2 Safety Aspects of Doppler and Color Doppler Sonography
Doppler sonography, with its ability to define and evaluate the fetal blood supply, has been an important addition to diagnostic ultrasound in obstetrics. Because these techniques require power outputs and ultrasound intensities that are considerably higher than in B-mode imaging, the essential safety that has been established for diagnostic ultrasound in general cannot be assumed for all applications. Consequently, the sonographer should be familiar with potential bioeffects and their physical mechanisms in order to avoid any risks that may be associated with the examination.
Mechanisms of Tissue Effects
The biological effects of ultrasound are based largely on heating and cavitation. These effects are dependent on different sound field parameters and tissue properties and can therefore occur separately from each other. Other primary physical effects have no bearing on the clinical safety of ultrasound.
Heating
Ultrasound energy that is transmitted into tissue is partially reflected, partially scattered, and partially absorbed and converted to heat. The degree of heating depends on different properties of the ultrasound field and the exposed tissue. A key factor is the spatial peak time average intensity (ISPTA). In pulsed Doppler flowmetry, however, the power output of the device is a more important factor than field intensity4,23. Ultrasound frequency is also a factor, since higher frequencies are absorbed more strongly, and therefore the applied energy is concentrated over a smaller volume.
Absorption coefficient. Sound absorption in biological tissues increases with the protein content of the tissue19. Water and body fluids such as urine and amniotic fluid absorb almost no sound energy, and their absorption coefficient α is between 0.002 and 0.003 dB cm–1 MHz. Soft tissues such as brain, liver, kidney, and muscle have approximately the same absorption (α = 0.4–0.6 dB cm–1 MHz). Bone is by far the strongest absorber (α = 5–10 dB cm–1 MHz), placing it at greatest thermal risk. The secondary heating of soft tissues in close proximity to bone should also be considered when evaluating risk21,25. Acoustically, the human embryo is equivalent to soft tissue. By the end of the first trimester, however, absorption increases owing to early mineralization of the bone. Thereafter it continues to increase during the rest of the fetal period.
Heat conduction and perfusion. Heat conduction and blood flow contribute to the elimination of tissue heat. They are the major factors that determine the final temperature that is reached, especially on prolonged exposure. Whereas heat conduction can be calculated to a good approximation, the perfusion in different tissues and organs varies substantially, and so the cooling component in different tissues can only be roughly estimated. With brief ultrasound exposure, perfusion contributes little to tissue cooling5.
Hyperthermia. An increase in temperature may be a risk, since cell division is inhibited above 30°C, and temperatures higher than 41°C can cause cell death with sufficiently prolonged exposure. A key factor besides the amount and duration of tissue heating is the sensitivity of the affected tissue3. The embryo-fetal brain is extremely sensitive to temperature increases. Although brain tissue has a low absorption coefficient, secondary heating from the more strongly absorbing cranial bone must also be taken into account4. Animal experiments have shown that whole-body hyperthermia above 41°C can be teratogenic, depending on the duration of exposure11,24.
Cavitation
Noninertial and inertial cavitation. Cavitation refers to the sound-induced formation and dynamic behavior of cavities and gas bubbles, which can produce a variety of physical, chemical, and biological effects in the medium25. Two types of cavitation occur: noninertial cavitation, which describes the prolonged resonant oscillations of preexisting gas microbubbles that do not collapse; and inertial cavitation, in which preexisting microbubbles (“cavitation nuclei”) rapidly expand in the rarefaction phase of the ultrasound wave and collapse again in the subsequent compression phase. This process can generate local pressure amplitudes greater than 1000 MPa and local temperature peaks in excess of 1000°C. This may be associated with electrical arcing (“sonoluminescence”) and the formation of free radicals (OH–, H+, H2O2, etc.) with mutagenic properties that are indistinguishable from the radicals produced by ionizing radiation15,25. A continuum exists between stable and transient cavitation.
Sound-pressure amplitudes. Cavitation is a threshold effect. The critical field parameter that determines whether cavitation occur is the negative sound-pressure amplitude p– (by consensus, this amplitude is stated as a positive value in MPa). Under certain physical conditions, however, the signs of the pressure values may change when an ultrasound wave is reflected, so that the reflected wave acquires a negative pressure amplitude equal to the positive pressure amplitude of the incident wave. As a result of this, the positive pressure amplitude may also be a relevant quantity. The cavitation threshold increases at higher frequencies.
Cavitation nuclei and cavitation threshold. Human tissue is fairly resistant to cavitation because generally it does not contain cavitation nuclei. Such nuclei are presumed to exist, however, following infusions, administration of echo contrast agent, gas gangrene infections, and open injuries. The negative sound-pressure amplitude necessary for cavitation to develop is unknown. In shock-wave lithotripsy, cavitation is known to occur at negative pressures that exceed 10 MPa, but modern sonographic techniques employ fields whose negative peak pressure does not exceed 5 MPa (Fig. 2.1a). Cavitation on a large scale would seriously affect imaging because the induced bubbles would cause extreme increases in scattering and absorption. So far these effects have not been observed in ultrasound examinations. Cavitation, then, is unlikely to be a risk factor in the ultrasound techniques in current use. It should be added, however, that echo contrast agents greatly lower the threshold for cavitation (see Ultrasound Contrast Agents, p. 31).
Fig. 2.1 Minimum (min), maximum (max) and mean values of the negative peak pressure p– (a) and ISPTA intensity (b) of various European ultrasound scanners in various modes. The corresponding values of therapeutic and lithotripsy devices are also shown for comparison. Imag: B-mode imaging; VagM: M-mode with a vaginal probe; M: conventional M-mode; Col: color Doppler; CW: continuous-wave Doppler; PD: pulsed Doppler; Ther: physiotherapy; Litho: lithotripsy. (After reference 6.)
Risk Assessment of Various Ultrasound Techniques
The risks associated with various ultrasound techniques depend chiefly on the likelihood of a biologically significant temperature rise and the potential for inducing cavitation. The field parameters of current diagnostic instruments that have a bearing on these effects are reviewed in Fig. 2.1. Recent measurements have essentially confirmed these values while also showing that newer systems tend to have higher field intensities but do not have higher negative peak pressures14.
Duplex Sonography
Duplex sonography combines B-mode imaging with pulsed Doppler flowmetry. B-mode provides the sectional image for locating the site of interest and positioning the Doppler sample volume, while flowmetry yields the desired information on blood flow velocity.
Heating. Biological risks have been ruled out for B-mode imaging. Flowmetry requires the additional use of a Doppler beam of greater pulse length and higher intensity (ISPTA). The intensity of this stationary Doppler beam may fall within the upper range of therapeutic intensities. Animal experiments have shown that Doppler pulses can produce a biologically significant temperature rise in soft tissues, and that this effect can no longer be considered harmless4,23 (Fig. 2.2). The heating effect can become particularly hazardous when bone located below the Doppler sample volume is exposed to the ultrasound beam, since bone has a high absorption capacity and undergoes rapid heating. It was found, for example, that when fetal guinea-pig brain was insonated within the intact skull, the temperature rise was greater near the bone on the opposite side of the skull than at the center of the brain4. Based on these findings, it is conceivable that instruments with a high power output and prolonged scan time (>30 seconds) can induce temperature rises during flowmetry that cannot be considered harmless.
Cavitation. Because the negative peak pressure is no greater in Doppler ultrasound than in B-mode and does not exceed 5 MPa, cavitation should not occur.
Fig. 2.2
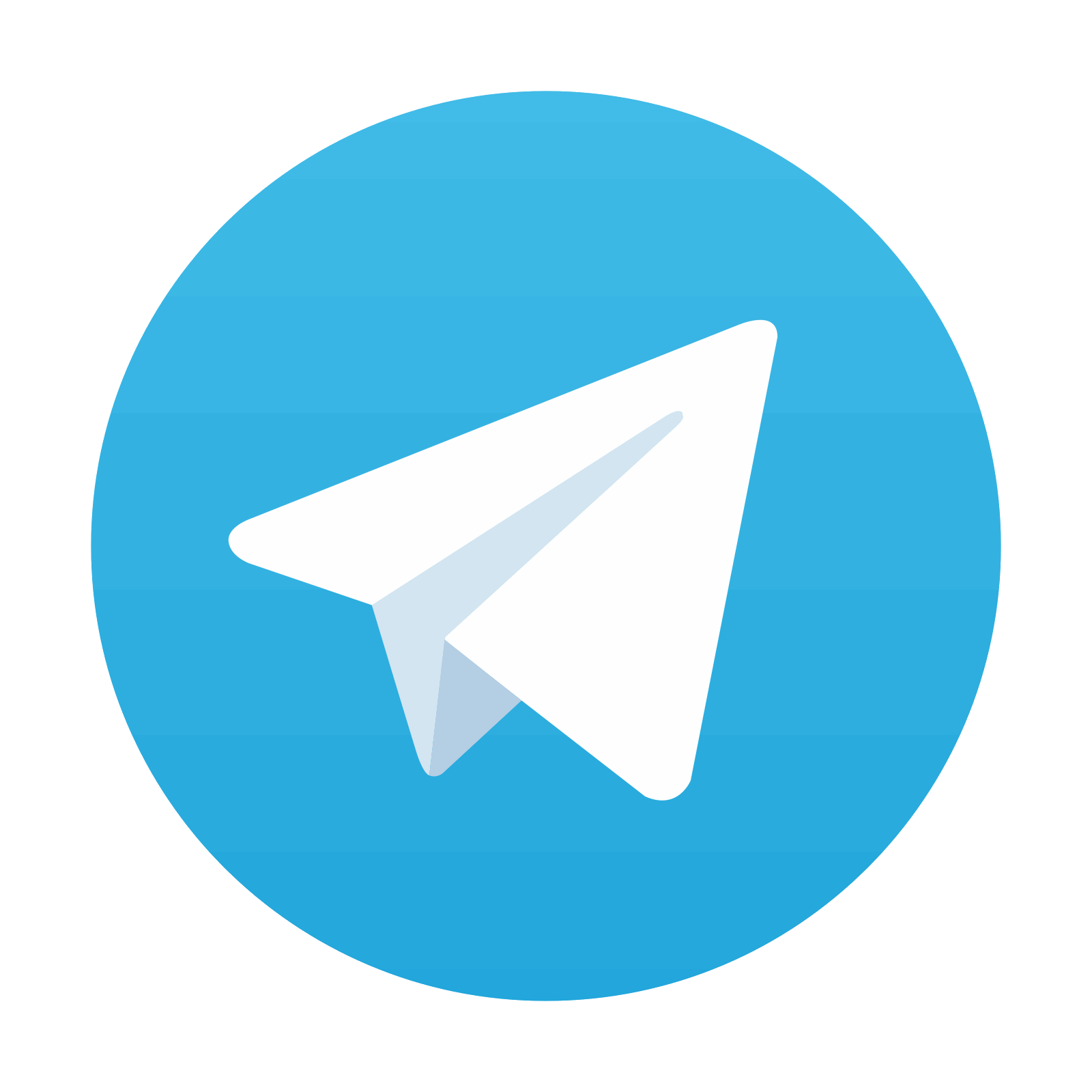
Stay updated, free articles. Join our Telegram channel

Full access? Get Clinical Tree
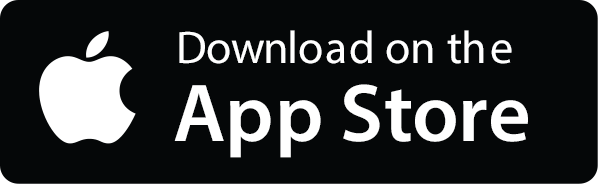
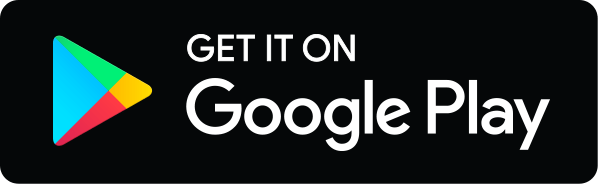