Distal femoral physeal fractures in children may be difficult to treat and are associated with a high rate of complications including growth arrest.1 Close follow-up is necessary to detect early angular deformity that may result from a partial growth arrest. The greater the severity of fracture and displacement across the physis, the higher the risk of growth arrest.2 There should be a low threshold for obtaining computed tomography (CT) or magnetic resonance imaging (MRI) of the affected physis when a bony bridge is suspected in order to allow early intervention.
Anatomy
The distal femur is the most rapidly growing physis in the human body and the first epiphysis to ossify. In a full-term infant, the distal femoral ossification center is usually present at birth. The distal femur contributes approximately 70% of the growth of the femur and 40% of the overall limb.3,4 The distal femur’s average growth is 1 cm a year until closure between 14 and 16 years of age in girls and 16 to 18 years of age in boys.5
The majority of the distal femoral epiphysis is covered by the articular cartilage and articulates with the patella and proximal tibia. The distal femoral physis is an extra-articular structure; the capsule originates distal to the physis from the epiphysis. The undulating contour of the physis may be more resistant to shear forces; however, when shearing injury does occur, there is greater risk of growth arrest. Liu et al.6 described the lateral and anteromedial peripheral notches located at the metaphyseal–epiphyseal junction of the distal femur. Additionally, three major undulations within the epiphysis were identified across cadaveric specimens. These ridges were identified centrally, laterally, and medially and found to decrease with age especially in the central ridge.
The stabilizing medial and lateral collateral ligaments originate from the distal femoral epiphysis. The gastrocnemius takes its origin from the posterior surface of the epiphysis. Whereas in adults the ligaments may be injured due to varus and valgus stress on the knee, in skeletally immature patients, the distal femoral physis is more commonly injured.
Multiple neurovascular structures are at risk with injury to the distal femur. Most vulnerable are the popliteal artery and peroneal and posterior tibial nerves. The popliteal artery may be injured by the metaphyseal spike of distal femoral fracture. This vulnerability is in part due to the tethering of the artery above at the adductor hiatus and distally by the fibrous tissue over the soleus muscle. Injuries may lead to thrombosis or bleeding from intimal tears. Displaced physeal fractures also have a risk of compartment syndrome, as arterial injury is more likely to occur. In a series of distal femoral physeal fractures, compartment syndrome occurred in 1.3% of patients.7 Injury to nervous structures may also occur with displacement. The posterior tibial and peroneal nerves form after the sciatic nerve branches in the popliteal space. Both are subject to traction injuries with displaced fractures. The incidence of peroneal nerve injuries was 7.3% in the same clinical series.7
Classification
The Salter-Harris classification is commonly used and easily applied to these fractures. This classification can help dictate treatment as well as predict outcomes and rate of complications associated with individual fracture. The classification for distal femur fractures can be seen in Figure 34.1 and is defined as follows8:
A Salter-Harris I fracture is defined as a separation through the distal femoral physis with no involvement of either the metaphysis or the epiphysis. Salter-Harris II fractures are a separation through the distal femoral physis fracture exiting through the metaphysis. The resulting metaphyseal fragment is called the Thurston-Holland fragment. The portion of the physis that is “unprotected” by the Thurston-Holland fragment is at risk of growth arrest.
A Salter-Harris III is a physeal fracture in which the fracture line exits through the epiphysis and into the knee joint. Fractures of the medial femoral condyle that originate at the medial physis and exit into the notch have been compared to transitional fractures of the ankle given their relation to physeal closure.9 Coronal shear fractures of the lateral condyles have also been described.10 Salter-Harris IV injuries originate in the physis and the fracture exits through both the metaphysis (with a Thurston-Holland fragment) and the epiphysis. Salter-Harris V injuries are a crush injury to the physis and are unable to be definitively identified until resulting growth arrest manifests.
Epidemiology
Classification and displacement of distal femur physeal fractures has been shown to be predictive of overall outcome.1 Overall, these fractures account for between 1.4% and 5.5% of all physeal injuries in children.11–13 Of all fractures in children, these represent little more than 1%.11
Mechanism of Injury
Epidemiologic studies have shown that when physeal fractures of the distal femur occur in younger children, they are usually due to severe trauma or a high-energy injury.13 However, Salter-Harris I and II fractures in older children and adolescents are generally related to lower energy injuries or sports-related activities.2 For Salter-Harris I and II injuries, the knee is generally in a position of hyperextension with or without varus or valgus strain (Fig. 34.2).5 Patients will usually present complaints of significant knee pain and have associated knee swelling.
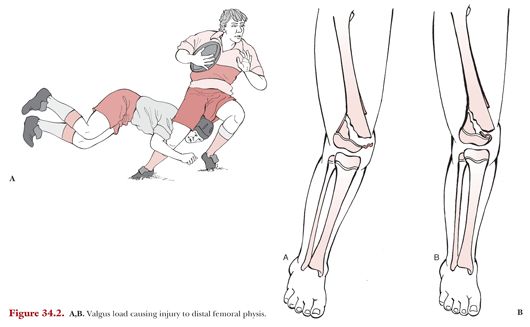
A Salter-Harris II fracture is more likely when both compression and distraction forces occur across the knee. This simultaneously creates tension and compression sides to the fracture and metaphyseal failure occurs due to compressive shear.14
Salter-Harris type III fractures are relatively rare injuries compared with the more common Salter-Harris I and II. They can lead to poor outcomes but have similar mechanisms of injury. Fractures of the medial femoral condyle result from a valgus force on the knee. These fractures will demonstrate valgus laxity on presenting exam; this has been shown to resolve after fracture healing.9 Coronal fractures involving the lateral femoral condyles result from a shear-type mechanism. The fracture types with an epiphyseal component such as a Salter-Harris III usually result in a larger effusion than types I and II injuries. Sports-related twisting or high-energy motor vehicle trauma can be the cause of a Salter-Harris IV. Similar to a Salter-Harris III, the patient will likely have a knee effusion due to the intra-articular component. The mechanism of a Salter-Harris V is unclear, but trauma causes a crush injury to the physis.
Diagnosis
For Salter-Harris I and II fractures that are nondisplaced, patients will present with localized tenderness to palpation at the level of the growth plate. An anatomic reference is that in the extended knee, the equator of the patella generally aligns with the physis unless the patient has significant patella baja or alta.15 Given the extra-articular nature of this fracture, patients may have swelling but not a joint effusion. This contrasts with Salter-Harris III and IV fractures in which there will generally be an effusion. For all these fractures, range of motion as well as any varus or valgus stress will likely elicit pain. Pain with weight bearing will also be seen. Displaced fractures will have a more dramatic presentation with potential gross deformity of the knee in the direction of displacement.
Imaging
Careful inspection of the physis on plain radiographs is necessary in order to identify minimally displaced fractures. At presentation, Salter-Harris I radiographs may demonstrate a widening or irregularity of the physis (Fig. 34.3). Sometimes a small fleck of bone may be evident adjacent to the physis. In nondisplaced fractures, the only sign of fracture may be periosteal reaction 2 to 3 weeks after the injury. Plain radiographs including anteroposterior, lateral, and oblique views of the knee are usually adequate for diagnosis. Although historically stress radiographs were used to document Salter-Harris I fractures, this has largely been abandoned. The impetus behind this shift in thinking is that given the nonoperative management of these injuries, the risk of stressing the physis and potentially causing additional injury to an already damaged physis is not indicated.16 MRI has largely replaced stress radiographs and should be considered in any case of knee trauma, pain and swelling, and negative plain radiographs in order to confirm the diagnosis of a nondisplaced Salter-Harris I fracture.
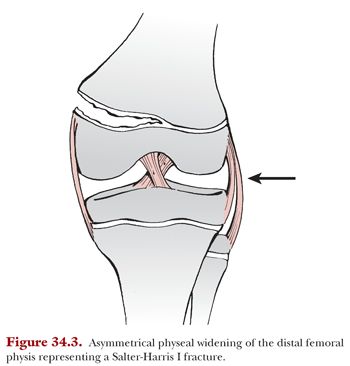
For Salter-Harris II fractures, it is important to identify the metaphyseal component of these injuries, the size of which may influence surgical planning. MRI has been used to identify the frequency of posterior periosteal disruption in seemingly mild injuries. This posterior periosteal disruption was associated with a hyperextension mechanism of injury.17
Plain radiographs may not be sufficient for Salter-Harris III or IV fractures due to their intra-articular nature. The overlying patella may hide the epiphyseal component of the fracture, the displacement of which may not be adequately visualized on lateral imaging. Oblique radiographs will improve the sensitivity of assessing these fractures; however, radiographs have been shown to significantly underestimate displacement of Salter-Harris III fractures.18,19 To most accurately determine the amount of articular step-off, a CT scan or MRI should be obtained. Advanced imaging has been shown to change surgical plan and is important given that many of these minimally displaced injuries are initially missed.15
Salter-Harris V fractures, as noted, are difficult to diagnose on imaging at time of initial presentation. These injuries are distinguished from their nondisplaced Salter-Harris I counterparts only in hindsight with growth arrest. It has been suggested that blurring of the bone structure around the physis may demonstrate a Salter-Harris V.20 MRI may be helpful to identify physeal injury in the setting of negative plain films at the time of injury.
Treatment
Nondisplaced fractures should be managed with a long leg cast typically for 4 to 6 weeks. In patients with obese body habitus not amenable to long leg casting, and in very young patients, spica casting may be necessary.15 Serial radiographs are important in order to identify displacement in a timely manner.
Displaced fractures must undergo gentle closed reduction under sedation. Reduction should be performed with longitudinal traction to minimize damage to the physis. Once anatomic reduction is achieved, it may be held with smooth crossed Kirschner wires (Fig. 34.4). Pins are typically placed in an antegrade manner from metaphysis across the physis into the epiphysis then bent to prevent pin migration. Avoiding entering through the joint also minimizes the risk of associated septic arthritis. Stability of fracture fixation should be confirmed through range of motion under fluoroscopy. Postoperatively, patients should be immobilized in a long leg cast until pin removal at 4 weeks. Two additional weeks of casting is generally prudent until allowing ambulation; however, this may vary based on age and healing potential.
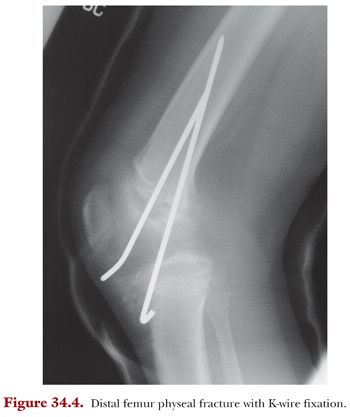
Salter-Harris II treatment varies based on the size of the metaphyseal fragment. Nondisplaced fractures may be treated in a cast but must be followed closely to assess any interval displacement. Reduction should only be performed up to 7 to 10 days after injury in order to avoid risk of further traumatic injury to the healing physis. Displaced Salter-Harris II fractures warrant closed or open reduction and pinning. If there is a large metaphyseal component, this may be transfixed, ideally, with two cannulated screws (Fig. 34.5). Transverse metaphyseal fixation with screws avoids crossing the physis and causing further injury to the traumatized growth plate. Postoperatively, patients should still be immobilized in a long leg cast in 20 to 30 degrees of flexion. In cases of significant soft tissue swelling and concern for a compartment syndrome or when arterial injury has occurred, apply a well-padded bivalved cast after definitive fixation.
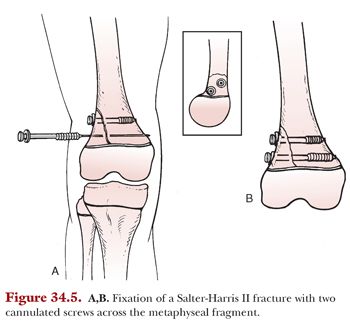
The majority of Salter-Harris III fractures need reduction and fixation due to intra-articular nature of this fracture (see Fig. 34.1). Lippert et al.18 asserted that if the fracture line is visible on x-ray, then it should be managed with open reduction internal fixation. This is due to the inherent instability of this fracture pattern, the intra-articular nature of the fracture, and the risk of growth arrest due to bony bridge formation without appropriate reduction and fixation. Anatomic reduction is essential, and only truly nondisplaced fractures as confirmed by axial imaging are amenable to cast immobilization.
As with Salter-Harris III fractures, Salter-Harris IV fractures should be managed with open reduction internal fixation in order to assess anatomic reduction of the epiphyseal component. When placing the transverse epiphyseal screw for fixation of the epiphyseal component, Wall and May15 described a target triangle bordered by the growth plate, the intercondylar notch, and the trochlear groove for appropriate screw placement. It is important to avoid overpenetration of the screw tip which will cause soft tissue irritation over time.
Salter-Harris V fractures are managed nonoperatively, as the initial diagnosis is usually a nondisplaced Salter-Harris I unless abnormal physeal findings are noted and suspicion arises. The described treatment for these fractures is largely the treatment of the ultimate growth arrest as a result of the injury as opposed to the fracture itself. Skak20 described the management of a 10-year-old female with a Salter-Harris V of the lateral distal femur that went on to growth arrest and a valgus deformity.
Outcomes
Growth disturbance is a common sequelae of distal femoral physeal fractures. Fractures that are displaced more than half of the diameter of the shaft are at greater risk for growth arrest.21,22 In a meta-analysis of distal physeal fractures, growth disturbance was found in 36% of Salter-Harris I fractures.23 It is important to follow the patient both radiographically and clinically to assess for growth arrest at 6 months intervals after injury. Any varus or valgus deformity may result due to medial or lateral growth arrest. On clinical exam, a knee flexion deformity may be a result of a bony deformity due to posterior growth arrest.15 Pelvis obliquity and leg lengths on examination are also important to follow.
In a meta-analysis of distal physeal fractures, growth disturbance was found in 58% of Salter-Harris II, 49% of Salter-Harris III, and 64% of Salter-Harris IV fractures.23 A retrospective study with 4-year follow-up of patients with Salter-Harris II injuries showed no complications for the nondisplaced injuries managed conservatively; however, there was a 70% complication rate for displaced fractures reduced under general anesthesia.24
Salter-Harris III and IV fractures can lead to poor outcomes; the literature has described complications including limb length discrepancy, angular deformity, knee flexion deformity, and posttraumatic arthritis. The outcomes for these intra-articular fractures are largely based on the degree of postoperative displacement, with poorer outcomes seen with greater displacement. Given the intra-articular nature of the injury, patients are at higher risk for posttraumatic osteoarthritis.
Patients who develop either limb length discrepancy or angular deformity after physeal fractures as a result of growth arrest may need secondary operative intervention. Although limb length discrepancies less than 2 cm are generally well tolerated, larger discrepancies may require contralateral epiphysiodesis or limb lengthening. Angular deformity due to partial growth arrest may be corrected by guided growth if there is sufficient growth remaining.
Fractures of the proximal tibial physis are rare and mechanism of injury can vary widely. For these reasons, evaluation and treatment of these patients often requires an experienced practitioner with a high index of suspicion. Osseous injuries may involve the articular surface of the proximal tibia or may be extra-articular and involve the proximal tibial physis or metaphysis. Treatments vary based on the severity and location, and sequela may not manifest for several years after the initial injury. In this section, we specifically discuss injuries involving the proximal tibial physis.
Anatomy
The proximal tibia extends from the metaphyseal flare to the articular surface. It includes the anterior and posterior tibial spines or intercondylar eminences, which, prior to ossification, are cartilaginous structures.25 This area also includes the tibial tubercle, which develops as an anterior extension of the proximal tibial physis in utero.26 Pertinent anatomy and a discussion of injuries to these structures have been covered in Chapters 31 to 33 and an in-depth discussion of the anatomy of the physis will be covered in Chapter 37.
Tibial growth occurs primarily in the first 5 years of life and then slows until puberty when it accelerates again. The epiphyseal ossification center can be visualized on radiographs between the first and third months of life. Overall, this center accounts for 50% to 60% of the overall length of the tibia.3,4 This corresponds to approximately 16 cm in females and 18 cm in males. Closure of the growth plate occurs in a posterior to anterior direction and is complete by age 13 to 15 years in girls and 15 to 18 years in boys.27
Anatomically, the proximal tibia is surrounded by both osseous and soft tissue structures. Laterally, the fibular head and neck abut the proximal tibial epiphysis. The fibular collateral ligament, popliteus muscle and tendon, semimembranosus, medial collateral ligament, and the structures of the pes anserinus form the surrounding soft tissue envelope without direct insertion into the epiphysis.28,29
Of particular importance when considering fractures of the proximal tibia is the potential for significant vascular injury as displaced injuries correlate with knee dislocation in adult. As with the distal femur, the popliteal artery courses directly behind the proximal tibial physis before branching to anterior tibial, the posterior tibial, and the peroneal artery (Fig. 34.6). Just distal to the physis, the popliteal artery is tethered as the anterior tibial artery passes through the interosseous membrane and the tibial artery passes beneath the soleus. Posterior displacement of the tibial metaphysis at this level has been implicated in injury of the popliteal artery. Although rare, this diagnosis can be devastating and leads to an increased risk of compartment syndrome, multiple surgical procedures, permanent neurologic disability, or even amputation.30–32
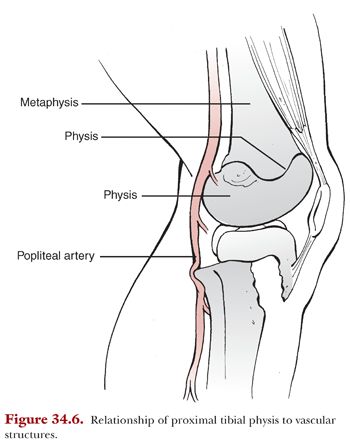
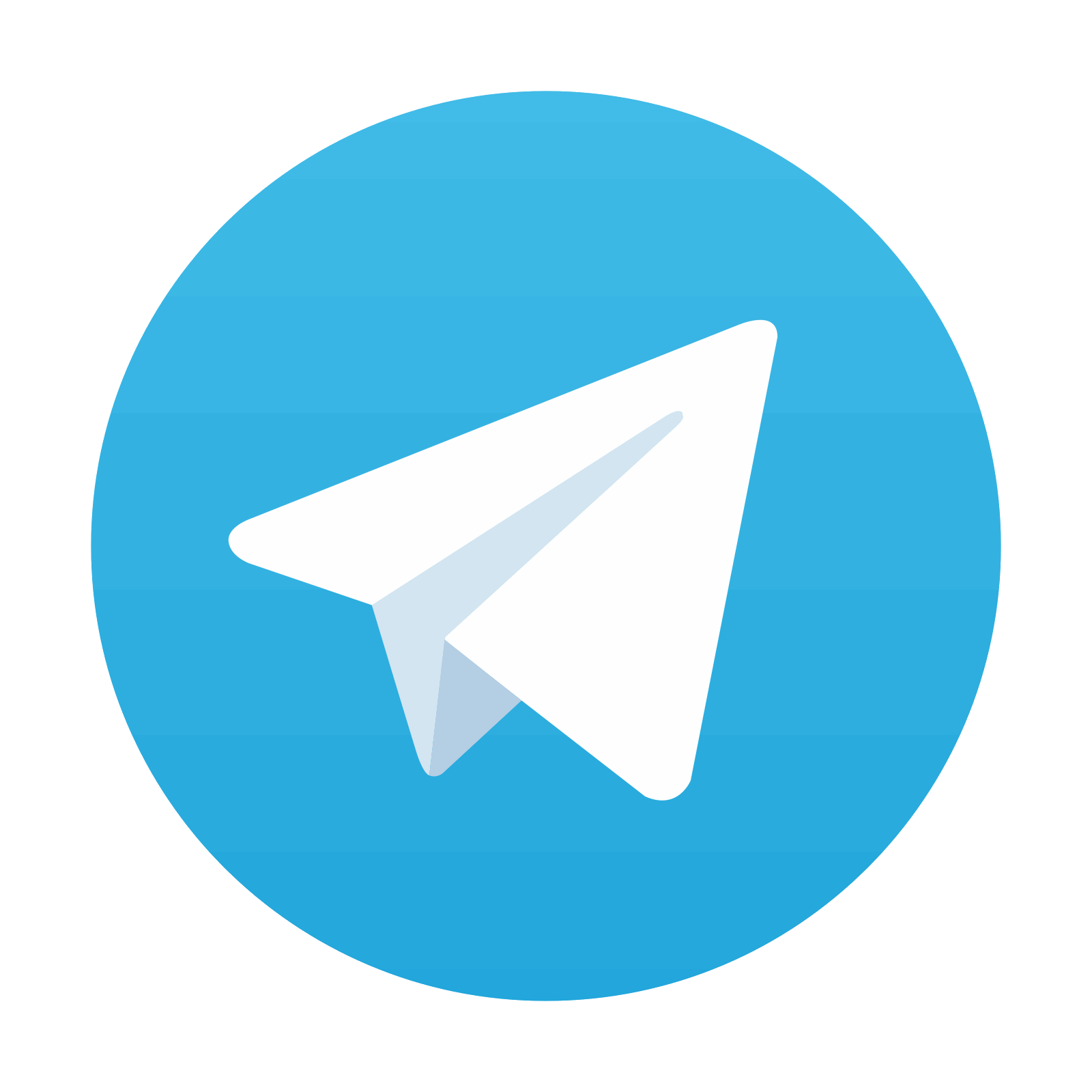