepatic arterial anatomy, thereby excluding potential donors with multiple arteries to segments 2 and 3, and facilitating minimal hilar vascular dissection at the time of LT. Experience has shown that, when donors were deemed unacceptable, 90% of patients were excluded on the basis of history, examination, laboratory screening, and ABO type. Donor safety has been excellent in all pediatric LD series.46–48
FIGURE 45-4 The number of living donor and deceased donor liver transplants in infants and children performed between 1998–2011. (Source: Scientific Registry Transplant Recipients—2011 Annual Report).
In most pediatric cases, the LLS donated from an adult is used as the graft. In situ dissection of the LLS, preserving the donor vascular integrity until the parenchymal division is completed, is undertaken. At the time of harvest, the left hepatic vein is divided from the vena cava, and the left branch of the portal vein and hepatic artery are removed with the allograft.46 Vascular continuity of the hepatic arterial branches to segment 4 is maintained, if possible. Increased experience has been gained using the right lobe as an LD allograft for larger recipients such as adolescents and adults.42,49,50 This more extensive operation has proven to be a challenge to the donor and recipient alike, with complication and mortality rates significantly exceeding that of left lateral segmentectomy. The number of right lobe LD recipients now greatly exceeds the number of children receiving LD grafts;51 however, several publicized donor deaths and increased interest in ‘split-liver’ cadaveric procurement have slowed the enthusiasm and growth of right lobe donation.
The selection of a donor segment with an appropriate parenchymal mass for adequate function is critical to success. However, the minimal mass necessary for recovery is not yet established. Any calculation must take into account loss of function following preservation damage, acute rejection, and technical problems. When the D:R weight range falls within the normal 8 : 1 to 10 : 1 ratio, risk is minimal. Estimates of donor graft to recipient body weight ratio (GRWR) may prove to be a more accurate predictor of adequate graft volume. When the GRWR is less than 0.7%, overall allograft and patient survival suffered. In extreme cases in which small-for-size grafts are used, excessive portal flow can lead to hemorrhagic necrosis of the graft. Large-for-size allografts (GRWR >5.0%) have a less deleterious effect.52 A review of these donor anatomic options is shown in Figure 45-5.
The Transplant Procedure
Control of hemorrhage is essential during the recipient hepatectomy, requiring meticulous technique. Coagulation factor assays (V, VII, VIII, fibrinogen, platelets, prothrombin time, partial thromboplastin time) allow specific blood product supplementation to improve clotting function. Use of venovenous bypass is reserved for recipients >40 kg who demonstrate hemodynamic instability at the time of venous interruption.
Before re-establishing circulation to the allograft, anesthetic adjustments must be made to address the large volume of blood needed to refill the liver as well as hypothermic solutions released upon reperfusion. Inotropic support using dopamine (5–10 µg/kg/min) is also started. Calcium and sodium bicarbonate are administered to combat the effects of hyperkalemia from any remaining preservation solution or from systemic acidosis due to aortic and vena caval occlusion. Sufficient blood volume expansion, administered as packed red blood cells to raise the central venous pressure (CVP) to 15–20 cmH2O and the hematocrit to 40%, minimizes the development of hypotension on unclamping and prevents dilutional anemia. Cooperative communication between the surgical and anesthesia teams facilitates a smooth sequential reestablishment of vena caval, portal venous, and then arterial recirculation to the allograft.
Biliary tract reconstruction in patients with biliary atresia or in those weighing less than 25 kg is achieved through an end-to-side choledochojejunostomy using interrupted dissolving monofilament sutures. A multifenestrated Silastic internal biliary stent is placed before completing the anastomosis (Fig. 45-6). In most cases, the prior Roux-en-Y limb can be used, with a 30–35 cm length being preferred. Primary bile duct reconstruction without stenting is used in older patients with whole organ allografts.
FIGURE 45-6 Bile duct reconstruction is shown using the common hepatic duct in whole organ transplants (left) and segmental hepatic ducts into a Roux-en-Y intestinal limb for reduced-sized liver transplants (right). An internal multifenestrated stent is used in both situations. (From Ryckman F. Liver transplantation. In: Ziegler MM, Azizkhan RG, Weber T, editors. Operative Pediatric Surgery. New York: McGraw-Hill; 2003. p. 1275.)
Immunosuppressive Management
Most centers use an immunosuppressive protocol based on the administration of multiple complementary medications. All use corticosteroids and cyclosporine or tacrolimus. Additional antimetabolites (azathioprine, mycophenolate) are used when more intensive treatment is needed. Prior protocols using polyclonal or monoclonal induction therapy have been abandoned in most cases due to the extent of the immunosuppressive potency. A sample protocol is given in Table 45-3.
Postoperative Complications
Most postoperative complications present with cholestasis, increasing hepatocellular enzyme levels, and on occasion fever, lethargy, and anorexia. Therapy directed at the specific causes of the allograft dysfunction is essential. Empiric therapy for presumed complications is fraught with misdiagnoses, morbidity, and mortality. A flow diagram outlining this evaluation is shown in Figure 45-7.
FIGURE 45-7 Schematic flow diagram for management of postoperative liver allograft dysfunction. ATG, antithymocyte globulin; OKT-3, monoclonal antibody.
Primary Nonfunction
Primary nonfunction (PNF) of the hepatic allograft implies the absence of metabolic and synthetic activity following LT. Complete nonfunction requires immediate retransplantation before irreversible coagulopathy and cerebral edema occur. Lesser degrees of allograft dysfunction occur more frequently, and are associated with several donor, recipient, and operative factors (Box 45-3). The status of the donor liver contributes significantly to the potential for PNF. Ischemic injury secondary to anemia, hypotension, hypoxia, or trauma is often difficult to ascertain in the history of multiple trauma victims. Donor liver steatosis has also been recognized as a factor contributing to severe dysfunction or nonfunction in the donor liver.53,54 Macrovesicular steatosis on donor liver biopsy is somewhat more common in adult than pediatric donors and, when severe, is recognized grossly by the enlarged yellow, greasy consistency of the donor liver. The risk of PNF increases as the degree of fatty infiltration increases.54 Histologic findings are classified as mild if less than 30% of the hepatocytes have fatty infiltration, moderate if 30 to 60% are involved, and severe if more than 60% of the hepatocytes have fatty infiltration. Livers with severe fatty infiltration should be discarded, and donors with moderate involvement are used with some concern, with the degree of steatosis and the condition of the recipient determining use of the allograft.
The use of ABO-incompatible donors has been controversial. Allograft and patient survival rates in adult recipients have not been comparable to those achieved using ABO-identical or compatible donors.55,56 However, pediatric recipients of ABO-incompatible allografts have achieved survival rates equivalent to those using ABO-compatible and ABO-identical donors with either cadaveric donors (CDs) or LDs.57–59
Vascular Thrombosis
Hepatic artery thrombosis (HAT) occurs in children three to four times more frequently than in adult transplant series, occurring most often within the first 30 days following transplantation.60,61 Factors influencing the development of HAT are listed in Box 45-4. HAT presents with a variable clinical picture that may include: (1) fulminant allograft failure; (2) biliary disruption or obstruction; or (3) systemic sepsis. Doppler ultrasound (US) imaging has been accurate in identifying arterial thrombosis, and is used as the primary screening modality to assess vascular flow following transplantation or whenever complications arise. Acute HAT with allograft failure most often requires immediate retransplantation. Successful thrombectomy and allograft salvage is possible if reconstruction is undertaken before allograft necrosis.62 Biliary complications are particularly common following HAT. Ischemic injury to the biliary tree or anastomosis can result in intraparenchymal biloma formation or cholestasis. The development of septicemia or multifocal abscesses in sites of ischemic necrosis secondary to gram-negative enteric bacteria, Enterococcus, anaerobic bacteria, or fungi can also occur. Antibiotic therapy directed toward these organisms, along with operative or percutaneous drainage, is indicated when specific abscesses are identified. Drainage and biliary stenting may control bile leakage and infection until retransplantation is undertaken.
Prevention of HAT requires meticulous arterial reconstruction at the time of transplantation. Anatomic reconstruction is preferred in whole organ allografts; direct implantation of the celiac axis into the infrarenal aorta is recommended for all reduced-size liver allografts. All complex vascular reconstructions of the donor hepatic artery should be undertaken ex vivo whenever possible using microsurgical techniques before transplantation. When vascular grafts are required, they should also be implanted onto the infrarenal aorta.63 No systemic anticoagulation is routinely used by our group, but aspirin (20–40 mg/day) is administered to all children for 100 days.
Portal vein thrombosis (PVT) is uncommon in whole organ allografts unless prior portosystemic shunting has altered the flow within the splanchnic vascular bed or unless severe portal vein stenosis in the recipient has impaired flow to the allograft. Preexisting PVT in the recipient can be overcome by thrombectomy, portal vein replacement, or extra anatomic venous bypass. In biliary atresia recipients, portal vein hypoplasia is best corrected by anastomosis of the portal vein to the confluence of the splenic and superior mesenteric veins in the recipient. When there is inadequate portal vein length on the donor organ, iliac vein interposition grafts are used. Early thrombosis following LT requires immediate anastomotic revision and thrombectomy. Discrepancies in venous size imposed by reduced-size allografts can be modified to allow anastomotic construction.64,65 Deficiencies of anticoagulant proteins, such as protein C and S, and antithrombin III deficiency in the recipient must also be excluded as a contributing cause for vascular thrombosis.66 Failure to recognize PVT can lead to either allograft demise or, on a more chronic basis, to significant portal hypertension with hemorrhagic sequelae or intractable ascites.
Biliary Complications
Complications related to biliary tract reconstruction occur in approximately 10% of pediatric liver transplant recipients. Their spectrum and treatment is determined by the status of the hepatic artery and the type of allograft used. Although whole and reduced-size allografts have an equivalent risk of biliary tract complications, the spectrum of complications differs.67,68
Primary bile duct reconstruction is the preferred biliary tract reconstruction in adults, but it is less commonly used in children. It has the advantage of preserving the sphincter of Oddi, decreasing the incidence of enteric reflux and subsequent cholangitis, and not requiring an intestinal anastomosis. Experience using primary choledocho-choledochostomy without a T-tube has been favorable.69 Late complications following any type of primary ductal reconstruction include anastomotic stricture, biliary sludge formation, and recurrent cholangitis. Endoscopic dilation and internal stenting of anastomotic strictures has been successful in early postoperative cases. Roux-en-Y choledochojejunostomy is the preferred treatment for recurrent stenosis or postoperative leak.
Reconstruction of the bile ducts in patients with reduced-size allografts is more challenging. Division of the bile duct in close proximity to the cut-surface margin of the allograft, with careful preservation of the biliary duct collateral circulation, decreases but does not eliminate a ductal stricture secondary to ischemia. In our early experience, in 14% of patients with left lobe reduced-size allografts, a short segmental stricture developed requiring anastomotic revision (Fig. 45-8). Operative revision of the biliary anastomosis and reimplantation of the bile ducts into the Roux-en-Y is necessary. Percutaneous transhepatic cholangiography is essential to define the intrahepatic ductal anatomy before operative revision, and temporary catheter decompression of the obstructed bile ducts promotes treatment of cholangitis and allows elective reconstruction. Operative reconstruction is accompanied by transhepatic passage of exteriorized multifenestrated biliary ductal stents, which remain in place until reconstructive success is documented. Late stenosis is unlikely. Dissection away from the vasobiliary sheath in the donor has significantly decreased the incidence of this complication.
FIGURE 45-8 Segmental bile duct stricture at the junction of the left lateral and left medial segmental bile ducts in a left lobe reduced-size allograft. Solid arrow, bile duct stricture; open arrow, Roux-en-Y loop and bile duct anastomosis. (From Ryckman FC. Liver transplantation in children. In: Suchy FJ, editor. Liver Disease in Children. St. Louis: CV Mosby; 1994. p. 941.)
Acute Cellular Rejection
Allograft rejection is characterized by the histologic triad of endothelialitis, portal triad lymphocyte infiltration with bile duct injury, and hepatic parenchymal cell damage.70 Allograft biopsy is essential to establish the diagnosis before treatment. The rapidity of the rejection process and its response to therapy dictates the intensity and duration of antirejection treatment.
Acute rejection occurs in approximately two-thirds of patients following LT.71 The primary treatment is a short course of high-dose steroids. Bolus doses administered over several days with a rapid taper to baseline therapy is successful in 80% of cases.72 When refractory or recurrent rejection occurs, antilymphocyte therapy using the monoclonal antibody OKT-3® or thymoglobulin® is successful in 90% of cases.38
Chronic Rejection
Uniform diagnosis and management of chronic rejection is complicated by the lack of a consistent definition or clinical course. Chronic rejection occurs in 5–10% of transplanted patients. Its incidence appears to be decreasing in all transplant groups, perhaps related to better overall immunosuppressive strategies. There is some suggestion that the use of tacrolimus based immunosuppression is a key element in this apparent decrease.73,74 Risk factors for its development are many, and no factor predicts the outcome of treatment. The chronic rejection rate is significantly lower in recipients of LD grafts compared with cadaveric grafts.75 In that study, African-American recipients had a significantly higher rate of chronic rejection than did Caucasian recipients. In addition, the number of acute rejection episodes, transplantation for autoimmune disease, occurrence of post-transplant lymphoproliferative disease (PTLD), and cytomegalovirus (CMV) infection were also significant risk factors for chronic rejection. The primary clinical manifestation is a progressive increase in biliary ductal enzymes (alkaline phosphatase, GGT) and progressive cholestasis. Chronic rejection can be initially asymptomatic or may follow unsuccessful treatment for acute rejection. It can occur within weeks of transplantation or later in the postoperative course.
Chronic rejection usually follows one of two clinical forms.76 In the first, the injury is primarily to the biliary epithelium and the clinical course is slowly progressive with preservation of synthetic function. Histologically, either interlobular bile duct destruction in the absence of ischemic injury or hepatocellular necrosis is seen. In full expression, this form is characterized as acute vanishing bile duct syndrome when severe ductopenia is seen in at least 20 portal tracts.77,78 The eventual spontaneous resolution in up to one-half of affected patients with tacrolimus therapy has led to the development of enhanced immunosuppression protocols for this patient subgroup.76 Retransplantation is occasionally necessary, but it is rarely needed emergently.
The second subtype is characterized by the early development of progressive ischemic injury to both bile ducts and hepatocytes, leading to ductopenia and ischemic necrosis with fibrosis. The clinical picture of cholestasis is accompanied by significant synthetic dysfunction with superimposed vascular thrombosis or biliary stricture formation. The vascular endothelial injury responsible for the progressive ischemic changes is characterized by the development of subintimal foam cells or fibrointimal hypertrophy. The clinical course is relentlessly progressive, and nearly always requires retransplantation. Unfortunately, recurrence of chronic rejection in the retransplanted allograft is common.77
Recent studies have focused on donor specific antibody mediated abnormalities that may be the pathophysiologic basis for chronic rejection.79
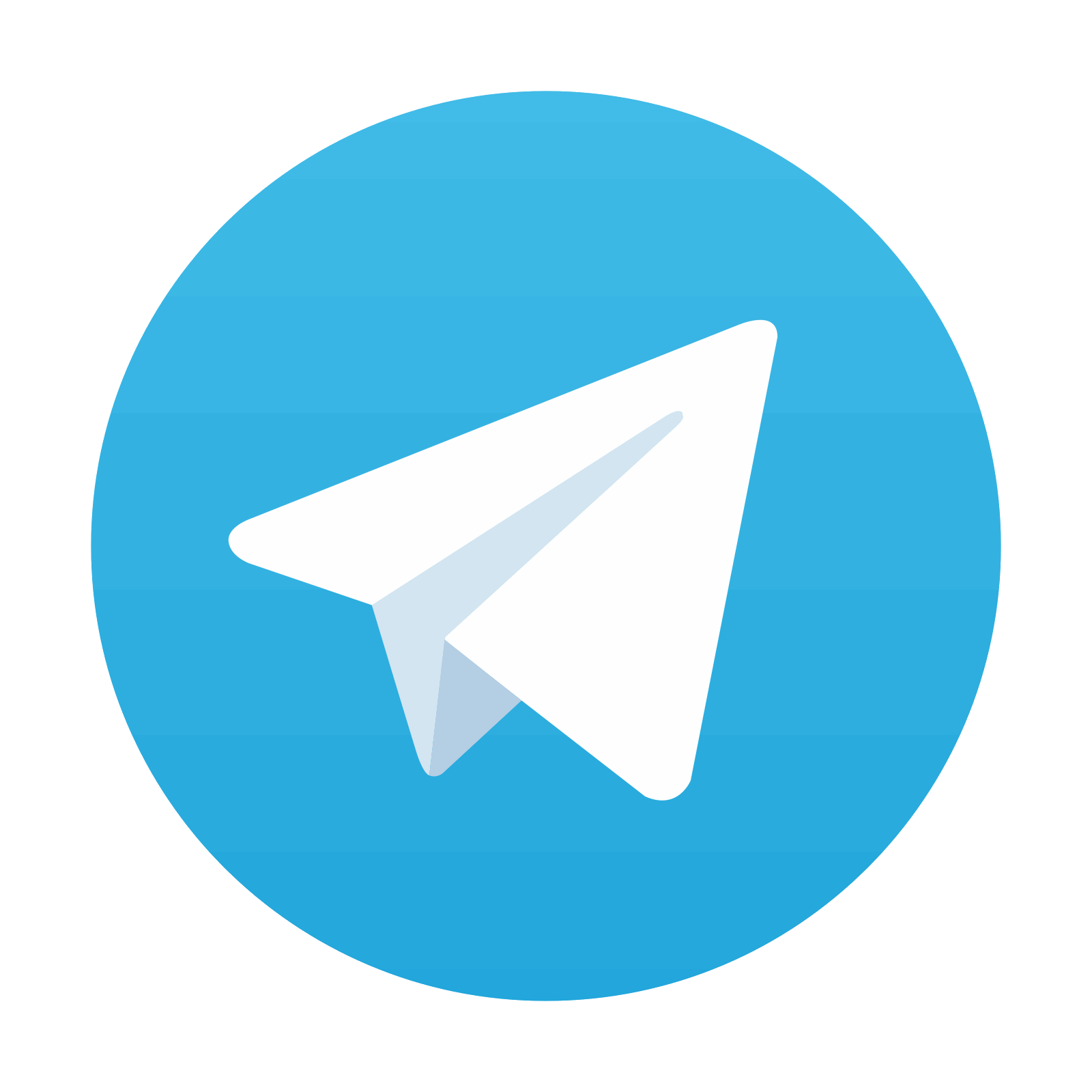
Stay updated, free articles. Join our Telegram channel

Full access? Get Clinical Tree
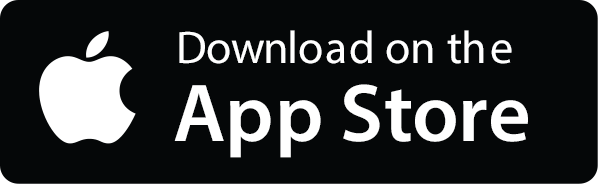
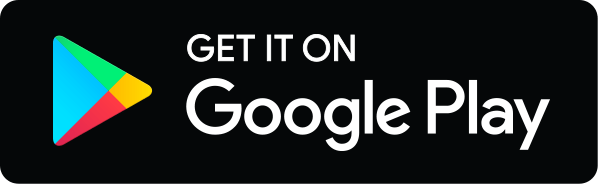