Sleep during Adolescence
Introduction
Sleep–wake behavior changes across adolescence are the product of changing intrinsic regulatory sleep mechanisms and an evolving psychosocial milieu.1 Often, these changing biological processes regulating sleep are in direct conflict with social demands, creating a state of chronically restricted and ill-timed sleep. A description of these sleep–wake patterns begins the chapter. To understand how these patterns emerge, the intrinsic regulatory mechanisms of sleep and wake – the homeostatic sleep system and the circadian timing system – and our current understanding of how they change across adolescent development are reviewed next. The chapter then identifies some of the salient psychosocial factors that likely contribute to adolescent sleep–wake behavior and how they interact with maturational changes in sleep-regulatory processes to modify sleep patterns.
Sleep–Wake Patterns
A hallmark behavioral change of adolescence is the tendency for sleep timing to become later. This delay of sleep patterns has been reported across the globe in pre-industrial and industrial countries.2 To start, many adolescents report going to bed later as they get older, especially on weekend and vacation nights. Self-reported school-night bedtimes in the US range from about 9:30 p.m. to 11:30 p.m.,3 and reported bedtimes are as late as midnight or 1:00 a.m. in European and Asian samples.4 Older adolescents (ages 14–19 years) typically report later bedtimes than their younger peers (ages 11–13 years).3,4 Wake-up times on school days remain relatively stable or get earlier across these age groups because they are dictated by school start times. The majority of US schools begin before 8:15 a.m., and many start before 7:30 a.m.5 School-day wake times range from about 6:00 a.m. to 7:00 a.m.,3,4 with some reports noting a tendency for older adolescent girls to wake earlier than boys.e.g.,6
Late bedtimes and early wake times on school nights reduce sleep opportunity time for the average teen. The range of self-reported time in bed is about 6.5 to 8.5 or 9 hours, with older adolescents usually reporting less time in bed than younger adolescents.4,7 The majority of high-school-aged adolescents (≈14–18 years) report 8 h of sleep or less, and Korean adolescents average closer to 5.5 to 6.5 hours of time in bed on school nights.8,9 Using actigraphy and self-reported pubertal status in a longitudinal design, Sadeh and colleagues10 reported that delayed sleep onset and a shortening in sleep duration emerges before the manifestation of secondary sexual characteristics associated with puberty. Later sleep onset and shorter total sleep time also predicted a faster progression of self-rated puberty status. Thus, the onset of puberty in this study was linked to the behavioral sleep changes observed during adolescence.
Despite this decreased time devoted to sleep on school nights, however, laboratory studies reveal that more mature adolescents may ‘need’ the same amount of sleep, and perhaps more compared to their younger and less mature peers. Carskadon and colleagues11 studied youngsters aged 10 to 12 years old longitudinally across three consecutive summers. Participants were given 10-hour nocturnal sleep opportunities (10 p.m.–8 a.m.) for 1 week before and then while in the laboratory for three consecutive nights. Polysomnographic (PSG) recordings revealed that the amount of time spent asleep during these 10 hours remained constant across puberty stages (Tanner stagesa 1 through 5), and averaged 9.2 hours. Furthermore, the mature adolescents were often awakened at the end of the 10-hour sleep opportunity, suggesting that these teenagers may have slept longer if permitted. The disparity between this estimate of sleep ‘need’ and reported school-night sleep duration suggests that most adolescents, especially older and more mature adolescents, are severely sleep-restricted, and are likely carrying residual sleep pressure during the school week.
It remains unclear whether the sleep loss accumulated over the school week can be ‘recovered’ on weekends. Many teenagers, however, exhibit behavior similar to a ‘behavioral sleep rebound’ on weekend nights, which may indicate that adolescents try to compensate for insufficient school-week sleep by sleeping more on non-school nights. Weekend time in bed averages about 1 to 1.5 hours more on weekend nights compared to school nights, and this disparity in sleep duration increases with age.4,13 Indeed, preteens and younger adolescents may show no weekend rebound. Furthermore, a majority of mid to older adolescents typically go to sleep about 1 to 2 hours later on weekends compared to school-nights and extend their sleep by waking 1 to as many as 4 hours later on weekend mornings.3 Self-reported average bedtime typically ranges from about 10:00 p.m. to as late as 2:00 a.m. on weekend nights, and weekend wake times typically range between 8:30 a.m. and 10 a.m. Standard deviations of an hour or more in some studies suggest that many teens sleep later than this average on weekend mornings. Again, older adolescents report later weekend bedtimes and wake times than their younger adolescent peers, on average.3,4 Changes to sleep–wake timing on weekends compared to school-nights results in sleep irregularity for many adolescents.
Sleep–Wake Regulation
Borbély14 was the first to describe a model clearly identifying the interaction between sleep–wake homeostasis and the circadian timing system. In his ‘Two-Process Model of Sleep Regulation,’ he designated the homeostatic sleep–wake component as Process S and the circadian component as Process C. Since its original description, this model has been refined and variations have been developed,15–20 and these models now guide our understanding of developmental changes to sleep timing and duration across adolescence.
Homeostatic Sleep–Wake System
A simple way to characterize the homeostatic sleep–wake process is that the ‘pressure’ to sleep increases the longer an individual is awake and dissipates as the individual sleeps. Physiological correlates of this process have been quantified using the sleep EEG. For example, EEG slow-wave activity, or SWA (power in the 0.75–4.5 Hz range) is a useful marker of ‘sleep pressure.’ SWA predominates at the beginning of the nocturnal sleep episode when sleep pressure is greatest and shows a decline across the night as sleep pressure dissipates.14,18,21,22 The SWA decline over the course of the night can be fit with an exponential decaying function, from which the time constant of the decline gives a metric of the rate of dissipation of sleep pressure. If the system is challenged by extending wakefulness, SWA increases during subsequent sleep episodes, and this increase is proportional to the time spent awake.21 The build-up of SWA is modeled using an exponential increasing function. The specific neuroanatomical and neurochemical factors involved in sleep–wake homeostasis are not fully understood.
Circadian Timing System
In contrast to the homeostatic sleep/wake system, the circadian system does not depend on prior sleep and wake duration, but is rather a self-sustaining system that intrinsically regulates a multitude of 24-hour rhythms, including sleep propensity. The ‘master clock’ that organizes the timing of these rhythms in mammals has been localized to the suprachiasmatic nuclei (SCN) of the hypothalamus.23
Physiological or behavioral outputs of systems regulated by the SCN can be measured over extended periods of time (hours to days), and events associated with these approximate 24-hour rhythms (e.g., when a hormone ‘turns on’ or ‘turns off’) are used to infer circadian time or phase. For example, melatonin, a hormone secreted by the human pineal gland, is regulated by the SCN and therefore oscillates with a circadian rhythm. Levels of the hormone are nearly absent during the day, rise in the evening, stay relatively constant during the night, and decline close to habitual wake-up time. The onset of melatonin secretion, also called the dim lightb melatonin onset (DLMO),24 is the most common and reliable marker of the human circadian timing system.25–27 The decline of melatonin, also called the dim light melatonin offset (DLMOff) phase is another phase marker of the circadian timing system derived from the melatonin rhythm (see also Chapter 5).
Circadian rhythms oscillate with an intrinsic period slightly different from 24 hours. In adults, this period ranges from 23.5 to 24.9 hours,28,29 and one analysis of over 150 adults reported an average period of 24.15 ± 0.2 hours.28 The majority of people have an endogenous period longer than 24 hours; only an estimated 21% have an intrinsic period of less than 24 hours.30 Sex30 and race29 differences in endogenous period have also been reported in adults. The circadian timing system is capable of entrainment, the process by which the endogenous period is synchronized to the environmental 24-hour day using external time-givers, or zeitgebers. The primary entraining stimulus to the system is light and dark,31,32 and the system shows some sensitivity to short-wavelength (≈460 nm) light.33,34 A small subset of intrinsically photosensitive retinal ganglion cells (ipRGCs) containing melanopsin and projecting to the SCN have been described in rodents.35–37 The small percentage of ipRGCs that contain melanopsin are photosensitive,38 are modulated by synaptic input from rods and cones,39 and are necessary for circadian entrainment by light.40 Light–dark cues come from the daily cycles of daylight and darkness and can also be artificially imposed in modern environments by switching an electric light on and off or by drawing a window shade.
One proposed entrainment mechanism (‘discrete entrainment’41) describes the process as a daily phase shift in response to photic cues that corrects the difference between the endogenous period and the external solar day length. This entrainment mechanism is predicted by a phase response curve (PRC) to light. Light PRCs are experimentally derived and describe how light shifts circadian rhythms to an earlier or later time when light is presented at various times across the circadian cycle. In general, the human system responds in a systematic and predictable manner: light just before habitual bedtime or in the first half of habitual sleep shifts circadian rhythms later (phase delay), while light during the second half of habitual sleep or just after waking shifts circadian rhythms earlier (phase advance).42–47 According to these general properties of the adult light PRC, morning light exposure facilitates entrainment in those with an intrinsic period greater than 24 hours, whereas evening light exposure entrains individuals with a period shorter than 24 hours. In adults, endogenous circadian period also predicts differences in how the system aligns to sleep,48 and those with a long period have a later entrained phase (clock time) than those with a short period.49
Two-Process Model of Sleep Regulation
Figure 6-1 is a schematic representation of how Process S and Process C may interact as opponent processes, and is based on the models proposed by others.19,20,50 The broad concept of ‘sleep pressure’ is on the y-axis as a function of time on the x-axis. Sleep pressure varies across the 24-hour day (Process C, red line), and also depends on the amount of time awake and asleep (Process S, blue line). In this schematic example, sleep occurs from 10 p.m. to 7 a.m. The onset of melatonin production (DLMO phase) usually occurs before habitual bedtime and is illustrated by the upward-facing arrow at 9 p.m. Process S is anchored to the onset of sleep and the onset of wake. The homeostatically driven pressure to sleep decreases as sleep progresses across a night of sleep and increases with hours of wakefulness across the day. Sleep pressure dictated by the circadian timing system is greatest about 7 hours after DLMO phase, near the end of habitual sleep and is lowest just before DLMO phase near to habitual bedtime. According to this two-process model, the alerting signal of the circadian process opposes a wake-dependent sleep-promoting process to maintain wakefulness across the day.19 Similarly, homeostatic sleep pressure dissipating across a night of sleep is countered by the circadian timing system as it reaches its maximal levels of sleep propensity during the second half of the habitual sleep period, thus maintaining or ‘protecting’ the second half of nocturnal sleep.20 Theoretically, the alignment of these two processes predicts maintenance of wakefulness and alertness during the daytime and maintenance of sleep at night. This ideal balance between the systems is challenged during adolescence as both processes show developmental changes and as behavioral demands and choices alter the balance.
Figure 6-1 A schematic representation of the Two Process Model of Sleep Regulation.
The broad concept of ‘sleep pressure’ is illustrated on the y-axis as a function of time spanning over 2 days. An ideal sleep duration of 9 hours per night from 10 p.m. to 7 a.m. is illustrated by shaded gray rectangles. The upward-facing arrow at 9 p.m. illustrates the time of melatonin onset (DLMO phase), which usually occurs 1 to 2 hours before bedtime. Process C (red curve) shows sleep pressure changing with a cycle length of 24 hours. The pressure to sleep is highest approximately 7 hours after DLMO phase and sleep pressure is lowest just before DLMO phase, and thus just before bedtime. Process S is illustrated by the blue curve, and is dependent on the timing and duration of sleep and wake. At sleep onset, sleep pressure is highest and dissipates over the course of sleep, whereas sleep pressure is lowest at the beginning of the waking day and accumulates over the course of wakefulness. Ideally, these two processes interact with one another to maintain sleep at night and maintain alertness during the day (see text). This figure was published in S.J. Crowley, C.N. Kyriakos, and A.R. Wolfson. Sleep Patterns and Challenges. In: Encyclopedia of Adolescence. B.B. Brown and M. Prinstein (eds.). Copyright Elsevier (2011).
Changes to Sleep–Wake Regulation Across Adolescence
One of the most readily observed and striking changes to the sleep EEG is a progressive developmental decline in the amplitude of the signal across adolescence. This decline typically begins around ages 9 or 10 and continues until the early 20s, though the precise timing of the beginning and termination of this decline varies across individuals and is debated.51,52 This decline likely reflects the pruning of synapses that occurs in the healthy adolescent cortex53,54 and is not state-specific. As a result, EEG power is diminished during waking55–57 and sleep.58–63
One sleep-specific change to the EEG is a redistribution of sleep-stage variables. Minutes of slow-wave sleep (SWS; sleep characterized by high-amplitude, low-frequency waves) declines by approximately 40%.60,61,64–67 At the same time, an approximate 20% increase in stage 2 sleep is observed in both longitudinal60 and cross-sectional61 samples. Whether these changes are also a reflection of adolescent cortical restructuring or are of functional significance to sleep-dependent processes remains unknown. Finally, young adolescents tend to ‘skip’ their first REM episode.60,66 The ‘skipped REM’ episodes are characterized by the emergence from ‘deep’ sleep stages 3 and 4 (SWS) into ‘lighter’ stages such as stages 1 and 2 for a duration of at least 12 minutes.61 In adults, ‘skipped REM’ episodes often accompany sleep deprivation protocols; therefore, some have speculated that the skipped REM episodes in young individuals may reflect increased sleep drive observed in younger individuals.68
The dynamics of the homeostatic system are also altered during puberty, though not involving the dissipation of Process S with sleep. One cross-sectional and two longitudinal studies which modeled the dissipation of sleep pressure across nights of sleep found that the time constant of the decay does not change across adolescent development.69–71 This finding lends further support to the notion that the recovery process occurring during sleep, from which some infer sleep ‘need,’ does not change across adolescent development. One cross-sectional study modeled the build-up of SWA using sleep before and after 36 hours of sleep deprivation and found an increase in the time constant of the build-up in post-pubertal versus pre-pubertal teens.70 In other words, mature adolescents accumulated sleep pressure at a slower rate across a waking period compared to their younger pre-pubertal peers. Longer sleep onset latency near bedtime in Tanner 5 adolescents compared to Tanner 1 adolescents provides further support for this developmental difference in sleep pressure at the end of the waking day.72 Figure 6-2 illustrates this relative change across adolescence. Process S of the mature adolescent (blue dashed line) increases across the waking day at a slower rate than the immature, young adolescent (blue solid line), and thus at a 10 p.m. bedtime has not reached the same level of sleep pressure as the immature adolescent. This slower accumulation of sleep pressure is thought to explain, in part, why mature adolescents are able to stay awake longer into the evening or night, whereas younger pre-pubertal adolescents are able to, and often do, fall asleep more rapidly and earlier in the evening.
Figure 6-2 A Schematic Representation Illustrating the Relative Change to Process C and Process S with Maturation.
Illustration symbols are the same as in Figure 6.1. Young, immature adolescents are shown in solid lines and mature, older adolescents are shown in dashed lines. The dissipation of Process S is shown as a solid blue line for both immature and mature adolescents since this recovery process shows little change across adolescence. A delayed Process C and a slower accumulation of Process S are permissive of later sleep times with maturation (see text).
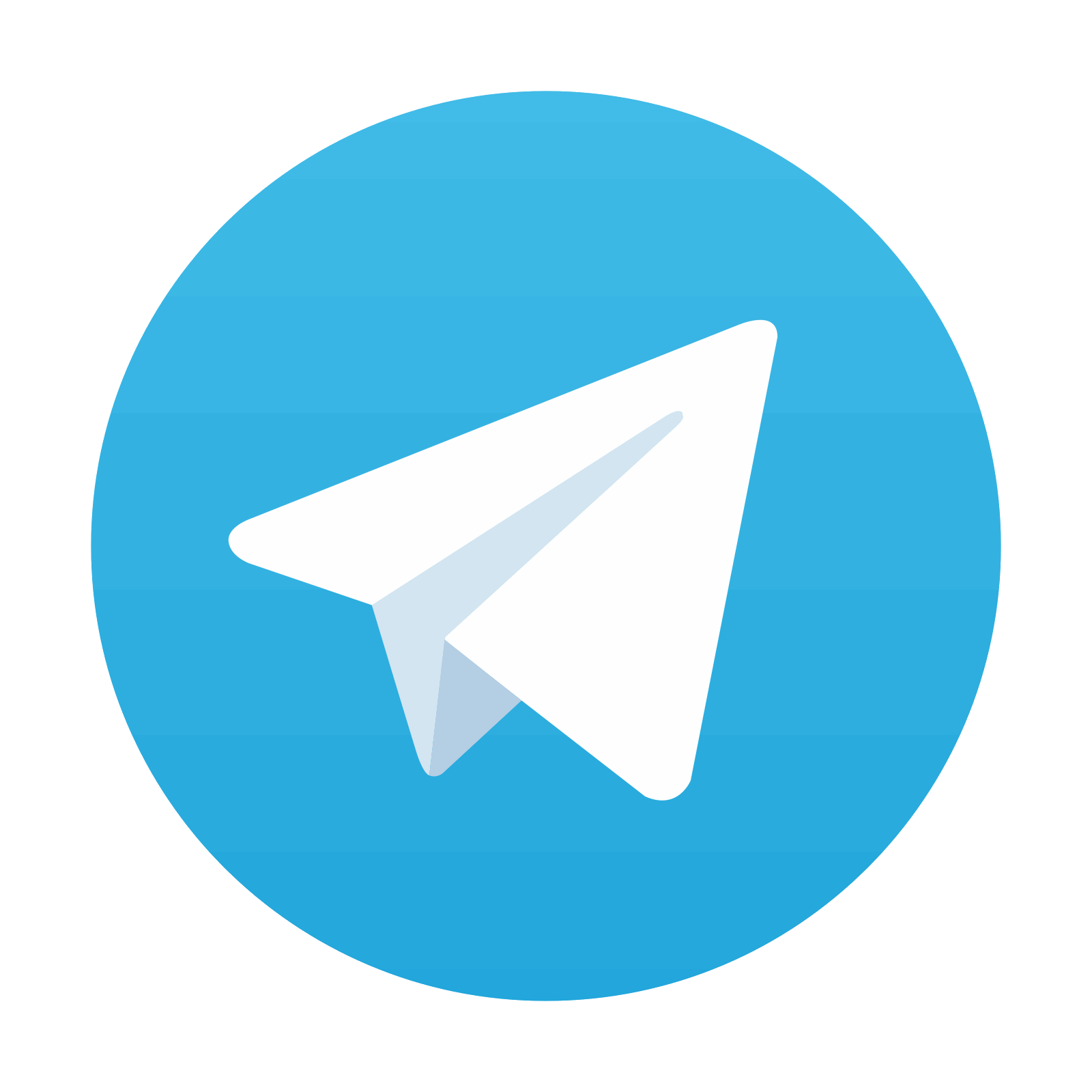
Stay updated, free articles. Join our Telegram channel

Full access? Get Clinical Tree
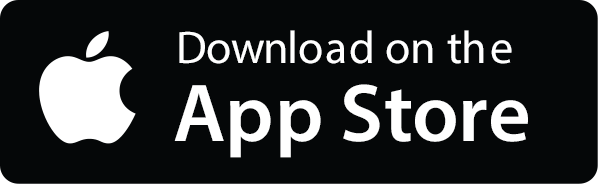
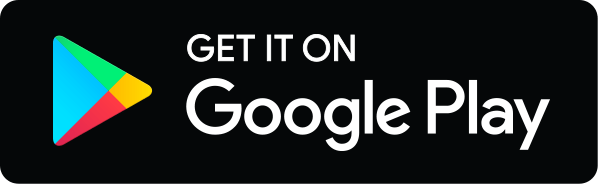