KEY POINTS
- 1.
Respiratory distress syndrome (RDS) remains an important cause of morbidity and mortality in preterm infants.
- 2.
The etiopathology of RDS involves structural immaturity and surfactant deficiency in the developing lung.
- 3.
The diagnosis rests on the presence of characteristic clinical and radiographic features in premature infants.
- 4.
Judicious use of antenatal steroids has reduced the frequency and severity of RDS.
- 5.
The use of continuous positive airway pressure, technologically advanced neonatal ventilation, and the availability of surfactant preparations have changed the clinical outlook in this condition.
- 6.
Heroic efforts by the neonatal community to conduct evidence-based testing of therapies have greatly advanced the science, sophistication, and outcomes of care.
Introduction
Respiratory distress syndrome (RDS), formerly known as hyaline membrane disease (HMD) and more recently simply as surfactant deficiency, has been a topic of discussion in the medical community for over a century. The diagnosis of RDS is based on the summation of clinical observations, required respiratory support, and findings on chest radiographs rather than on a gold-standard single diagnostic criterion. HMD was first described in 1903 in the German literature by Hochheim, who detailed the presence of hyaline membranes at autopsy in the lung of an infant who had died of respiratory disease. Over the next 50 years, multiple investigators proposed a variety of etiologies for HMD, including in utero inhalation of amniotic fluid.
In 1959, Avery and Mead authored a sentinel paper that compared the surface-tension-lowering abilities of extracts of minced lung from infants who had succumbed due to HMD and from infants without HMD who had died of other causes. They reported that the lung extracts of infants who died of HMD were not able to reduce surface tension on a modified surface-balance instrument, whereas lung extracts of infants who died of causes other than HMD were able to produce low surface tensions. Avery and Mead concluded that HMD was caused by a deficiency of a lung substance that prevents generalized atelectasis through its ability to reduce surface tension at the alveolar liquid-air interface.
In 1959, clinicians and pathologists gathered informally and attempted to define necessary clinical criteria to diagnose HMD and to grade its severity. Some of the clinical criteria included prematurity, history of intrauterine distress, family history of respiratory distress, diabetes, birth weight, respiratory rate, work of breathing, cyanosis, temperature instability, and hypotonia. The group also discussed laboratory and radiographic and autopsy criteria for diagnosis.
In 1963, President John F. Kennedy’s son died of HMD at a gestational age of 34 weeks. This event accelerated a new focus on maternal and infant healthcare that the National Institutes of Health had initiated in 1962 with the creation of the National Institute of Child Health and Human Development.
Research into mechanical ventilation, continuous positive airway pressure (CPAP), and eventually artificial surfactants expanded from the late 1960s into the 1980s. After years of failed attempts by the neonatology community to adapt adult techniques of mechanical ventilation to newborns, in 1971, Gregory and colleagues published a landmark study on the use of CPAP in infants with idiopathic RDS. This simple technique represented the first major therapeutic advance that effectively targeted and ameliorated the underlying pathophysiology of RDS.
Unfortunately, clinical translation of Avery’s observation of surfactant deficiency was delayed due to an imperfect understanding of the composition and physiologic function of natural surfactant. As a result, clinical trials that demonstrated no improvement in infants with HMD after tracheal instillation of phosphatidylcholine set back basic and clinical research on surfactant for nearly two decades. It was not until the late 1970s, when Fujiwara and colleagues showed efficacy of instillation of a bovine-derived surfactant in ameliorating lung disease in premature lambs, that interest in exogenous surfactant replacement therapy rekindled. Results from a series of in vitro studies that quantitated surface tension properties of artificial surfactants and animal studies that demonstrated efficacy of instilled artificial surfactants with respect to short-term improvements in pulmonary function led to small human trials in premature infants that replicated these effects. Subsequently, large randomized human clinical trials throughout the 1980s resulted in Food and Drug Administration (FDA) approval, in 1990 and 1991, of the first two exogenous surfactants for the prophylaxis and treatment of RDS. Although surfactant replacement therapy of infants at risk for or who have established RDS significantly improved survival and reduced early respiratory morbidities such as pulmonary air leaks, the hopes of clinicians that this treatment would also translate into a reduction in the incidence of bronchopulmonary dysplasia (BPD) were soon dashed.
Pathophysiology
As with most pathologic processes in neonatology, a robust understanding of both normal in utero and abnormal ex utero development of the pulmonary airways and vascular bed is needed to understand the clinical features of RDS. Lung development begins early in gestation (as early as 3 weeks’ gestation), and maturation progresses throughout the pregnancy, extending into childhood and potentially adolescence. Respiratory tract development is divided into five stages, which perforce overlap but nonetheless represent different structural evolutions that occur under changing hormonal environments ( Fig. 11.1 ). Depending at what point preterm birth exposes a neonate to unprogrammed treatment exposures and physiologic aberrations, subsequent disturbances of normal lung growth and development can have very different effects on long-term morbidity and mortality. The purpose of this chapter is not to provide a detailed description of embryology but instead to review the developmental biology briefly so that RDS and associated morbidities can be understood within the context of the embryologic background.

The first, or embryonic, stage of lung development occurs during the first 8 weeks of gestation. During this time, through complex interactions between transcription factors, growth factors, and surrounding mesenchyme, the early respiratory tract buds form from the developing foregut to create the trachea, the left and right main bronchi, and the bronchial buds (three on the right and two on the left) that will eventually become the lobes of the lungs. The pulmonary vasculature bed also begins to form during the embryonic stage and continues to branch and mature in parallel with extension of the airway tree throughout gestation. By the end of the second, or pseudoglandular, stage at 17 weeks’ gestation, branching of the large airways is completed. , The third or canalicular stage of lung development occurs between 16 and 26 weeks’ gestation. Given current therapies, fetuses first become viable at 22 to 23 weeks’ gestational age, in the middle of the canalicular stage. As might be expected, the formation of the first rudimentary gas exchange units occurs during this stage. However, the total surface area for gas exchange is only a fraction of that of a term infant. In addition, surfactant production is minimal, with the result that most infants born at this developmental stage who are not treated with exogenous surfactant or supported very early after birth with positive distending pressure will manifest signs of RDS and be at risk to develop associated sequelae. During the saccular stage (24–38 weeks’ gestation) and alveolar stage (36 weeks’ gestation and beyond), expansion of acini, a dramatic increase in the surface area of the air-lung interface, maturation of surfactant production, and reduction in diffusion distance between the acinus and pulmonary capillaries combine to allow the lungs to replace the placenta as the organ of gas exchange. ,
Diagnosis
The diagnosis of RDS depends on predisposing factors such as the degree of prematurity, the timing of onset, clinical conditions such as maternal diabetes, and the clinical and radiologic manifestations. The mainstay of diagnosis is radiography; findings such as low lung volumes, diffuse ground-glass haziness or a reticulogranular pattern, and air bronchograms are considered to be highly suggestive ( Fig. 11.2 ). Exciting work on the possibility that modalities such as ultrasound and computerized tomography may help in the assessment of severity and/or recovery is also ongoing ( Fig. 11.3 ).


Management
Early Interventions to Prevent RDS
In 2021, a gestational age of 22 to 23 weeks represented the earliest point in pregnancy at which preterm birth may result in a birth of an infant who survived to home discharge. However, many preterm infants who survive sustain significant respiratory and nonrespiratory morbidities. RDS is the hallmark respiratory morbidity of prematurity. Moreover, the treatment of RDS involves invasive procedures and intensive support that can lead to other complications. A major goal of prevention and early treatment strategies for RDS is to minimize other morbidities that may prolong recovery and affect the survival and long-term neurodevelopmental outcome. Although prevention or treatment of early RDS in the most premature infants does not by itself alter the likelihood of BPD, the totality of changes in the care of premature infants has altered the spectrum of anatomic, clinical, and physiologic manifestations of the “new BPD”—also referred to as chronic lung disease—among preterm survivors. Although beyond the scope of this chapter, continued focus on reducing the incidence and severity of BPD is critical because this condition can result in later infant mortality and often has long-term effects on subsequent respiratory health, neurodevelopment, and the quality of life of both the child and the family.
In this section, we will review several antenatal, delivery room, and early postnatal interventions with respect to their direct effect on the pathophysiology of RDS or their indirect effect as reflected by improved neonatal stabilization or via a reduction in the risk of later morbidity.
Antenatal Steroids
As more has been learned about the pathophysiology of RDS and the natural history of injury, repair, and development of the ventilated preterm lung, research has focused on the development of effective interventions that can reduce the severity of RDS. The most significant predisposing factor for RDS is premature birth. Administration of antenatal steroids in the setting of anticipated premature birth due to spontaneous labor or other maternal of fetal indication, for the purpose of accelerating the maturity of fetal lungs and other organs, remains the most effective antenatal therapy on behalf of the fetus. The benefit of antenatal steroids was first reported in 1969 by Liggins based on his observation that preterm lambs exposed to antenatal dexamethasone who normally would have succumbed to respiratory failure in fact had minimal respiratory dysfunction. The increase in lung inflation found on autopsy was likely due to accelerated production or secretion of surfactant. Based on this seminal observation, Liggins and Howie performed a randomized controlled clinical trial in 1972 that investigated the effects of antenatal betamethasone administered to mothers with threatened preterm delivery on neonatal respiratory outcomes. Their initial study demonstrated a significant decrease in the incidence of RDS in infants whose mothers received antenatal betamethasone (9%) compared with infants whose mothers did not receive the steroids (25.8%); however, the authors noted that the difference was significant only among infants born at less than 32 weeks’ gestational age and whose mothers had received treatment between 24 hours and 7 days prior to delivery. Since the initial trials by Liggins and Howie, there have been many studies that have examined more focused issues related to the efficacy of antenatal corticosteroids and the potential risks of this therapy. A 2017 Cochrane Review of 30 individual studies comprising 7774 women and 8158 infants provided definitive evidence for the efficacy and safety of antenatal corticosteroids. This review also identified areas that require further study, including outcomes in countries with fewer resources, outcomes among infants in multiple-gestation pregnancies, and potential long-term adverse outcomes.
The American College of Obstetricians and Gynecologists (ACOG) strongly recommends corticosteroid treatment for women between 24 0/7 and 36 6/7 weeks’ gestation who are judged to be at risk of a preterm delivery within 7 days. The statement also advises consideration of corticosteroid treatment between 23 0/7 and 23 6/7 weeks’ gestation, when active resuscitation is planned. This recommendation applies to multiple gestations and to pregnancies complicated by premature rupture of membranes. One additional “rescue” course of corticosteroids can be considered for women less than 34 0/7 weeks gestation who are at risk for preterm delivery within 7 days but who received their initial course of antenatal corticosteroids more than 14 days prior. Some centers now offer antenatal steroid therapy in the 22nd week of gestation, based on increasing survival rates of infants born at 22 to 23 weeks’ gestational age.
Delayed Cord Clamping
Until recently, clamping of the umbilical cord immediately after birth of an infant and prior to delivery of the placenta had been a routine obstetric practice. During the past two decades, multiple studies have revisited the optimal timing of cord clamping to elucidate potential benefits of delayed cord clamping (DCC) on short- and long-term outcomes of preterm and term infants. Two recent large meta-analyses of DCC in preterm infants reached different conclusions. Members of the International Liaison Committee on Resuscitation’s Neonatal Life Support Task Force examined 42 randomized controlled studies of DCC and intact-cord milking (ICM) that included 5772 premature infants <34 weeks’ gestational age. This analysis suggested at best a very modest survival benefit (risk ratio, 1.02) associated with either DCC or ICM, but the 95% confidence intervals for the risk ratios indicated there was no effect. A second analysis reported by Canadian investigators included 56 studies that included 6852 preterm infants and found that both DCC and ICM were associated with significantly lower odds of intraventricular hemorrhage and a need for packed red cell transfusions. DCC but not ICM was associated with lower odds of mortality. Nonetheless, neither study provided additional insight to alter a prior conclusion that the optimal duration of DCC is unknown. In term infants, DCC increases blood hemoglobin levels after birth and iron stores during the first several months of life at the expense of a small increase in the percentage of infants who receive phototherapy to treat neonatal jaundice. Current ACOG guidelines from 2020 supported DCC for at least 30 to 60 seconds for vigorous term and preterm infants.
Animal studies have suggested that resuscitation and assisted ventilation prior to cord clamping could assist with the cardiorespiratory transition at birth. , As a result, the feasibility, safety, and efficacy of resuscitation prior to cord clamping is under study. A 2019 Cochrane Review concluded that data remain insufficient to provide guidance on the advisability of resuscitation prior to cord clamping.
Oxygen Titration
Guidelines from the Neonatal Resuscitation Program (NRP) recommend resuscitation with 21% oxygen for infants greater than or equal to 35 weeks’ gestational age and resuscitation with 21% to 30% oxygen for infants less than 35 weeks’ gestational age. The NRP guidelines also provide recommended target peripheral oxygen saturations for newborns by minute after delivery to guide titration of supplemental oxygen. This focus on utilizing the lowest fraction of inspired oxygen (Fi o 2 ) is based primarily on concerns that early high partial pressure of oxygen (Pa o 2 ) levels increase the risk of severe retinopathy of prematurity; however, others have voiced concern that a 21% Fi o 2 is insufficient for resuscitation of infants born at <28 weeks’ gestational age. , A 2018 Cochrane Review also supports the need for further studies on the optimal Fi o 2 at delivery.
Respiratory Practices in the Delivery Room
Care for preterm infants immediately following birth has become more sophisticated during the past several decades with the advent of new pharmaceutical treatments and strategies of mechanical ventilation. A seminal 1987 report surveyed eight clinical centers to evaluate the incidence of chronic lung disease in preterm infants with birth weights between 700 and 1500 g. This report noted that one center, Columbia, reported a dramatically lower incidence of chronic lung disease among its preterm infants compared with all other centers. At the time, Columbia was the only center among the eight that initiated CPAP at birth, permitted partial pressure of carbon dioxide (Pa co 2 ) levels as high as 60 torr (permissive hypercapnia), and eschewed muscle relaxants. Since that controversial study, multiple other trials have compared the relative merit of CPAP to early intubation, especially in light of proposed benefits of early surfactant administration, including the COIN, SUPPORT, VON-DRM, CNRN, and CURPAP trials. Together, these studies have shown that early CPAP reduces the need for intubation and decreases days of mechanical ventilation. However, these studies did not support that CPAP compared with early intubation significantly reduced the primary outcomes of survival and/or BPD. Others have interpreted these studies as supporting a practice that limits surfactant treatment to a rescue intervention for a select group of neonates rather than espousing routine prophylactic surfactant treatment of all high-risk neonates. As a result, clinical practice has trended toward early use of noninvasive support directly after delivery rather than early intubation and surfactant treatment, although there are nuances in practice related to gestational age.
Postdelivery Care
Although the most clinically apparent effect of antenatal steroid therapy is a reduction in the incidence and/or severity of RDS due to accelerated fetal lung maturity, most postdelivery interventions and care guidelines aim to minimize other morbidities and other causes of mortality. With the increased emphasis on standardization of practice, the neonatology community has focused on choreographing care during the first “golden hour” of postnatal life. “Golden-hour” clinical protocols embrace a comprehensive bundle of practices that are supported in the evidence-based literature or that have some strong rationale based on expert consensus. This bundle best applies to the care of preterm infants who require some form of resuscitation following delivery.
Postnatal Strategies to Treat Established RDS
Surfactant
The use of exogenous surfactant to prevent and treat RDS has been the standard of care for decades. Several surfactants, both synthetic and animal-derived, have reduced the incidence, severity, and some short-term respiratory morbidities associated with RDS. The composition of surfactant is increasingly better known ( Fig. 11.4 ), and this has had exciting implications for both treatment and ongoing research focused on refining these products.

Types of Surfactants
First-Generation Synthetic Surfactants
In 1987, a multicenter trial of the synthetic surfactant pumactant (a 7:3 weight:weight ratio of the principal lung surfactant phospholipids dipalmitoylphosphatidylcholine [DPPC] and phosphatidylglycerol) demonstrated a significant reduction in mortality and respiratory support in very preterm infants even though this surfactant lacked surfactant proteins B (SP-B) and C (SP-C). The development of alternate synthetic additives that were chosen to mimic the roles of SP-B and SP-C in achieving low surface tensions led to production of the first FDA-approved completely synthetic and protein-free surfactant, colfosceril palmitate (Exosurf), for the treatment of RDS in the United States. Several randomized clinical trials that tested Exosurf for the prevention and treatment of RDS showed significant reduction in neonatal and infant mortality as well as in pulmonary air leaks. However, a 2001 meta-analysis established that treatment with these first-generation synthetic surfactants was associated with increased mortality and a greater risk of pneumothorax compared with treatment with animal-derived surfactants. The inferiority of first-generation synthetic surfactants, as demonstrated in vitro via bubble surfactometry and in vivo in animal models of RDS, was principally owed to the lack of substances that reproduced the critical roles of SP-B and SP-C. Exosurf eventually fell into disfavor with neonatologists due to its slower mechanism of action and its lesser ability to protect against pulmonary air leaks compared with surfactants derived from animal sources that more closely mirrored the composition of natural surfactant.
Animal-Derived Surfactants
Preliminary proof-of-concept animal studies published in the 1970 showed that preterm rabbits treated with natural surfactant that contained both phospholipids and surfactant-associated proteins reduced the clinical severity of RDS .
The first successful administration of exogenous surfactant to newborn infants with RDS was reported in 1980. An open-label trial of surfactant extract from bovine lungs (surfactant TA [Tokyo Akita]) improved mean respiratory parameters in 10 preterm infants. Subsequently, large, randomized, placebo-controlled or surfactant-comparison trials for the prophylaxis and treatment of neonatal RDS led to FDA approval of several surfactants in the 1990s (Exosurf, 1990; beractant [Survanta], 1991; calfactant [Infasurf], 1998; and poractant alpha [Curosurf], 1999). These surfactants differ in source, mode of preparation, composition, in vitro profile of surface tension properties, and in vivo profile of onset, magnitude, and duration of physiologic effects in animal models of RDS. Exosurf is a completely synthetic surfactant; Survanta and Infasurf are derived from an extract of minced cow lung and from a calf lung lavage, respectively; and Curosurf is derived from an extract of minced pig lung. Survanta requires addition of synthetic DPPC, tripalmitin, and palmitic acid to achieve acceptable surface-tension-lowering capabilities; Infasurf contains no additives; and Curosurf undergoes additional processing by liquid gel chromatography to concentrate polar phospholipids. Although Curosurf has higher concentrations of DPPC and SP-B than does Infasurf, Infasurf has a higher SP-B content as a percentage of phospholipids by weight that more closely approximates the composition of natural surfactant and may provide greater protection against natural proteins such as albumin and hemoglobin that can inhibit surfactant activity in vivo. Poractant alpha exhibits the lowest viscosity of all four surfactants, which may provide advantages for less invasive surfactant treatment (LIST) techniques.
Two large studies compared Exosurf and Infasurf in the prophylaxis and treatment of RDS. , The prophylaxis study demonstrated that compared with Exosurf, Infasurf significantly reduced the incidence of RDS, the severity of early lung disease, the incidence of pulmonary air leak, and the rate of mortality due to RDS but did not improve long-term outcomes including the incidence of BPD or the rate of survival without BPD. A parallel study of treatment of established RDS found that compared with Exosurf, treatment with Infasurf reduced the severity of lung disease and the incidence of pulmonary air leak without translation to a reduction in BPD or survival without BPD.
Trials that compared Survanta and Infasurf did not demonstrate significant differences in long-term clinical outcomes or dosing-related complications. However, among infants treated for RDS, a subgroup of Infasurf-treated infants demonstrated greater clinical improvement as evidenced by a lower inspired oxygen concentration and mean airway pressure in the first 72 hours of life and a longer interval between doses compared with infants treated with Survanta.
The first multicenter, placebo-controlled trial of Curosurf was reported in 1988. Among 146 infants, Curosurf significantly reduced neonatal mortality (from 51% to 31%), pneumothorax (from 35% to 18%), and pulmonary interstitial emphysema (PIE) (from 39% to 23%) and increased the Pa o 2 /Fi o 2 ratio by nearly threefold. A subsequent study demonstrated that retreatment with up to two additional doses of Curosurf within 24 hours of the initial dose led to lower ventilator requirements at 2 to 4 days after randomization compared with a single treatment. Subsequent studies of Curosurf showed that early treatment produced more favorable results compared with later administration.
Six randomized comparison trials of Curosurf and Survanta have been published to date. These studies showed that Curosurf-treated infants demonstrated more rapid reductions in Fi o 2 and mean airway pressure (MAP), less frequent repeat dosing, fewer days on mechanical ventilation, lower mortality among infants less than 32 weeks’ gestational age, fewer air leaks, and increased survival without BPD compared with infants treated with Survanta. In a large retrospective study, Ramanathan et al. compared 14,173 infants treated with Curosurf, Infasurf, and Survanta and found significantly higher mortality rates in infants treated with Infasurf and Survanta compared with Curosurf-treated infants. However, a larger retrospective study that evaluated comparative effectiveness between these three surfactants in 51,282 infants showed no differences in mortality.
A systematic review and meta-analysis of five randomized controlled trials (RCTs) compared outcomes of 529 infants who received either Curosurf or Survanta treatment for established RDS. The incidence of oxygen dependence at a postmenstrual age of 36 weeks was similar in both groups. Infants treated with low-dose (100 mg/kg) or high-dose (200 mg/kg) Curosurf demonstrated statistically significant reductions in death, the need for repeat dosing, Fi o 2 administration, and durations of treatment with mechanical ventilation and supplemental oxygen. Statistically fewer deaths occurred among Curosurf-treated compared with Survanta-treated infants. The need for repeat dosing was lower with high-dose but not with low-dose Curosurf. Studies of other surfactant preparations have shown that use of a higher dose is associated with better clinical outcomes. Use of a higher dose of Curosurf results in longer physiologic half-life, a lower oxygenation index, and less frequent repeat dosing. Another meta-analysis concluded that compared with treatment with Survanta, treatment with Curosurf resulted in fewer complications associated with administration, reduced the need for repeat dosing, led to greater improvements in acute respiratory parameters, and reduced the risk of mortality. In the most recent Cochrane reviews, significant differences in outcomes were noted when trials of beractant were compared with poractant alpha, including a significant increase in the risk of mortality prior to discharge, death, oxygen requirement at 36 weeks of postmenstrual age, patent ductus arteriosus (PDA) requiring treatment, and patients receiving more than one dose of surfactant in infants treated with beractant compared with poractant alpha. The difference in these outcomes was observed only in the studies that employed the higher initial dose of Curosurf.
Second-Generation Synthetic Surfactants
Second-generation synthetic surfactants that incorporated compounds meant to reproduce in vivo functions of SP-B or SP-C offset some of the shortcomings of first-generation surfactants. The main attractions of these surfactants were the homogeneity of surfactant preparation and the elimination of any risk related to transmission of prion-mediated disease (mad cow disease or bovine spongiform encephalopathy that results in variant Creutzfeldt-Jakob disease in humans). ,
The two second-generation synthetic surfactants that have undergone clinical trials are lusupultide (Venticute) and lucinactant (Surfaxin). Venticute contains recombinant SP-C, but it was not studied in neonates and was mainly used in clinical trials in adults with acute lung injury. Surfaxin contains two phospholipids, a fatty acid, and a synthetic peptide that has surface-tension-lowering properties similar to that of SP-B, and it was approved for use by the FDA in 2012.
Surfaxin was compared with Exosurf and Survanta in a trial that enrolled 1294 infants born before 33 weeks’ gestational age. Prophylactic Surfaxin (administered within 30 minutes of life) significantly decreased rates of RDS at 24 hours after birth and significantly reduced RDS-related mortality. Compared with Exosurf, Surfaxin also reduced the rate of BPD at 36 weeks’ postmenstrual age. Compared with Survanta, Surfaxin reduced RDS-related and overall deaths but did not change other morbidities associated with prematurity. The Surfaxin Therapy Against RDS (STAR) study compared Surfaxin with Curosurf in preterm infants using a noninferiority trial design but was prematurely terminated due to slow recruitment. These data collectively suggest that second-generation synthetic surfactants achieve outcomes comparable to those of first-generation animal-derived surfactants. However, the advantages of Surfaxin did not outweigh practical disadvantages (the need for large volumes, prewarming, and multiple doses), and the product was withdrawn from the market in 2015.
Third-Generation Synthetic Surfactants
In animal studies, third-generation synthetic surfactant has been shown to be superior compared with Infasurf. In a study with preterm lambs, a synthetic surfactant containing both SP-B and SP-C analogs (CHF 5633) resisted inactivation better than Curosurf. A phase 1 human clinical trial of synthetic surfactant CHF 5633 in RDS involved 40 preterm infants born at 27 to 34 weeks’ gestational age (20 infants received 100 mg/kg and 20 received 200 mg/kg). Both doses were well tolerated and showed promising clinical efficacy. Recently reported phase 2 multicenter double-blind randomized controlled clinical trials compared CHF 5633 (200 mg/kg dose with repeat doses of 100 mg/kg if indicated) with Curosurf for treatment of established RDS. The Fi o 2 and the respiratory severity score (defined as Fi o 2 × mean airway pressure) decreased from baseline at all time points assessed ( P < .001), but there was no statistically significant difference between groups. Moreover, the use of rescue surfactant and the incidence of BPD and mortality at day 28 were similar in the two treatment groups. No immunogenicity was detected per the report, and the authors concluded that treatment with CHF 5633 demonstrated clinical efficacy and safety similar to that of Curosurf in the treatment of preterm neonates with moderate to severe RDS.
Indications for Surfactant Administration
The American Academy of Pediatrics and the updated 2019 European Consensus Guidelines have recommended institution of nasal CPAP as the first treatment modality for an infant at risk for and demonstrating clinical signs of RDS, with deferment of intubations and surfactant administration until or unless the infant demonstrates apnea or develops severe disease. These guidelines suggest that subsequent surfactant administration (persistent requirement of an Fi o 2 >30%) may decrease mortality and morbidity in infants with RDS born before 30 weeks’ gestational age. Multiple doses of surfactant using the INSURE procedure (intubate, administer surfactant, extubate) has also been successfully used and has not been shown to worsen outcome.
The 2019 European Consensus Guidelines state that infants should be given natural surfactant, preferably as an early rescue treatment, if there is evidence of RDS (Fi o 2 > 0.30 on CPAP pressure of at least 6 cm H 2 O). They suggest administering higher doses of Curosurf (200 mg/kg) and support less invasive surfactant administration (LISA) as the preferred mode of administration in spontaneously breathing infants.
Timing of Surfactant Administration
Prophylaxis Versus Rescue Surfactant
In an era where antenatal treatment with steroids is nearly universal, the strategy to treat with prophylactic surfactant compared with a strategy of immediate lung recruitment using nasal CPAP is associated with a greater risk of death or BPD (risk ratio [RR], 1.13; 95% confidence interval [CI], 1.02–1.25). Hence, in infants who have the advantage of adequate antenatal steroid exposure and where the resuscitation team can achieve effective lung recruitment with nasal CPAP or noninvasive ventilation (NIV), surfactant therapy in the delivery room can be safely deferred.
Early Versus Delayed Rescue Surfactant
One clinical trial that compared the two strategies of early versus later threshold-based intratracheal surfactant treatment of preterm infants on nasal CPAP showed that early rescue treatment was associated with significantly less need for intubation and/or death before 7 days of life and lower mortality at hospital discharge. A similar clinical trial a decade later demonstrated significantly less need for intubation as well as less a lower incidence of air leaks associated with early rescue treatment. A decrease in the duration of mechanical ventilation is an important clinical outcome, especially when medical resources are limited, and it may result in less BPD in both developed and low-resource areas. However, the optimal timing for surfactant administration remains unclear, especially among infants treated with NIV.
Techniques of Surfactant Administration: INSURE, INSURE, and INRecSURE
Initially, surfactant administration occurred via prophylactic rapid bolus intratracheal instillation (to a fluid-rich lung in the delivery room) in infants at high risk for RDS or by slower infusion of multiple aliquots in infants with established RDS after endotracheal intubation. Prophylactic surfactant has fallen into disfavor because clinicians prefer to recruit surfactant-deficient lungs with early application of nasal CPAP and/or initiation of NIV support and to reserve surfactant treatment only for infants who exceed a prespecified threshold of respiratory support. The INSURE procedure originated in the early 1990s as an attempt to reduce the duration of an indwelling endotracheal tube. , This technique involves surfactant instillation after endotracheal tube intubation followed by rapid extubation after a brief period of mechanical ventilation. Leone et al. showed that the INSURE procedure resulted in a longer duration of improved oxygenation compared with rescue surfactant administration during invasive mechanical ventilation. In addition, premature infants treated with INSURE developed fewer respiratory comorbidities, including pneumothorax, BPD, and death or BPD. A recent meta-analysis that included nine clinical trials and 1551 infants compared INSURE to CPAP alone. There were no statistically significant differences between early INSURE and CPAP alone for all outcomes, such as the combined outcome of BPD and/or death, BPD, death, air leaks, severe IVH, neurodevelopmental delay, or death and/or neurodevelopmental impairment. The authors concluded that “currently, no evidence suggests that either early INSURE or CPAP alone is superior to the other.” Although INSURE minimizes the duration of invasive ventilation, it may not represent the ideal method of surfactant administration because of (1) the need to administer opioid medication before intubation and (2) the not infrequent reluctance of providers to extubate promptly. Concerns that even a brief period of ventilation can initiate lung injury in a vulnerable preterm infant have led to the exploration of alternative surfactant administrations strategies to avoid tracheal intubation. This has led to use of the laryngeal mask airway (LMA) and special catheters such as feeding tubes for surfactant instillation and to the development of a modified INSURE (mINSURE) procedure.
A randomized trial of the LMA among preterm infants with RDS with a gestational age greater than 29 weeks and a birth weight greater than 1000 g found a decreased need to initiate mechanical ventilation in the LMA compared with the INSURE group. The mINSURE procedure typically involves surfactant administration using a small tube (e.g., a feeding tube, an angiocatheter, or a specially made small-diameter catheter) while the baby is breathing spontaneously on nasal CPAP or nasal intermittent positive pressure ventilation (NIPPV), without using premedication. This technique, most commonly termed LISA , or minimally invasive surfactant treatment (MIST), , is currently being studied in many centers around the world. Investigators have used many other names for the mINSURE procedure, including avoidance of mechanical ventilation, surfactant without intubation, the Take Care method, Sonda Nasogastrica SURfactante Extubacion (SONSURE), Early CPAP and Large volume Modified InSure Technique (ECALMIST), and Minimally Invasive SURFactant administration (MISURF). Kanmaz compared surfactant administration in infants breathing spontaneously on CPAP using a 5F sterile flexible nasogastric tube (Take Care) with the INSURE procedure. The duration of CPAP and mechanical ventilation and the incidence of BPD were significantly shorter in the Take Care group compared with the INSURE group. With a decreased need for and duration of mechanical ventilation, infants in the Take Care group had a lower rate of BPD. Use of the LISA technique in a single-center cohort of 224 infants born between 23 and 27 weeks’ gestational age was associated with significantly higher survival rates and decreased incidences of total IVH, severe IVH, and cystic periventricular leukomalacia. In a similar study, infants on CPAP who received surfactant replacement therapy via a thin catheter experienced a significantly lower rate of intubation and mechanical ventilation compared with infants treated using the INSURE procedure (19.2% versus 65%). In addition, the incidence of BPD was lower among infants who received surfactant via a catheter. In a recent multicenter trial from the German neonatal network, use of the nonintubated surfactant application (NINSAPP) technique in 211 preterm infants born between 23 and 26 weeks’ gestational age resulted in significantly higher combined survival without severe adverse events compared with administration of surfactant via an endotracheal tube to infants on mechanical ventilation. In another study, MIST has been shown to result in a rapid and homogenous increase in end-expiratory lung volume and improved oxygenation.
A systematic review and meta-analysis of six RCTs using mINSURE or LIST techniques to administer Curosurf in premature infants with RDS resulted in decreased risks of BPD (RR, 0.71 [0.52–0.99]; number needed to treat [NNT], 21), death or BPD (RR, 0.74 [0.58–0.94]; NNT, 15), early CPAP failure (RR, 0.67 [0.53–0.84]; NNT, 8), and invasive ventilation requirements (RR, 0.69 [0.53–0.88]; NNT, 6). Compared with INSURE, LIST decreased the risks of BPD and/or death (RR, 0.63 [0.44–0.92]; NNT, 11) and of early CPAP failure (RR, 0.71 [0.53–0.96]; NNT, 11). In another meta-analysis, the use of the LISA technique reduced the composite outcome of death or BPD (RR, 0.75 [95% CI: 0.59–0.94]; P = .01) and BPD at 36 weeks’ postmenstrual age among survivors (RR, 0.72 [0.53–0.97]; P = .03), the use of mechanical ventilation within 72 hours of birth (RR, 0.71 [0.53–0.96]; P = .02), and the use of mechanical ventilation at any time before hospital discharge (RR, 0.66 [0.47–0.93]; P = .02). There were no differences in total mortality and other neonatal morbidities. A large, multinational, multicenter, randomized masked controlled trial (OPTIMIST TRIAL) in preterm infants born between 25 and 28 weeks’ gestational age that compares surfactant delivery using a semirigid surfactant instillation catheter to sham treatment is currently underway.
A 2021 report of a multicenter trial of 35 centers (involving 218 spontaneously breathing infants on nasal CPAP who met failure criteria) compared outcomes in infants randomized to INSURE versus a novel method of surfactant administration and rapid extubation after lung recruitment with high-frequency oscillation (INtubate-RECruit-SURfactant-Extubate: IN-REC-SUR-E). The use of mechanical ventilation during the first 72 hours of life was reduced in the IN-REC-SUR-E group (40%) compared with the IN-SUR-E group (54%; adjusted RR, 0.75; 95% CI, 0.57–0.98; P = .04; NNT, 7.2; CI, 3.7–135). Infants in the IN-REC-SUR-E group had nonsignificantly lower rates of mortality at discharge (19% in the IN-REC-SUR-E group versus 33% in the IN-SUR-E group), pneumothorax (4% versus 6%), and severe (grade 3 or 4) IVH (12% versus 15%). The authors speculated that the decreased use of mechanical ventilation in the first 72 hours may facilitate successful use of noninvasive respiratory support strategies.
Some trials have studied even less invasive methods of surfactant administration by aerosolization, nebulization, or atomization. Actual or theoretical advantages of administering aerosolized surfactant include ease of administration, avoidance of hypoxemia, more homogenous distribution, less likelihood of airway complications, and less use of mechanical ventilation. In an open-label pilot study of aerosolized Surfaxin, the authors concluded that aerosolized surfactant could be safely given via CPAP as an alternative to surfactant administration via endotracheal tube. Another study examined the feasibility of administering two doses of aerosolized Survanta (100 versus 200 mg phospholipid/kg) in infants on noninvasive respiratory support (high-flow nasal cannula [HFNC], nasal CPAP, and NIPPV) through short binasal prongs within 72 hours of birth. Cummings et al. randomized infants with early RDS to aerosolized Infasurf or conventional therapy and found that aerosolized surfactant reduced the rate of intubation and mechanical ventilation by nearly 50% but did not affect respiratory or survival outcomes at 28 days.
Other Respiratory Approaches
Caffeine Therapy
Many centers have adopted caffeine therapy as a standard practice in the management of premature infants. The typical loading dose of caffeine citrate is 20 mg/kg loading followed by a daily maintenance dose of 5 to 10 mg/kg. The Caffeine for Apnea of Prematurity (CAP) trial showed that caffeine was associated with earlier extubation and a reduced use of supplemental oxygen at 36 weeks’ postmenstrual age. Most notably, neurodevelopmental follow-up through 18 to 21 months’ corrected age showed significantly lower rates of cerebral palsy and cognitive delay in infants randomized to caffeine therapy. The literature supports a strategy of early caffeine administration, before the onset of apnea of prematurity, to achieve maximum efficacy.
Permissive Hypercapnia
Under the theoretical framework that the likelihood of BPD increases with longer exposures to supplemental oxygen and positive pressure ventilation, it was reasonable to test the hypothesis that use of ventilatory strategies that minimize volubarotrauma might reduce the incidence of BPD. One anticipated consequence of less aggressive mechanical ventilation is a higher Pa co 2 , referred to as permissive hypercapnia.
To date, no evidence supports greater efficacy for ventilation strategies that result in permissive hypercapnia. In fact, post hoc analysis of data from the SUPPORT trial showed an association between higher Pa co 2 and a greater risk of death, intraventricular hemorrhage, BPD, and adverse neurodevelopmental outcome. The Permissive Hypercapnia in Extremely Low Birthweight Infants (PHELBI) trial randomized ventilated preterm infants to two target P co 2 levels for the first 14 days of ventilation, the higher arm reaching about 75 mm Hg and the lower arm about 60 mm Hg. In this study, the primary outcome of death or BPD was the same in both groups. Moreover, the rates of mortality, intraventricular hemorrhage, and retinopathy of prematurity did not differ between groups. Results from these important trials highlight the need for further evaluation of ideal Pa co 2 targets in preterm infants.
Postnatal Steroids
Lung inflammation is a characteristic finding in neonates who develop BPD. Strategies that reduce lung inflammation during the acute stage of RDS may potentially limit the time on mechanical ventilation and the incidence or severity of BPD. Dating back to the presurfactant study by Avery and colleagues, neonatologists have used postnatal steroids under diverse treatment protocols. Enthusiasm for steroid use has waxed and waned over time, and it is fair to summarize that no consensus exists about an optimal treatment protocol. Although many studies showed that postnatal dexamethasone therapy improved short-term respiratory outcomes and decreased BPD, steroid treatment has declined dramatically after investigators identified an association with an increased risk of cerebral palsy. However, BPD is also associated with death and adverse neurologic outcomes, and it is possible that infants who are at high risk of or have severe BPD might, on balance, benefit from steroid treatment. A short course of low-dose dexamethasone (<0.2 mg/kg/day) is an option for infants who remain intubated, especially after 1 to 2 weeks. Inhaled budesonide could be a viable alternative to systemic steroids. A large RCT of 863 infants showed that treatment with early (<24 hours after birth) inhaled budesonide significantly reduced the incidence of BPD (from 38% to 28%); however, a nonsignificant increase in mortality was also noted (16.9% versus 13.6%).
Inhaled Nitric Oxide
Inhaled nitric oxide (iNO) achieves pulmonary vasodilatation and theoretically has anti-inflammatory effects. A meta-analysis of all clinical trials that have tested the hypothesis that iNO will decrease BPD or the combined outcome of mortality or BPD has concluded that neither routine prophylactic iNO initiation nor rescue therapy with iNO improves these outcomes. The state of the evidence is that that nitric oxide should not be used to treat preterm infants with RDS or later respiratory conditions that result in hypoxemia. Some evidence suggests that use of iNO in the premature infants with pulmonary hypoplasia and/or documented pulmonary hypertension, by extrapolation to studies in term neonates, may improve outcomes.
Noninvasive Ventilation
NIV (e.g., modes of ventilation that do not require endotracheal intubation) is considered the preferred method of providing support to preterm infants with RDS. NIV modalities include CPAP devices, NIPPV, and humidified oxygen delivered by HFNC. Traditionally, noninvasive methods were used as a step-down from ventilation through an endotracheal tube, and initially, CPAP was the only method used. Further studies showed that initiation of CPAP immediately upon delivery followed by intubation and surfactant administration if needed was not inferior to strategies of routine intubation for stabilization or prophylactic surfactant administration.
Many centers have adopted NIPPV as their primary mode of ventilatory support, using conventional ventilators to deliver peak inspiratory pressures. NIPPV has been shown to reduce extubation failure, but it has not consistently been associated with a reduction in BPD. Studies in which NIPPV was most successful used synchronization of inspiratory pressure; however, delivering effective synchronization using flow sensors is challenging due to large leaks during CPAP, and it is unclear whether unsynchronized NIPPV is less effective.
The use of heated humidified HFNC as an alternative to CPAP has increased in popularity as a respiratory support for newborn infants due to its relative ease of use and perceived improvement in patient comfort. A recent meta-analysis of 15 studies comparing HFNC with other modes of noninvasive respiratory support showed similar rates of efficacy for preventing treatment failure, death, and BPD. Additionally, following extubation, HFNC was reported to be associated with less nasal trauma and might be associated with reduced pneumothorax compared with nasal CPAP. However, the role of HFNC as a first-line therapy for RDS has been studied in only a few trials, most of which have employed noninferiority designs. HFNC was used as the first-line mode of respiratory support in the delivery room in a small study that demonstrated feasibility, but in a large multicenter clinical trial that compared HFNC with nasal CPAP as the initial support modality for preterm infants with respiratory distress, neonates managed with HFNC had a significantly higher rate of treatment failure than neonates whose care was managed with nasal CPAP (25.5% versus 13.3%). Further studies are needed to evaluate the safety and efficacy of HFNC in subgroups of extremely preterm infants and to compare different HFNC devices.
In one noninferiority trial, a total of 316 infants were randomized either to HFNC (4–6 lpm) or CPAP/BiPAP (4–6 cm H 2 O), and the initiation of mechanical ventilation within 72 hours from the beginning of respiratory support was examined as the primary outcome. Failure occurred in 10.8% versus 9.5% of neonates in the HFNC and CPAP groups, respectively (95% CI of risk difference, 6.0%–8.6% within the noninferiority margin; P = .71). The authors concluded that use of HFNC resulted in profile of safety and efficacy similar to that of the use of CPAP/ bilevel positive airwary pressure (BiPAP) as the initial approach to managing preterm infants more than 28 weeks’ gestational age with mild to moderate RDS. In another recent and larger trial, 754 infants were randomized to either HFNC or CPAP in nontertiary intensive care nurseries. The primary outcome was a predefined treatment failure within 72 hours after randomization. Treatment failure occurred in 78 of 381 infants (20.5%) in the HFNC group and in 38 of 373 infants (10.2%) in the CPAP group (risk difference, 10.3 percentage points; 95% CI, 5.2–15.4).
Mechanical Ventilation Strategies
Almost 50% of extremely preterm infants with RDS do not tolerate NIPPV and progress to intubation and mechanical ventilation. Common to all mechanical ventilation strategies for RDS is the tenet of optimizing lung volumes for gas exchange without overdistending airways or alveolar units, thus avoiding volubarotrauma and hypocarbia. Hyperdistention increases the risk of air leaks such as pneumothorax and PIE. Using too low a mean airway pressure in an infant with RDS, on the other hand, will result in atelectasis that impairs gas exchange.
Pressure-limited, flow-cycled ventilation and volume-targeted ventilation are the most common modes used. Pressure-limited ventilation does not have any control over volume generated and potentially leads to excess tidal volumes, especially in the setting of rapid compliance change after surfactant administration in infants with RDS. Excessively high-tidal volumes expose the lung to volutrauma from overdistention, and the increased minute ventilation can cause hypocarbia that has been associated with certain types of brain injury. , In contrast, low-tidal volumes may cause uneven inflation of alveoli, resulting in hypercarbia and an agitated infant who must assume an increased work of breathing. A volume-targeted mode, on the other hand, delivers controlled tidal volumes and automatically will wean distending pressure as lung compliance improves. Compared with pressure-cycled ventilation, the volume-targeted ventilation may reduce the rate of death and/or BPD, the rate of intraventricular hemorrhage, and the duration of mechanical ventilation. ,
When high pressures are needed to achieve adequate lung inflation, many clinicians use high-frequency oscillatory ventilation (HFOV) in preference to conventional mechanical ventilation. HFOV allows gas exchange at very low-tidal volumes delivered at very fast rates while optimal lung inflation is maintained using a continuous high distending pressure. A recently updated meta-analysis of RCTs that compared HFOV with conventional ventilation showed that use of elective HFOV compared with conventional ventilation resulted in a small reduction in the risk of BPD, but this evidence was weakened by the inconsistency of this effect across trials. In addition, infants managed with HFOV experienced a higher rate of acute air leak. Adverse effects on short-term neurologic outcomes have been reported in some studies, but these effects did not achieve overall significance. Most trials reporting long-term outcome have not identified any difference.
Infants with air leaks, including PIE, can be effectively managed with the high-frequency jet ventilator (HFJV). The HFJV offers theoretical advantages compared with HFOV, including lower frequencies and higher expiratory times and the ability to “sigh” the lungs using a low rate of conventional breaths.
Irrespective of the choice of ventilator used, clinicians should be careful to avoid the extremes of hypocarbia and hypercarbia due to their association with an increased risk of BPD, periventricular leukomalacia, and intraventricular hemorrhage. Continuous end-tidal CO 2 monitoring can be helpful, especially during the first few days of ventilation. Weaning of pressure support should commence immediately upon establishment of effective gas exchange or improvement in lung compliance.
Nonrespiratory Strategies
Temperature Control
Maintaining body temperature between 36.5°C and 37.5°C during delivery room stabilization and NICU admission is extremely important for preterm infants. To facilitate achieving this goal, labor and delivery units should provide an environmental temperature greater than 25°C at the time of delivery of a preterm neonate. Initial stabilization of neonates less than 32 weeks’ gestational age should be performed using a polyethylene bag under a radiant warmer. Warming and humidification of gases required for stabilization are helpful in achieving normothermia.
Following admission to the NICU, the premature neonate should be managed in servo-controlled incubators set at 36.5°C with high relative humidity to reduce insensible water losses. For very premature infants, who have gelatinous skin and very high insensible water loss, a humidity of 70% to 80% is required initially, which can be reduced over time as the skin keratinizes and insensible losses decrease. Infants should be maintained in a thermal environment that maintains the core body temperature between 36.5°C and 37.5°C.
Fluid Management
Maintaining fluid balance can be challenging, because the most premature neonates initially can lose very high amounts of fluid per kilogram of body weight via evaporation through an immature skin barrier. One goal of fluid administration is to achieve a slightly negative balance to allow the reduction in extracellular fluid that occurs naturally after birth. Excessive fluid intake has been independently associated with higher rates of PDA, necrotizing enterocolitis, and BPD.
Routine use of diuretics (particularly furosemide) in preterm infants with RDS should be avoided due to lack of demonstrated benefit. Diuretic use often leads to electrolyte abnormalities, especially hyponatremia and hypokalemia, as well as hypochloremic alkalosis, which may elevate P co 2 and result in higher ventilatory pressures.
Nutritional Management
Parenteral nutrition should be started immediately after admission to the NICU. Early initiation of amino acids leads to positive nitrogen balance, decreases time to regain birth weight, and increases the rate of weight gain through discharge. Minimal enteral nutrition with breast milk should be initiated as soon as possible. Breast milk is the preferred option for initiation of feeding; however, if unavailable, donor breast milk is a reasonable alternative to formula feeding and may reduce the risk of necrotizing enterocolitis.
Judicious Use of Antibiotics
Traditionally, clinicians treat premature infants with RDS with antibiotics on the supposition that maternal infection is a cause of premature birth. More recent evidence has shown that routine prolonged antibiotic use without any evidence of infection may result in greater harm. Hence, antibiotics should be used more judiciously and discontinued promptly when there is no clear evidence of infection. Neonatal treatment with antibiotics is not indicated when preterm delivery occurs for maternal or fetal indications without preterm labor or evidence of maternal infection.
Cardiovascular Management
In premature infants, blood pressure is lower in the first few hours of life and increases gradually during the first 24 hours of life. Blood pressure varies with gestational age as well as chronologic age. Hypotension in infants with RDS may result from hypovolemia, large left-to-right ductal shunts, or myocardial dysfunction.
Hypovolemia can be minimized by delaying cord clamping. When cautious volume expansion is unsuccessful, dopamine is more effective than dobutamine in increasing blood pressure and also may improve cerebral blood flow in hypotensive infants. Epinephrine and hydrocortisone are typically used to treat refractory hypotension when other options have failed to optimize blood pressure and systemic perfusion.
PDA in very preterm infants with RDS can cause low blood pressure, poor systemic tissue perfusion, and pulmonary edema and may delay weaning from mechanical ventilation. Permissive tolerance of PDA in an infant without clear hemodynamic instability is an acceptable strategy if the infant is thriving, tolerating feeds, and requiring minimal respiratory support.
Complications of Treatment
The routine use of antenatal steroid therapy and the avoidance of atelectasis with early initiation of positive pressure and/or surfactant administration has significantly reduced early pulmonary complications associated with RDS. Complications still occur related to the underlying nature of the disease or to therapeutic interventions such as placement of arterial catheters, the use of supplemental oxygen and positive pressure ventilation, and the use of endotracheal tubes.
Complications Due to Endotracheal Tubes
Adverse events related to intubation can occur in as many as 40% of neonates. Displacement or misplacement of the endotracheal tube may occur on occasion. Inadvertent endotracheal tube positioning into a main stem bronchus (usually the right) is the most common occurrence and results in hyperinflation of the ventilated lung and atelectasis of the contralateral lung. The hyperinflation increases the risk of acute air leak. Other complications associated with prolonged intubation include subglottic stenosis and atelectasis after extubation. Esophageal and pharyngeal perforations are rare and usually occur with devastating results in the most fragile and premature neonates.
Pulmonary Air Leaks
Pulmonary air leaks are common acute complications of RDS and occur with an incidence of approximately 6% in all low birth weight infants. Air leaks result from the rupture of overdistended alveoli. These may occur during spontaneous breathing (in the era before ventilators, the incidence of pneumothorax was 25% in neonates with RDS) or in association with positive-pressure mechanical ventilation. Air may dissect toward the hilum, resulting in a pneumomediastinum, or more commonly into the pleural space, resulting in a pneumothorax.
Less commonly, air may dissect into the pericardial space, subcutaneous tissue, or peritoneal space to produce pneumopericardium, subcutaneous emphysema, or pneumoperitoneum, respectively. In the preterm infant, the more abundant and compliant perivascular connective tissue allows air to be trapped in the perivascular space, producing PIE.
The risk of pneumothorax increases with decreasing gestational age. Pneumothorax can also result from progressive lung overinflation as compliance improves after surfactant administration. Pneumothoraces can be managed by insertion of tubes or catheters connected to a negative pressure drainage system, and they usually resolve within days. On the other hand, intrapulmonary air leak (pulmonary interstitial emphysema) cannot be relieved by negative pressure drains and often takes more than 5 days to resolve.
Pneumothorax may occur spontaneously within the first few breaths, and if large, it will present with tachypnea, grunting, and retractions. In larger neonates who are more difficult to transilluminate, a chest radiograph may be required to differentiate it from RDS. Pneumothorax in a neonate on ventilatory support often presents acutely with an acute increase in heart rate, decrease in blood pressure due to cardiac tamponade, and sudden onset of hypoxemia and hypercapnia. Pneumothorax should always be suspected in neonates who have recently been treated with surfactant if they experience a sudden clinical deterioration after treatment.
A bronchopleural fistula is an abnormal acquired communication between the bronchial tree and pleural space. Clinically, this may present as a persistent air leak or as a failure of the lung to reinflate despite good chest tube drainage for greater than 24 hours. Neonates with a large fistula are difficult to ventilate (because most tidal volume is diverted through the fistula) and demonstrate persistent lung atelectasis and delayed weaning from mechanical ventilation.
Ventilator Management of Complications of RDS
Management of pneumothorax is based on the cause and severity of illness. Most often, pneumothoraces require continuous drainage and resolve within days. To minimize the likelihood of air leak, ventilatory parameters should be adjusted to maintain tidal volumes of 3 to 4 mL/kg and inspiratory times of approximately 0.3 seconds. A tension pneumothorax may be temporarily treated by needle aspiration but almost always requires subsequent chest tube placement due to a continuing large air leak. High frequency ventilation (HFV) is the preferred modality for management of persistent or refractory pneumothorax. Infants may be maintained on HFV until the pneumothorax resolves completely. Some infants may be able to be extubated to NIPPV.
Infants with PIE are managed conservatively. The majority of these infants are extremely premature and already receiving significant ventilatory support. Most often these infants are transitioned to HFOV or HFJV. Lower peak pressures provided by HFV aid in decreasing ongoing air leak while promoting more rapid healing of distal airway injury.
An HFJV is most frequently used when a neonate fails HFOV. This mode enhances ventilation at lower peak and mean airway pressures while maintaining a constant inspiratory time. One tradeoff in maintaining lower ventilatory pressures to facilitate resolution of PIE is a transient need for higher Fi o 2 (0.5–0.75). Sigh breaths are not recommended for managing infants with air leaks. Unilateral PIE may be managed by positioning the infant with the affected side down to minimize lung aeration to that side. Some severe cases have responded to selective bronchial intubation.
Management strategies for bronchopleural fistula have included conservative measures such as the insertion of one or more large caliber chest tubes to accomplish more effective drainage and allow lung healing. In general, ventilatory pressures are scaled back, and higher levels of P co 2 are tolerated.
Most air leaks will resolve spontaneously over a few days. Small tears or punctures usually heal quickly, whereas greater structural damage to the lung or a major bronchus may not heal with conservative management, particularly if high pressures are required for adequate gas exchange. Differential lung ventilation through double-lumen tubes has been used in some cases. For proximal leaks, fiberoptic bronchoscopy and direct application of sealants have been attempted with some success. Refractory cases have been managed surgically using thoracoplasty, lung resection/stapling, pleural abrasion/decortication, or other techniques.
Summary
During the past several decades, clinicians have translated many pharmacologic and device innovations to achieve improved early pulmonary outcomes and lower mortality of infants who have or are at risk for RDS. Unfortunately, the early hope that prevention or mitigation of lung disease caused by surfactant deficiency would lead to longer term improvements in respiratory outcomes such as BPD is unrequited. Increased success in the management of RDS has instead unmasked a much more complicated pathophysiology and biology of BPD. It is unlikely that any single new future therapy that will mimic the quantum improvement in early outcomes that surfactant achieved will soon be forthcoming. Continued progress in improving the survival and long-term respiratory and neurodevelopmental outcomes of extremely preterm infants will demand meticulous attention to continuous judicious respiratory management and to other nonrespiratory issues such as optimal management of the systemic circulation, enhanced nutritional therapy, and prevention of nosocomial infections.
REFERENCES
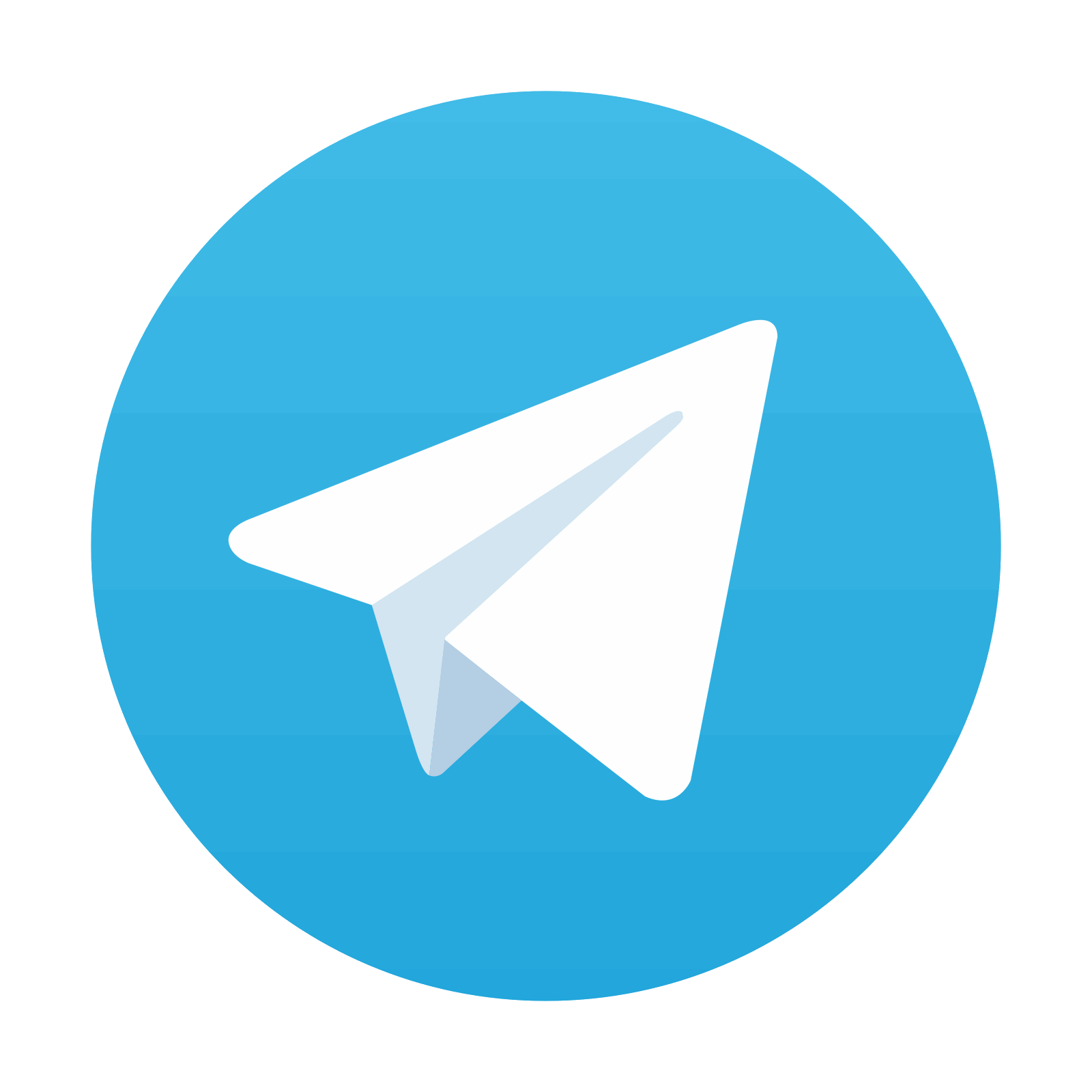
Stay updated, free articles. Join our Telegram channel

Full access? Get Clinical Tree
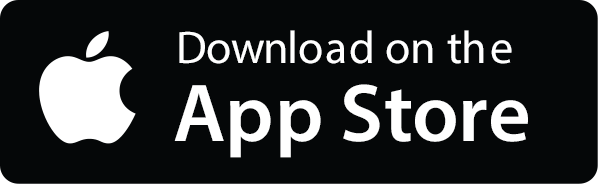
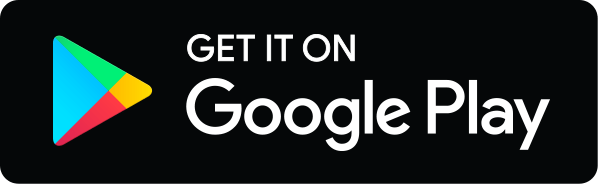
