Respiratory care encompasses a set of practices, usually implemented by a multidisciplinary team (physician, respiratory therapist, nurse), to ensure the optimal delivery of respiratory support to newborn infants with respiratory distress or other problems. These practices include resuscitation, artificial airway management, invasive and noninvasive monitoring of gas exchange, airway clearance, and aerosolized drug administration. A thorough understanding of these techniques will help clinicians provide optimal invasive and noninvasive respiratory support to sick neonates and simultaneously avoid iatrogenic injury such as skin breakdown, airway injury and inflammation, infection, ventilator-induced lung injury, and other complications. Unfortunately many of these interventions have not been rigorously studied, and the recommendations and suggestions in this chapter are often based on low-quality evidence and experience.
Techniques to Provide Positive-Pressure Ventilation
Manual Ventilation
Positive-pressure ventilation can be provided through a face mask or through an endotracheal tube either with a mechanical ventilator or with one of the three commonly used manual resuscitators: the self-inflating bag, the flow-inflating (“anesthesia”) bag, and the T-piece resuscitator. Each possesses inherent features that distinguish one from the other. The clearest differences are found in their ability to control ventilating pressures, their reliance upon a gas source, and their potential to deliver free-flow oxygen, continuous positive airway pressure (CPAP), positive end-expiratory pressure (PEEP), and sustained inflations.
Both flow-inflating and self-inflating bags come in a wide variety of configurations, but all configurations share some basic attributes, including an oxygen inlet, patient outlet, flow-control valve, and pressure manometer attachment site. The self-inflating bag, as the name implies, reinflates after squeezing and does not require the flow of oxygen to reinflate. However, this bag with an oxygen source can deliver only about 40% oxygen because as the bag reinflates, room air is drawn into the bag and mixes with 100% oxygen from the oxygen source. A reservoir will not allow room air to come into the bag; therefore, the self-inflating bag attached to an oxygen source with a reservoir is able to deliver 90% to 100% oxygen to the baby.
Two other important characteristics of most self-inflating bags are a pressure-relief (“pop-off”) valve, which is set at 30 to 40 cm H 2 O, and a nonrebreathing valve, which is built into the bag and prevents the reliable delivery of free-flow oxygen. To deliver free-flow oxygen, the operator needs to disconnect the oxygen tubing from the bag and hold the oxygen tubing close to the nose of the baby. In contrast, the flow-inflating bag is an excellent source of free-flow oxygen, especially with the use of the appropriate-sized mask attached to the bag. Finally, both of these bags require a pressure manometer to provide safe and effective ventilation to the newborn. Table 27-1 compares the two ventilation bags (or manual resuscitators).
Self-Inflating Bag | Non-Self-Inflating Bag | |
---|---|---|
Types | Laerdal, Hope II, PMR 2, and a host of disposable equivalents | “Anesthesia bag” with spring-loaded or variable-orifice bleed port |
Operator | Requires education on bag characteristics | Requires both experience and knowledge of bag characteristics for adjustment of flow and bleed |
Oxygen–air source positive FiO 2 delivery | Operates with room air | Requires compressed gas |
Efficacy of O 2 delivery dependent on correct use of closed reservoir system and closure of pop-off valve (use of open reservoir or pop-off valve reduces FiO 2 ) | Delivers FiO 2 of gas source unambiguously | |
Many brands deliver room air on spontaneous breaths (in-house verification of brand performance is recommended) | Oxygen delivery same on spontaneous breaths as it is on mandatory breaths | |
Pressure delivery | Having excessive trust in pop-off feature is unwise; occlusion of pop-off valve and use of manometer allow performance equal to that of non-self-inflating bags | With manometer attached, any pressure can be easily given |
Comments | Relatively complex mechanism with possibility of failure, particularly when reusable units are reassembled | Simple, reliable mechanism dependent on gas supply |
If pop-off pressure is adequate, allows removal of bulky manometer for transport | Manometer is bulky |
The self-inflating bag is the only resuscitator that can operate with or without a gas source, making it ideal for transport. Because of its design, however, it cannot deliver free-flow oxygen or “blow-by oxygen.” Some devices incorporate a reservoir hose coming from the back that can be used for this purpose. Improvements in sensitive valve mechanisms now allow spontaneous breathing and CPAP without having to squeeze the bag and at a relatively low work of breathing. Sustained inflations are not reliably given across all available models of self-inflating bags. PEEP can be delivered with a self-inflating bag but only with an adjunctive PEEP valve affixed to the exhalation port on the resuscitator. The self-inflating bag does not reliably control ventilating pressures and volumes even when the device is equipped with a manometer and pressure-relief valve.
A flow-inflating bag, as the name suggests, requires flow from a pressurized gas source to operate. Its design allows for free-flow oxygen delivery as well as CPAP during spontaneous breathing and PEEP during positive-pressure ventilation. Inspiratory pressure, PEEP, and/or CPAP is very difficult to maintain with these systems because the clinician must coordinate a mask seal and regulate egress of flow using the thumb valve while observing chest rise and pressure readings on a manometer. One study showed more excessive PEEP (defined as >10 cm H 2 O for >10 seconds) with the flow-inflating bag than with the self-inflating bag. Flow-inflating bags have traditionally been believed to be superior to self-inflating bags because they allow the clinician to feel changes in lung compliance better than with a self-inflating bag. Repeated laboratory scenarios have found that the flow-inflating bag produced more variable tidal volume and PEEP than the self-inflating bag. Studies have also shown that this sense of “feeling compliance changes” with a flow-inflating bag is less reliable than with a self-inflating bag. In fact, experienced physicians were unable to detect when an endotracheal tube was occluded in a lung model 75% of the time. Additionally, experienced respiratory therapists specializing in neonatology were shown not to distinguish changes in compliance better with a flow-inflating bag than with a self-inflating bag.
Most manual ventilation devices used during resuscitation have been shown to result in variability in delivered volumes and pressures. Displayed tidal volume permits better detection of compliance changes than monitored or preset pressures. Future devices are needed to display volume to avoid hypo/hyperventilation and lung injury in neonates.
The T-piece resuscitator is the newest and most sophisticated of the manual resuscitator designs. Similar to the flow-inflating bag, it requires a pressurized gas source to operate and can be used to administer free-flow oxygen and CPAP to a spontaneously breathing patient. Its greatest advantage lies in its ability to regulate ventilating pressures.
Face Masks for Ventilation
In cases in which the baby requires manual ventilation prior to endotracheal intubation, a ventilation bag with a manometer and the appropriate-sized mask should be used. The mask should be clear and have a soft, form-fitting cushion that extends around the circumference. The alternative is the rigid but anatomically shaped Rendell-Baker/Soucek mask, which may have less dead space but has been demonstrated to be more difficult to use, often resulting in ineffective ventilation.
Endotracheal Intubation
Endotracheal intubation is commonly required during neonatal resuscitation at birth or postnatally in an infant with apnea or severe respiratory failure. Certain anatomic lesions may cause obstruction at the level of the nasopharynx, larynx, and upper trachea and may necessitate endotracheal intubation of affected neonates during initial resuscitation. Beginning at the nasal level, these lesions include bilateral or severe unilateral choanal atresia or stenosis, pharyngeal hypotonia, and micrognathia, such as may be seen in the Robin sequence (which may include cleft palate and glossoptosis). At the level of the larynx, obstructive problems may include laryngomalacia (or laryngotracheomalacia), laryngeal web, bilateral vocal cord paralysis, and congenital subglottic obstruction. In addition, critical airway obstruction may be secondary to other lesions that may compress the airway and impair normal respiration. These may include cystic hygroma, goiter, or hemangioma. Many of these lesions, particularly those causing significant fixed obstruction at the level of the larynx or below, may render endotracheal intubation extremely difficult and may require emergency tracheostomy (see Chapter 25 ).
Infants in the neonatal intensive care unit may require endotracheal intubation and positive-pressure ventilation because of respiratory failure related to a variety of causes. Two common scenarios that merit particular consideration include preterm infants with worsening respiratory distress syndrome and infants with postextubation respiratory failure.
A variety of competing factors will influence the decision to intubate an infant who has worsening respiratory distress syndrome (RDS). The symptoms of untreated RDS will tend to worsen during the first 48 to 72 hours of life, until the infant begins to make significant amounts of endogenous surfactant. Therefore, an infant with moderately severe respiratory insufficiency and distress during the first 24 hours of life may merit intubation and ventilation in anticipation of worsening disease, whereas an infant with comparable disease severity at 3 or 4 days of life may avoid intubation in anticipation of spontaneous improvement.
Although the optimal timing of surfactant administration for treatment of RDS is controversial, the available data suggest that early treatment and multiple doses are more effective, and this observation may thus lead to earlier intubation. On the other hand, positive-pressure ventilation delivered through an endotracheal tube is well known to cause lung injury, particularly if large tidal volumes are used. Some centers that make extensive use of nasal CPAP to avoid intubation and mechanical ventilation have reported fewer apneic events, less need for (re)intubation, and low rates of chronic lung disease. However, these finding were not duplicated in randomized controlled trials. Different practitioners will weigh these competing factors differently, and the indications for intubation of an infant with RDS will vary depending on the clinical circumstances and local practices.
Postextubation respiratory failure is a common occurrence in preterm infants, occurring in as many as one-third of infants. Causes of respiratory failure in these infants include central or obstructive apnea, respiratory insufficiency leading to progressive atelectasis, and early chronic lung disease. While premature infants represent a large percentage of the patient population treated in the neonatal intensive care unit (NICU), term or postterm babies can become critically ill and develop life-threatening respiratory failure. This can result from congenital diaphragmatic hernia, primary pulmonary hypertension, meconium aspiration, sepsis, and pneumonia. Early application of nasal CPAP directly following extubation has been shown to result in lower incidence of respiratory failure, need for mechanical ventilation, and risk of developing bronchopulmonary dysplasia (BPD) in preterm neonates than extubation to no respiratory support, but CPAP may fail in 25% to 40% of infants. Some evidence suggests that intermediary forms of noninvasive ventilation (NIV) or noninvasive intermittent mandatory ventilation may be more effective in preventing postextubation respiratory failure. Other techniques to avoid reintubation include the use of methylxanthines. Indications for reintubation include progressive respiratory acidosis, increased work of breathing, grunting, stridor, significant oxygen requirement, or severe apnea.
It is not uncommon to have significant respiratory failure following extubation due to upper airway edema or subglottic stenosis, especially if the patient received prolonged ventilator support or multiple intubations or had traumatic intubation. Patients who develop respiratory failure following extubation often present with stridor, grunting, nasal flaring, and prolonged exhalation. These infants are at significant risk for severe clinical deterioration and cardiopulmonary arrest. Often, infants with a known risk of failed extubation attempts will be given intravenous steroids to reduce inflammation prior to extubation. Many clinicians will monitor the endotracheal tube leak displayed on the ventilator to determine the degree of airway edema present. However, this practice may not accurately reflect whether the neonatal patient will develop distress following extubation. Also, cuffed endotracheal tubes have been introduced into the NICU arena. Attempts should be made to reduce the cuff volume and prevent excessive pressure on the tracheal mucosae but only if the patient is able to be ventilated appropriately. In the event that there is airway compromise from edema following extubation, inhaled aerosolized racemic epinephrine can be delivered to reduce swelling, but there are no data to support or refute this practice. Nonetheless, many NICUs will have supplies ready at the bedside of high-risk neonates in the event that they develop stridor and respiratory distress. Additionally, clinicians should also have reintubation supplies ready at the bedside in case the patient develops severe respiratory failure. If the baby is unable to maintain adequate ventilation despite interventions, then reintubation and suctioning should be accomplished. There are multiple reasons for extubation failure; Box 27-1 provides a comprehensive list. Extubation failure should prompt a search for a cause that can be corrected before the next extubation attempt.
- I.
Pulmonary
- A.
Primary disease not resolved
- B.
Postextubation atelectasis
- C.
Pulmonary insufficiency of prematurity
- D.
Bronchopulmonary dysplasia
- E.
Eventration or paralysis of diaphragm
- A.
- II.
Upper Airway
- A.
Edema and/or excess tracheal secretions
- B.
Subglottic stenosis
- C.
Laryngotracheomalacia
- D.
Congenital vascular ring
- E.
Necrotizing tracheobronchitis
- A.
- III.
Cardiovascular
- A.
Patent ductus arteriosus
- B.
Fluid overload
- C.
Congenital heart disease with increased pulmonary flow
- A.
- IV.
Central Nervous System
- A.
Apnea (extreme immaturity)
- B.
Intraventricular hemorrhage
- C.
Hypoxic ischemic brain damage/seizures
- D.
Drugs (phenobarbital)
- A.
- V.
Miscellaneous
- A.
Unrecognized diagnosis (e.g., nerve palsy, myasthenia gravis)
- B.
Sepsis
- C.
Metabolic abnormality
- A.
Routes of Intubation
Intubation can be performed orally or nasally. The choice of route depends on the circumstances and the preference of the clinician. Both oral and nasal endotracheal intubation have their unique complications and share a few as well. Oral intubation is easier, faster, and less traumatic to perform, and it may be preferable in an emergency. Available data have failed to demonstrate statistically significant differences between oral and nasal intubation with respect to tracheal injury, frequency of tube retaping, or tube replacement. However, a higher incidence of postextubation atelectasis has been noted in nasally intubated patients, especially in preterm infants with birth weight less than 1500 g; atelectasis was associated with a marked reduction in nasal airflow through the previously intubated nares and stenosis of the nasal vestibule. Midface hypoplasia has been reported to be associated with long-term intubation for BPD.
On the other hand, proponents of nasal intubation believe that fixation of the tube to the infant’s face is easier and more stable because it minimizes the chance for accidental dislodgment and decreases tube movement, which can result in subglottic stenosis. Prolonged oral intubation can result in palatal grooving and defective dentition. Furthermore, there is evidence that acquired subglottic stenosis is increased in patients who were orally intubated and whose birth weight was less than 1500 g. The same study and one other offer evidence that the nasotracheal tube is easier to stabilize than an oral tube and that extubation occurs less frequently than in oral intubation. Acquired subglottic stenosis secondary to oral intubation may be a sequela of tracheal mucosal damage from the endotracheal tube itself or from repeated intubations. Most significantly, severe damage can occur from the up-and-down movement of the endotracheal tube. Even with perfect fixation of the tube, up-and-down movement of 7 to 14 mm has been reported owing to the varying degrees of flexion of the neck. The caretaker team can minimize palatal grooving and defective dentition by rotating the fixation site from side to side during periodic retaping. Devices are available commercially that serve as palate protectors for prolonged intubation of very low birth-weight infants (Gesco Pla-nate, MedChem Products, Woburn, Massachusetts, USA). Continuing attention to the quality of fixation, together with stabilization of the infant’s head position, minimizes tube shifting and accidental extubation with the oral approach. However, both the oral and the nasal techniques will continue to have a place in the care of the ventilated neonate. Problems associated with oral and nasal endotracheal tube use are summarized in Box 27-2 .
Common Problems
- •
Postextubation atelectasis—more common with nasal endotracheal tubes
- •
Pneumonia/sepsis
- •
Accidental extubation
- •
Intubation of main stem bronchus
- •
Occlusion of tube from thickened secretions
- •
Tracheal erosion
- •
Pharyngeal, esophageal, tracheal perforation
- •
Subglottic stenosis
Problems Unique to Nasal Endotracheal Tubes
- •
Nasal septal erosion
- •
Stricture of the nasal vestibule
Problems Unique to Oral Endotracheal Tubes
- •
Palatal grooving
- •
Interference with subsequent primary dentition
Equipment
The equipment needed for intubation is listed in Box 27-3 , and the guidelines for choosing the correct tube size and suction catheters are listed in Tables 27-2 and 27-3 .
- •
Laryngoscope with premature (Miller No. 0) and infant (Miller No. 1) blades; Miller No. 00 optional for extremely premature infant
- •
Batteries and extra bulbs
- •
Endotracheal tubes, sizes 2.5, 3.0, 3.5, and 4.0 mm i.d.
- •
Stylet
- •
Suction apparatus (wall)
- •
Suction catheters: 5.0, 6.0, 8.0, and 10.0 F
- •
Meconium aspirator
- •
Oral airway
- •
Stethoscope
- •
Non-self-inflating bag (0.5 L), manometer, and tubing; self-inflating bag with reservoir, manometer optional for self-inflating bag
- •
Newborn and premature mask
- •
Source of compressed air/O 2 with capability for blending
- •
Humidification and warming apparatus for air/O 2
- •
Tape: ½-inch pink (Hy-Tape)
- •
Scissors
- •
Magill neonatal forceps
- •
Elastoplast (elastic bandages)
- •
Cardiorespiratory monitor
- •
Carbon dioxide monitor or detector
- •
Pulse oximeter (SpO 2 )
Tube Size (Inside Diameter in mm) | Weight (g) | Gestational Age (week) |
---|---|---|
2.5 | <1000 | <28 |
3.0 | 1000-2000 | 28-34 |
3.5 | 2000-3000 | 34-38 |
3.5-4.0 | >3000 | >38 |
Endotracheal Tube Size (mm) | Catheter Size (French) |
---|---|
2.5 | 5 or 6 |
3.0 | 6 or 8 |
3.5 | 8 |
4.0 | 8 or 10 |
The use of tubes of appropriate size minimizes trauma, airway resistance, and excessive leak around the tube. A standard kit containing all of the equipment, as listed in Box 27-3 , can be prepared and stocked, but it must be checked regularly to ensure that all of the necessities are present. The infant should be placed under a radiant warmer for endotracheal intubation. A laryngoscope with a Miller No. 0 or No. 1 blade should be used to visualize the vallecula, epiglottis, and glottis. The No. 0 blade is used for almost all newborns. The No. 1 blade is used for infants who are several months of age or newborns whose birth weight is greater than 4 to 5 kg. A Miller No. 00 blade has been touted for use in extremely low birth-weight infants because its smaller blade is more easily accommodated in the mouths of micropreemies. However, because the light source is set back farther from the blade tip, some clinicians believe the visualization is not as good as with the No. 0 blade.
Types of Tubes
The endotracheal tube should be made of a nontoxic, thermolabile, nonkinking material that molds to the airway. The tube should meet the standards of the American Society for Testing and Materials F1242-89 and be radiopaque or have a radiopaque line. Cuffed endotracheal tubes are not routinely used in neonates because the bulk of the cuff may prevent the practitioner from inserting a tube with as large a diameter as would otherwise be possible. There is always a serious concern that the inflated cuff may damage the very sensitive airway mucosa of the small baby. If sealing the space around the tube becomes a priority, cuffed tubes are now available (Sheridan, Teleflex, Morrisville, North Carolina, USA).
The type of endotracheal tube used most commonly is the Murphy endotracheal tube ( Fig. 27-1 ). The Murphy tube is preferred for long-term ventilation. Most often, Murphy tubes have centimeter markers to show the overall depth of the tube, as well as vocal cord guide markers near the tip. These markers, under laryngoscopic visualization, show the clinician the depth within the trachea. Standard default markers should be used with caution because of the range of anatomic variation. In one review of the length of the black area at the tip of endotracheal tubes produced by four major manufacturers, the marker length varied by 10 mm in 2.5-mm internal-diameter tubes.

A Murphy tube has a tip bevel that allows smooth passage through the nares and a side hole whose purpose is to allow ventilation even if the tip is partially obstructed or is placed in the right main stem bronchus. Some clinicians avoid using side-hole (“Murphy eye”) tubes for prolonged ventilation because of anecdotal evidence that these tubes can abrade the trachea and cause scarring. Exclusive use of these endotracheal tubes in one institution was associated with an increased incidence of subglottic stenosis that ended when use of the tubes was discontinued. It can be adequately maintained in the correct position if the lip marker is placed on the tube at the lip level and it is fixed to the face. After proper placement is determined, the marker can be used as a reference to ensure that the tube’s position remains constant. The Murphy tube is pliable (and becomes even less firm when it is allowed to remain under a radiant warmer while preparations are made for resuscitation). Many clinicians prefer to use an obturator or stylet to facilitate insertion. The stylet should not extend beyond the distal tip of the tube to avoid tracheal damage from the insertion process.
The vicious cycle of asphyxia is frequently in progress in the critically ill neonate who requires emergent tracheal intubation. The process of intubation in such an infant can exacerbate the difficulties that he or she is already experiencing. Intubation is associated with severe bradycardia, hypoxia, and elevation of arterial blood pressure and intracranial pressure.
Depth of Tube Insertion
In addition to direct visualization of the tube as it passes through the glottis, there are a number of suggested “rules of thumb” for initial estimation of proper depth of tracheal tube placement. These rules use the centimeter markings on the side of a standard Murphy tube to gauge the depth of placement. The most common rule uses birth weight and a simple formula, the rule of 7-8-9. An endotracheal tube is advanced 7 cm to the lip for a 1-kg infant, 8 cm for a 2-kg infant, and 9 cm for a 3-kg infant. The rule of 7-8-9 is not appropriate for infants with hypoplastic mandibles (e.g., those with Pierre Robin syndrome) or short necks (e.g., those with Turner syndrome). While the 7-8-9 rule appears to be an accurate clinical method for endotracheal tube placement in neonates weighing more than 750 g, when applied to infants weighing <750 g, caution is warranted. This may lead to an overestimated depth of insertion and potentially result in clinically significant consequences. Nasotracheal tube insertion is generally governed by adding 1 cm to the 7-8-9 rule.
Determination of Placement
Placement of the endotracheal tube after intubation is determined first clinically and then by chest radiograph. Clinical determination includes the following:
- •
Improvement or maintenance of heart rate in the normal range
- •
Good color, pulses, and perfusion after the intubation
- •
Good bilateral chest wall movement with each breath
- •
Equal breath sounds heard over both lung fields
- •
Breath sounds heard much louder over the lung fields than are heard over the stomach
- •
Presence of inspiratory and expiratory tidal volume measurements
- •
No gastric distention with ventilation
- •
Presence of vapor in the tube during exhalation
- •
Direct visualization by laryngoscope of the tube passing between the vocal cords
- •
Presence of exhaled CO 2 as determined by a CO 2 detector and/or an end-tidal CO 2 monitor or capnography
- •
Tip-to-lip measurement: Add 6 to the newborn’s weight in kilograms (rule of 7-8-9)
The chest radiograph should be performed to demonstrate that the tube is in the mid trachea. Tube position can change during the X-ray procedure if the infant’s neck is in a flexed or extended position. Endotracheal tube (ETT) position can be confirmed on X-ray by following both of the main stem bronchi back to the carina and cephalad to the tip of the tube. Occasionally a lateral radiograph is necessary to confirm placement in the trachea. Only one study has been conducted to compare differences in outcomes related to radiographic placement of the ETT. They concluded that the ETT tip should be kept at the level of the first or second thoracic vertebrae in extremely premature babies to reduce the incidence of nonuniform lung aeration and adverse pulmonary outcomes.
Tube Fixation
Secure fixation of the ETT is important, not only to prevent accidental extubation but also to minimize tube movement during ventilation and other interventions such as suctioning, chest physiotherapy (CPT), surfactant administration, and positioning the patient. An insecure fixation of the tube is one of the most common reasons accidental extubation can occur. Accidental extubation and repeated intubations have been demonstrated to be associated with the development of subglottic stenosis, as well as increased mortality. The likelihood of accidental extubation also has been associated with younger gestational age, higher level of consciousness, higher volume of secretions, and slippage of the tube. It also is clear that there is no consensus as to which tube fixation method is most effective. The technique shown in Figure 27-2 represents a modification of the method originally described by Gregory. Another approach that was initially described by Cussel et al. is to secure an umbilical clamp with a drilled hole in the center with tape to the face. Several modifications have been described using this technique. Compared with standard taping to the upper lip, methods using a modified umbilical clamp have been shown to substantially reduce unplanned or accidental extubation in neonatal patients ( Fig. 27-3 and Table 27-4 ).


|
Other studies have compared standard taping to approved commercially available fixation devices, and the ETT location was shown to be directly attributable to the type of fixation being used. Another important development is that tincture of benzoin is no longer used, especially in extremely low birth-weight infants (micropreemies). Also, some of these techniques can be used to secure nasotracheal tubes ( Fig. 27-4 ) without the use of tincture of benzoin. Several devices for fixation of neonatal ETTs and adhesive materials are available from various manufacturers. (See also discussion in Chapter 7 .)

Acquisition and Maintenance of Intubation Skills
Intubation of the trachea is a complex psychomotor skill taught to a variety of health care professionals. Although ventilation can be accomplished successfully using a bag and mask, there are many instances in which neonatal tracheal intubation is required. The challenge is maintaining a high skill level for a procedure that may be performed only sporadically by individual providers.
Depending on the clinical setting, intubation skill may be required of attending physicians, residents, nurses, respiratory therapists, and paramedics. Institutional or departmental policies may require or expect that certain individuals be proficient at intubation yet may be unable to provide opportunities to maintain proficiency. The challenge is that, without regular practice, individual intubation skill level decreases over time, and the complications from an unskilled intubation may be severe.
Initial training in intubation often occurs in a clinical skills lab using plastic manikins made specifically for the purpose of intubation. This is typically the first exposure to the skill of intubation for medical students as well as for other disciplines. Courses such as the Neonatal Resuscitation Program and the Pediatric Advanced Life Support course include intubation education, practice, and testing on a manikin. The fact that students are “tested” on their ability to intubate a plastic manikin airway model may lead some to think that they are now “proficient.” It is important to emphasize that such courses provide only limited exposure to the intubation skill and that the ability to intubate a plastic manikin does not immediately translate to the bedside. However, improvements in the manikin, especially the anatomy and “feel” of the airway, have made this simulation experience more readily transferable to actual clinical situations.
Studies have shown that, over time, cognitive knowledge remains but the actual hands-on skill level declines and that ongoing review and proctored skills practice are needed to maintain a level of proficiency. A 10-year study of neonatal intubations performed by pediatric residents at one institution showed that median success rates varied from 33% for PL 1 residents to 40% for PL 2 and PL 3 residents. Success rates for residents with greater than 20 intubation attempts was 49%, whereas those residents with fewer than 20 attempts had a 37% success rate. These same pediatric residents may go on to take positions in which they are expected to and need to have intubation proficiency, yet the study showed that they did not have the opportunity during their training to achieve a high level of success at the procedure. Another study found that, although pediatric residents stated that they felt confident with neonatal intubation skills, objective findings showed that they did not meet the study-specified definition of technical competence.
Neonatal and pediatric transport teams typically use a combination of registered nurses, respiratory therapists, paramedics, and physicians, and there are many teams that run primarily as nurse–therapist or nurse–paramedic. Because team members are expected to perform advanced level skills, team training generally includes skills such as intubation, umbilical line, and needle aspiration/chest tube insertion. In addition to a didactic and skills practice orientation, transport team members should attend regular update and competency sessions. Experts recommend that team members perform a minimum number of transports to maintain skills and, if the number of transports is low, there be other mechanisms to simulate transport team function.
Since 1990 there have been many changes related to the indications for intubation of the neonate. Historically, all newly born infants with meconium staining of the amniotic fluid require intubation and suctioning. The latest Neonatal Resuscitation Program (NRP) recommendations call for intubating only those infants with meconium staining who are not vigorous. Newer oxygen delivery methods, such as high-flow nasal cannula and nasal CPAP, allow more infants to be cared for without the need for intubation. Ready availability of high-risk perinatal units and NICUs ensures that more critically ill neonates are born at a center with high-level skills. All of these advances are good for the neonate, but they have unfortunately resulted in a decreased number of intubations available for pediatric residents and other practitioners, necessitating alternate methods to ensure that competence in airway management is maintained.
Health care educators need to be creative in providing the initial intubation education and also in monitoring and facilitating the continuing education of those individuals expected to respond to a neonatal emergency. A blended learning approach can integrate online learning with supervised manikin practice. Although expensive, animal intubations (usually cats) can provide an excellent practice model but must be done adhering to the NIH Office of Animal Care and Use guidelines. The airline industry has long been using simulators for initial training and for continuing education and competency evaluation. The simulator manikin setup is expensive but provides an excellent learning model that functions in real time. Patient simulations are generally enjoyed by students (generic and professional) and are perceived by the students to be of benefit. The newest neonatal simulator manikins, although expensive, provide an excellent resource for this training.
Looking to the future, educators should consider the use of virtual reality simulation. Virtual reality simulators are available to teach trauma assessment and skills and also diagnostic bronchoscopy. In one study the virtual reality bronchoscopy simulator was used to train new students in doing a diagnostic bronchoscopy. With minimal time practicing on the simulator, the new students were able to attain a level of proficiency similar to that of more experienced bronchoscopists. Virtual reality simulation can be used for initial education and practice and at regular intervals to reinforce skills.
Laryngeal Mask Airway
The laryngeal mask airway (LMA) has been available for a number of years as an alternative to endotracheal intubation in babies, infants, children, and adults. It is mentioned but not recommended for routine use in the new NRP textbook, and a variety of papers discuss its use in various clinical scenarios, including the following:
- •
In neonatal resuscitation of term and larger preterm infants (size 1 LMA)
- •
In the difficult airway, such as in the Robin sequence, and other situations when micrognathia is profound
- •
As an aid to endotracheal intubation
- •
As an aid in flexible endoscopy
- •
In surgical cases in place of endotracheal intubation
The success rate of insertion of the LMA has been reported to be greater than 90% in a number of descriptive studies of small series of infants and children (see Chapter 4 for further discussion of LMA use).
Noninvasive Ventilation and Continuous Positive Airway Pressure
Avoiding ventilator-induced lung injury is a common goal of neonatal intensive care and has led to considerable interest in less invasive means of providing effective respiratory support. Neonates who might require respiratory support short of intubation and mechanical ventilation include those with apnea of prematurity, mild to moderate RDS, and atelectasis caused by respiratory insufficiency, as well as recently extubated infants at risk for postextubation respiratory failure. Nasal CPAP has traditionally been a widely used support modality in these infants. It has the advantage of being well studied and is known to improve pulmonary mechanics and to stabilize the upper airway. The use of CPAP and noninvasive ventilation is discussed in more detail in Chapter 17 .
Heated Humidified High-Flow Nasal Cannula
Humidified high-flow nasal cannula (HHFNC) devices have come into widespread use in NICUs. These devices differ from traditional nasal cannula therapy in that the gas flow to the patient is warmed and humidified up to the point of patient contact, allowing the use of higher gas flows without causing nasal drying, mucosal trauma, and patient cooling. Gas flow rates used in neonatal HHFNC therapy may range between 2 and 8 L/min. The higher gas flows that can be attained with HHFNC have led many to view this therapy as a viable alternative to nasal CPAP that is less bulky and easier to maintain.
The level and consistency of CPAP that can be attained with HHFNC have been examined in several small case series reports, with variable results. There is general agreement that HHFNC can produce a clinically significant level of CPAP, particularly at higher flow rates. However, several variables appear to play an important role in determining the level of CPAP attained, including patient size, cannula diameter, and whether the mouth is open or closed. In some instances, particularly when the nasal cannula completely occludes the nares, dangerously high levels of CPAP may be produced. It should be noted that, unlike nasal CPAP devices, HHFNC devices at this time do not incorporate a safety pop-off valve in their design, raising the possibility that very high pressures could be transmitted to the lungs. Until better safety and efficacy data are available, HHFNC should be used with caution and not be viewed as a substitute for nasal CPAP devices.
Monitoring during Respiratory Support
Monitoring during Noninvasive Respiratory Support
Infants are supported with noninvasive respiratory support using nasal CPAP (NCPAP) or NIV to prevent intubation or following weaning and extubation from invasive support. Such patients may require more attention at the bedside than a patient who is being ventilated invasively. Patients receiving this form of support do not have accurate or reliable mechanics or tidal volume measurements. Also, many of these devices are limited by a lack of alarms. As such, ongoing assessment, gas exchange, and evaluation of work of breathing are vital to the management and success of this form of support. Patients receiving noninvasive approaches can be instrumented with physiologic monitoring to include SpO 2 , transcutaneous CO 2 , heart rate, and blood pressure. Routine blood gases are not frequently obtained and are reserved for situations in which the patient has developed clinical deterioration and for correlating values with noninvasive monitoring. Chest X-rays are a poor surrogate for determining lung volumes during noninvasive support. Unfortunately, there are no approved devices for monitoring inspiratory and end-expiratory lung volumes. Novel technology using electrical impedance tomography may prove to be useful once it has been developed for newborns. Because of the lack of physiologic monitoring, many institutions have embraced respiratory scoring tools (i.e., Silverman–Anderson Respiratory Severity Score) to guide clinical management. These scores have been shown to have good interrater reliability among clinicians and can be useful for determining when the patient requires support or ongoing settings adjustments and for weaning.
Airway management is a time-consuming endeavor but perhaps the single most important aspect for improving outcomes and reducing complications in infants receiving noninvasive support. This detailed approach is more art than science, and the increased use of noninvasive support since 2005 has provided new experience that is summarized in some excellent resources. Many neonates are being supported for weeks and sometimes months with this support. Briefly, clinicians caring for infants receiving CPAP should select prongs of the optimal size and ensure that the prongs are not displaced. Prongs that are too small increase the resistance to gas flow and work of breathing and are associated with excessive leaks. An optimally sized prong fills the entire opening without blanching the external nostril. The infant’s nostrils should be suctioned periodically, and the nasal airway evaluated for skin breakdown. There are many approaches that are used to prevent nasal breakdown, including (1) alternating between nasal prongs and mask, (2) nasal barrier devices, and (3) nasal airway interfaces/fixation that are less likely to cause sustained pressure on the nasal airway.
As mentioned previously, many noninvasive devices do not provide clinical monitoring of pressure or alarms. Disconnection of the patient from the device (e.g., dislodged prongs) may not result in an alarm to indicate low pressure. Two such devices, bubble NCPAP and HHFNC, do not have integrated alarms as a standard system component. Thus, it is important to provide continuous physiologic monitoring. In a bench model, condensation forming in the expiratory limb of a commercially available bubble NCPAP system resulted in substantially higher CPAP levels than desired. Whenever possible, stand-alone pressure manometers, alarms, and pressure-relief devices should be used. Also, bedside clinicians must provide measures to frequently empty the exhalation limb of condensate, provide water traps, or use circuits that incorporate heated wires or are constructed from material that wicks moisture to the environment.
Monitoring during Conventional and High-Frequency Ventilation
Electrocardiography, blood pressure, and serial arterial and/or capillary blood gases have been the traditional mainstays of bedside monitoring of the newborn, and they still have an important role. In general, the emphasis on noninvasive monitoring has resulted in the development and availability of new technologies that allow close monitoring without invasive procedures. The following is a list of those instruments:
- •
Transcutaneous monitoring of P o 2 and P co 2
- •
Pulse oximetry to provide continuous measurement of hemoglobin saturation with O 2
- •
End-tidal CO 2 monitoring
See Chapter 7 , Chapter 17 for a more in-depth discussion of these noninvasive monitoring techniques.
Nearly all of the neonatal mechanical ventilators that are currently available provide airway graphics monitoring. A complete understanding of airway graphics can be vital for determining response to different therapies (i.e., surfactant and bronchodilators), clinical improvement and deterioration, equipment malfunction, and appropriate setting changes. In the NICU, there is also the additional difficulty of dealing with ETTs that are uncuffed, leading to large positional leaks around the ETT, which can lead to autocycling and missed trigger attempts by the patient. Assessing airway graphics can be useful, in cases such as these, for providing improved patient comfort. Airway graphics and tidal volume monitoring are best accomplished using a proximal flow sensor in neonates. Tidal volume monitoring and targeting have steadily become a widely accepted practice in most NICUs. Seminal animal studies have shown that ventilation for 15 minutes with a volume of 15 mL/kg has been shown to cause lung injury and as few as three overdistending breaths at birth have been shown to compromise the therapeutic effect of subsequent surfactant replacement. Conversely, small or inadequate volumes can also cause lung injury. Tidal volume is also an important measurement in volume-targeted ventilation. Compared with pressure ventilation, volume-targeted ventilation has been shown to reduce pneumothorax, days of ventilation, hypocarbia, periventricular leukomalacia or grade 3-4 intraventricular hemorrhage, and combined outcome of death/BPD. Monitoring expiratory volumes at the level of the ventilator valves may overestimate tidal volume delivery and reduce the accuracy of airway graphics, despite attempts to adjust for circuit tubing compliance with the ventilator. Proximal flow sensors are prone to accumulating secretions, malfunctioning, creating torque on the ETT, and adding mechanical dead space. The benefit of a flow sensor outweighs all of these risks. Flow sensors may not accurately predict the delivered volumes when tube leaks are present but measured expiratory volume is usually more accurate than inspiratory tidal volumes for predicting the delivered volume in these cases. Appropriate bedside maintenance of flow sensors, calibration, and standardized cleaning procedures are important clinical quality measures.
For infants on high-frequency ventilation (HFV), pulmonary care involves new technology and keen observation. These critically ill babies require a definite team approach, including an experienced respiratory therapist and nurse, and the traditional tools, including cardiorespiratory monitoring, intermittent arterial blood gases (from an arterial line), and “wiggle” assessment. A sample of a protocol used in the infant special care unit at one institution includes the following:
Assessments every hour:
- •
Vital signs from monitors, including heart rate, arterial blood pressure, body temperature
- •
Vibration (or wiggle) assessment (scale +1 to +3)
- •
Capillary refill
- •
Comfort level
Assessments every 4 hours—“hands-on assessment”:
- •
Auscultation of breath sounds on oscillator
- •
Palpation of pulses
- •
Nasogastric tube placement can be assessed without having to take the baby off of the ventilator
Assessments every 8 hours—ventilator is turned off but the patient remains on the circuit or backup rate (high-frequency jet ventilation):
- •
Heart rate, position of point of maximum intensity of heart, presence or absence of a heart murmur
- •
Bowel sounds
Other assessments:
- •
Arterial blood gases after initiation of HFV: hourly for 6 hours, every 2 hours for 6 hours, and every 4 hours and as needed thereafter
- •
Tidal volumes (when available)
- •
Chest radiograph schedule: just prior to being placed on HFV, within 1 hour of initiation of HFV, every 12 hours twice, and then daily and as needed
- •
Continuous monitoring of oxygen saturation using the pulse oximeter
- •
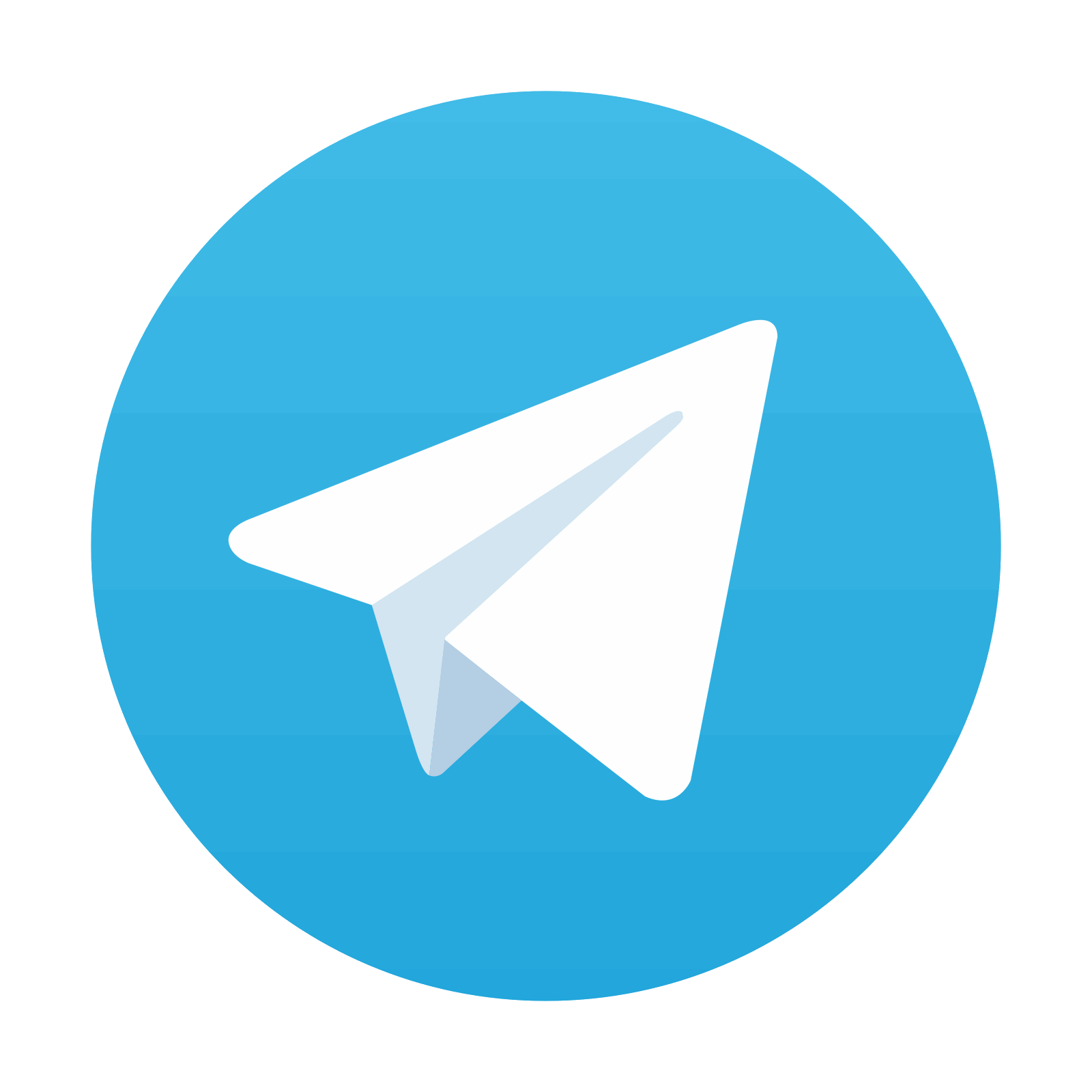
Stay updated, free articles. Join our Telegram channel

Full access? Get Clinical Tree
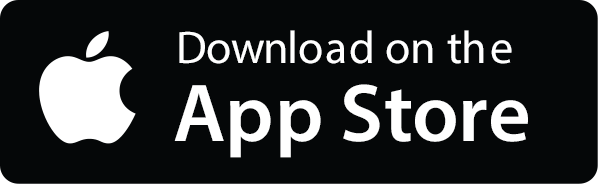
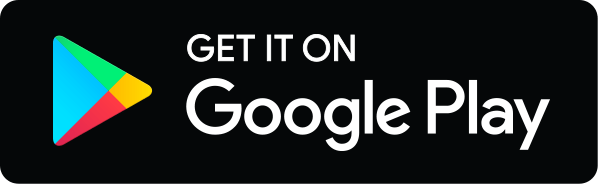