Initiation
Ideally, VG should be initiated as soon as possible after intubation because this is the time when the most rapid changes in lung mechanics are likely to occur
There are two basic ways to initiate VG. If the patient is already mechanically ventilated with PLV and has good gas exchange, it is reasonable to select a target V T based on the observed V T during PLV and then increase the PIP limit by about 20 % from baseline to allow the microprocessor to adjust the PIP up and down as needed
The alternate method when starting VG as the initial mode is to select the starting V T based on the particular patient’s characteristics and select the inflation pressure limit 3–5 cmH2O above the level estimated to be sufficient to achieve a normal V T in that infant
If the target V T cannot be reached with this setting, the pressure limit should be increased until the desired V T is generated, but excessive pressures must be avoided. It is important to make sure the endotracheal tube is not kinked, malpositioned in the mainstem bronchus, or obstructed on the carina. Significant volutrauma and/or air leak could result from failure to recognize single-lung intubation with any modality of VTV
The usual starting target V T for infants 500 g–1,000 g is 4.5–5 mL/kg during the acute phase of RDS. Larger infants with RDS only need 4–4.5 mL/kg, but infants with MAS may require slightly larger V T (5–6 mL/kg) due to the larger alveolar dead space related to heterogeneous inflation and air trapping (American Association for Respiratory Care 1992)
All of these values were derived from VG use in combination with A/C or PSV. If using SIMV at rates <40 the V T targets may need to be slightly larger to achieve the same minute ventilation
Larger tidal volumes (as much as 6–8 mL/kg) are needed in older infants with chronic lung disease because of increased anatomic and physiologic dead space as a result of dilated large airways and wasted ventilation resulting from air trapping and heterogeneous aeration (Stark et al. 1979)
The PIP should be set about 20 % higher than the working pressure (the PIP currently needed to deliver the target V T) to give the device adequate room to adjust PIP but low enough for optimal safety
The working pressure, which is the true reflection of the level of support the infant is receiving must be recorded, in addition to the PIP limit
Caution should be exercised when administering surfactant during VG. Under normal circumstances the working pressure increases as needed in response to the transient small airway obstruction caused by surfactant and maintains normal gas exchange. However, if the ventilator senses complete ETT obstruction, it will drop inflation pressure midway between PIP limit and PEEP as a safety measure. If this is not recognized, significant deterioration may occur (Nikischin et al. 1996)
Subsequent adjustments
Subsequent adjustment to the target V T can be made based on PaCO2, persistent tachypnea, or signs of increased WOB. The usual increment is 0.5 mL/kg
Although adjustment is seldom necessary when the initial value is chosen carefully, it should be understood that the suggested V T settings are only the typical values. Individual patients may have different needs, based on factors such as larger than normal physiologic dead space due to excessively high PEEP leading to overexpansion, the presence of metabolic acidosis (pH, not PaCO2 drives the respiratory control), or increased CO2 production, such as may occur with fever or sepsis
The PIP limit needs to be adjusted from time to time to keep the PIP limit sufficiently close to the working pressure (remember that inflation pressure will default to the PIP limit if the flow sensor is removed, malfunctions for any reason, or with “manual inflation”), but high enough to avoid frequent alarms. In most infants, keeping the pressure limit 4–6 cmH2O above average working pressure is appropriate. Note: Ideally, when removing the flow sensor for significant periods, such as when nebulizing medications, the PIP limit should be adjusted to roughly match the average or recent working pressures
If the infant appears agitated, with episodes of spontaneous hyperventilation, optimize positioning, limit noise, and consider light sedation (avoid oversedation, with complete suppression of respiratory effort)
If the PaCO2 is too low despite V T set at the low end of normal, make sure there is no substantial leak around the ETT (or make sure the leak-compensated V T is being used with the VN 500). Other causes of hypocapnia may be agitation and low PEEP causing the infant to be tachypneic (shorten expiratory time) in an effort to maintain lung volume. In extremely small infants, PSV may lead to excessive tachypnea due to their very short time constant – A/C may be preferred in the first few days of life
If the infant has persistent tachypnea or is consistently breathing above the set V T, the WOB will be excessive. Consider increasing the V T target even if the PaCO2 and pH are adequate. However, if the PaCO2 is low and the respiratory rate is high, comfort measures or sedation may be indicated
If the low V T alarm sounds repeatedly, increase the pressure limit to allow the device to reach the desired V T. Repeated alarms suggest that there has been a change in lung mechanics or patient respiratory effort (e.g., atelectasis, pneumothorax, pulmonary edema, right mainstem bronchus intubation, patient becoming septic, respiratory depression from narcotics). This early warning system is an important benefit of the VG mode and should not be ignored, but it will not be effective if the PIP limit is set too high
If the pressure limit has to be increased substantially, verify that the V T measurement is accurate (assess chest rise, obtain a blood gas), seek the cause of the change in lung mechanics (examine the patient, verify ETT position, obtain a chest x-ray and blood gas), and evaluate spontaneous respiratory effort
Weaning from mechanical ventilation
Weaning from VG does not require lowering of the target V T, because the physiologic V T does not decrease – what decreases is the pressure needed to generate that V T
When the target V T is set at the low end of the normal range (but generally not <4 mL/kg) and PaCO2 is allowed to rise enough to have a pH <7.35, weaning occurs automatically, as the infant should have a good respiratory drive and gradually takes over the work of breathing
For infants who have chronic lung disease, a higher V T should be used even during weaning because of the increased physiologic dead space. Automatic weaning occurs as long as the pH is low enough to give the infant good respiratory drive
If the V T is set too high, resulting in pH ≥7.4, the baby will not have a respiratory drive and will not “self-wean.” Instead, the baby will become dependent on the ventilator due to lack of respiratory muscle training
If the set V T is too low, there will be increased WOB with eventual respiratory deterioration. Working pressure at or near the level of PEEP indicates that the set V T is below the infant’s physiologic need. If the infant is not tachypneic and without retractions, extubation is indicated. If the opposite is true, V T should be increased to provide adequate support and avoid fatigue
To ensure good respiratory effort, sedation should be avoided during the weaning phase
If an infant appears to not be weaning as expected, despite apparently improving lung disease, try lowering V T by 0.5 mL as long as blood gases are adequate and the work of breathing does not appear excessive.
If significant oxygen requirement persists, PEEP may need to be increased to keep mean airway pressure from falling excessively as PIP is automatically lowered. Failure to do so may result in atelectasis, worsening lung compliance, and failure of extubation
Most infants can be extubated when they consistently maintain V T at or above the target value with delivered PIP <10–12 cmH2O (<12–15 cmH2O in infants >1 kg) with FiO2 <0.35 and sustained respiratory effort
Observing the graphic display of the working PIP is helpful in assessing for periodic breathing (variable respiratory effort) and apnea that may presage failure of extubation. Lowering the backup rate of A/C or PSV to 20 bpm for a few hours may help unmask inconsistent respiratory effort
Due to the limits of volume control discussed above, increasingly, PCV is utilized as a protective lung strategy, particularly in patients with acute lung injury (ALI) and acute respiratory distress syndrome (ARDS) (Prella et al. 2002). In neonates, there is a growing acceptance of combining pressure control with synchronized intermittent mandatory ventilation (SIMV) to offer the advantages of pressure control and the synchronization of SIMV. Pediatric patients with ALI or ARDS have a higher proportion of diseased, collapsed, or consolidated lung regions that take a longer time to open, and thus may require a longer T i. During PCV, the inspiratory pressure and time are set by the clinician, and the ventilator adjusts the inspiratory flow to achieve and maintain the set inspiratory pressure based on the compliance and resistance of the patient. Setting adequate T i is essential in all of the pressure control-style modes to enable the preset pressure to reach both healthy and diseased lung units (Marik and Krikorian 1997).
Due to the identified disadvantages of volume- and pressure-controlled modes, dual control modes, which allow the clinician to set a volume limit breath during a pressure-controlled breath, have been developed to provide the benefits of both volume and pressure control while avoiding the shortcomings of single control modes. Dual control modes include complex closed-loop systems that switch between pressure control and volume control either within a single breath or between individual breaths based on measured patient characteristics. In the first sections of this chapter, we will discuss common control modes of ventilation including assist control (A/C), pressure control, and volume control. Our discussion will focus on the technical aspects of each mode and their advantages and disadvantages in neonatal and pediatric patients. In the subsequent sections, we will focus on dual control modes as an alternative to traditional control modes and finally a discussion of support modes.
21.1 Assist-Control Ventilation/Intermittent Positive Pressure Ventilation
21.1.1 Principles
Assist-control ventilation, also known as patient-triggered ventilation and synchronized intermittent positive pressure ventilation, is a ventilator mode where every spontaneous inspiratory effort is assisted by a positive pressure breath triggered in synchrony. In the absence of spontaneous breathing efforts or if these are insufficient to trigger a mechanical cycle, the ventilator automatically initiates a “controlled” or backup ventilator rate of intermittent mandatory ventilation (IMV) type of breaths. The concept behind the assist-control modality is that it attempts to guarantee a baseline V E by means of a targeted V T along with a predefined respiratory rate (Mador 1994). In addition, the patient is given the opportunity to exceed this prescribed V E, if he desires, through his own spontaneous breathing efforts. Thus, the ventilator will control ventilation to a certain level, but has the flexibility to enable the patient to exceed the set targets. The “control” targets can be volume (volume control), pressure (pressure control), or volume target with pressure titrated (volume control plus or pressure-regulated volume controlled).
Incorporation of the Ayre’s T-piece principle to neonatal ventilators enables maintenance of a continuous flow of gas in the breathing circuit (bias flow), which is used to generate pressure at the airway opening and to remove exhaled gases (Kirby et al. 1972). Partial restriction of the bias flow by an expiratory valve increases airway pressure to the set positive end-expiratory pressure (PEEP), which is aimed at maintaining a continuous distending pressure to prevent alveolar collapse and maintain lung volume. This is particularly important because the endotracheal tube bypasses the upper airway, which prevents the glottis closure mechanism for maintenance of lung volume. The expiratory valve further obstructs the bias flow at intervals determined by the set expiratory time (T e). This produces a controlled increase in the pressure at the airway opening to the desired PIP for the set T i.
Intermittent positive pressure ventilation (IPPV) is termed time-cycled pressure-limited ventilation. This mode of ventilation is more commonly referred as IMV, but is also referred to as conventional and controlled mandatory ventilation (both abbreviated as CMV). Intermittent mandatory ventilation became the main stay of neonatal ventilation and remains one of the most common modes of ventilation worldwide.
21.1.2 Inspiratory Flow Settings
In most neonatal ventilators, there is a single flow control setting during IMV, which is applied during both the inspiratory and expiratory phases, while in other devices there is a separate setting for the inspiratory flow. In many new ventilators, an airway pressure profile setting is used in addition or in lieu of the inspiratory flow rate setting. Flow during the inspiratory phase determines the airway pressure profile. At higher flow rates, the airway pressure rises more rapidly and reaches the set PIP earlier. A rapidly rising airway pressure profile (approaching a square waveform) produces an initially high flow of gas moving through the patient’s airway towards the distal portions of the lung. The patient’s inspiratory flow profile, which indicates the speed at which the lung is inflated, is characterized by an initial peak that rapidly decays towards zero as the lung fills.
Higher inspiratory flow rates will also prolong the plateau of the airway pressure during which the lung is kept inflated before the set inspiratory phase ends. Although this increases mean airway pressure and may improve oxygenation by better alveolar expansion, high flow rates are usually avoided to reduce the risk of lung injury caused by the sudden expansion of air spaces. In contrast, a low inspiratory flow rate may be insufficient to reach the set PIP during a limited T i and deliver a smaller V T. This will occur in older generation ventilators that do not self adjust the inspiratory flow to reach the set PIP. Inspiratory flow rates in small preterm infants are usually maintained between 5 and 8 L/min, but these can be set at higher values for larger infants. In general, there is little data on the most adequate flow rate for different patient sizes or disease conditions.
The flow rate during the expiratory phase plays an important role in maintaining the PEEP level. An insufficient bias flow, set manually or automatically, can induce elastic loading during spontaneous breathing when the infant’s inspiratory flow is higher than the bias flow. This can be recognized by a decline in the PEEP level below the set value or even below zero during each inspiration. In newer ventilators, a flow demand valve adjusts the flow to maintain the PEEP level constant during the changing demands induced by spontaneous breathing. The expiratory flow rate is also used to remove the exhaled gases that would otherwise remain in the circuit near the airway opening and possibly inhaled again.
The work of breathing is minimized once the inspiratory flow rate reaches 65 Lpm in adults, and the patient is ventilated primarily in the control phase of the A/C modality (Ward et al. 1988). As the patient’s spontaneous efforts become more frequent or dead space is added to the circuit, then the patient’s work of breathing significantly increases and is difficult to attenuate with flow delivery in A/C ventilation (Marini et al. 1986). Historically, assisted breaths were facilitated with square wave flow patterns in the A/C form of ventilation. However, now they can be delivered by alternate designs such as sine wave and accelerating and decelerating waveforms (Mador 1994). Perhaps more importantly, rates of flow can be adjusted during inspiration on many of the newer generation ventilators; however, the resultant flows created are still dependent on respiratory system compliance (Jolliet and Tassaux 2006; Richard et al. 2002). Nevertheless, the slope of pressurization which could result in less time for patient demand and less work of breathing is vastly improved in regard to the decelerating flow setting in the newer generation ventilators when evaluated in lung models (Richard et al. 2002).
21.1.3 Cycle Time Settings
The transition from inspiration to expiration is known as cycling. Typically, this occurs when instantaneous inspiratory flow (V′insp) decreases to a predetermined fraction of peak inspiratory flow (V′ insp/V′ peak) which is referred to as an “expiratory trigger” (ET) (Jolliet and Tassaux 2006; Brochard 1994). Normally, cycling would coincide with the end of the patient’s inspiratory demand. However, delayed cycling occurs when pressurization is extended by the machine into the patient’s expiratory phase. This delayed cycling can lead to expiratory asynchrony and increased work of breathing (Jubran et al. 1995; Parthasarathy et al. 1998). On many ventilators, the cutoff value of ET is predetermined, usually at a default setting of 0.25; that is, the ventilator cycles when V′ insp has decreased to 25 % of V′ peak (Jolliet and Tassaux 2006). When airway resistance increases, the shape of the inspiratory flow curve changes. Hence, the 0.25 point will be reached later, which in turn increases the likelihood of delayed cycling (Jolliet and Tassaux 2006). Inspiratory time is managed to achieve the desired lung inflation. In A/C the ventilator cycles at the infant’s spontaneous breathing frequency, therefore, long T i is usually avoided to minimize the risk of insufficient time for exhalation that can lead to gas trapping or disruption of the breathing frequency. In some ventilators, the duration of the ventilator breath can be flow cycled and the set T i becomes the duration limit.
The increase in airway pressure during the mechanical inspiratory cycle produces lung inflation. T i should be sufficiently long to allow complete lung inflation with the set PIP. Duration of the actual lung inflation does not depend on T i except when T i is too short. Lung inflation continues as long as the gradient produced by the increase in airway pressure relative to the alveolar pressure remains positive. As the lung inflates against the lung’s recoil, the intrapulmonary pressure increases until it reaches equilibrium with the airway pressure and inflation ends. If T i is insufficient, the inspiratory cycle ends before equilibrium and the delivered V T is smaller. This is illustrated in Fig. 21.1.
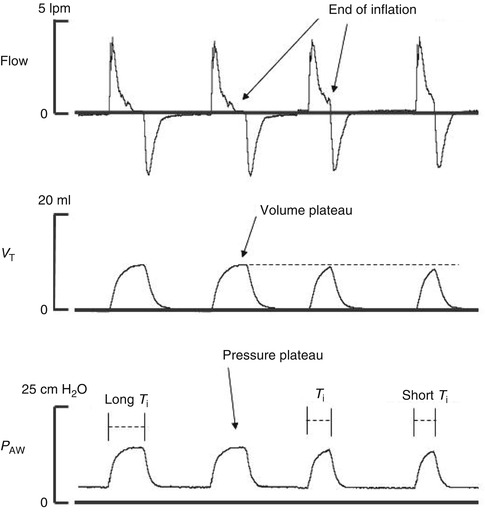
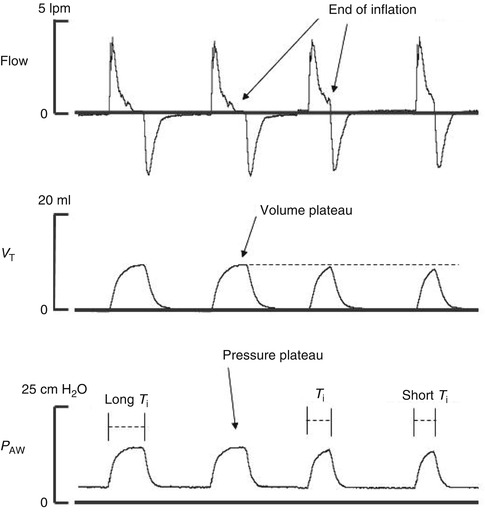
Fig. 21.1
Recordings of flow, tidal volume (V T), and airway pressure (P AW) show the effects of a long T i that prolongs the duration of the inspiratory phase and produces a volume plateau without achieving a gain in V T. On the other hand, a short T i does not allow for pressure equilibrium to be reached and full inflation is not achieved
The mechanical characteristics of the infant’s respiratory system, compliance and airway resistance determine, the time constant of the respiratory system (their product), which varies during different phases of respiratory disease in preterm infants. In restrictive conditions such as RDS, lung compliance is low (recoil is high with short time constant) and the pressure in the lung rises rapidly. Extending T i may not increase V T but only maintain a volume plateau. Thus, in cases of low lung compliance, an increase in PIP may be required to achieve the desired V T, whereas a longer T i may only prolong the inspiratory volume plateau. In obstructive conditions (high airway resistance, long time constant), the patient’s inspired flow is reduced, which delays inflation and the rise in intrapulmonary pressure. The gradient between airway and the alveolar pressure persists longer, and equilibrium is reached later. In these cases, an insufficient T i may not allow the desired V T. In preterm infants presenting with RDS requiring mechanical ventilation, T i is typically set between 0.25 and 0.4 s. Longer T i and shorter T e may result in gas trapping at very high ventilator frequencies as shown in Fig. 21.2.
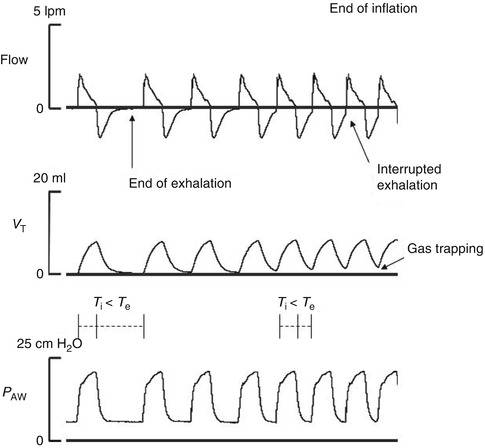
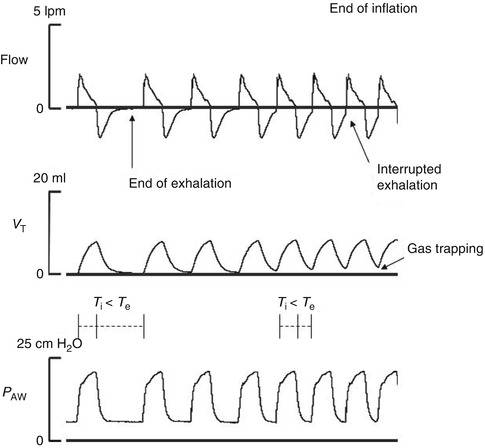
Fig. 21.2
Recordings of flow, tidal volume (V T), and airway pressure (P AW) show the gas trapping produced when T e is too short. Each new ventilator cycle interrupts and does not allow for a complete exhalation
The set T e and T i determine the ventilator frequency. Similarly to lung inflation, the actual duration of exhalation is not determined by T e except when T e is too short. When T e is too short, a new ventilator cycle interrupts exhalation resulting in gas trapping. This is more common with frequencies that result in T e shorter than 0.5 s and when the time constant is prolonged. The longer time constant in obstructive conditions require careful adjustment of the ventilator rate to maintain the minimum T e required for complete lung deflation.
21.1.4 Expected Physiologic Effects
Assist-control mechanical ventilation is designed to adequately eliminate carbon dioxide (CO2) and deliver oxygen in order to meet metabolic requirements regardless of the patient’s own breathing efforts (Mador 1994). Thus, the idea is to immediately avoid respiratory acidosis and to optimize aerobic metabolism. Respiratory acidosis is known to deepen sedation and cause “narcosis” and can clinically cause stupor and coma (Kirsch and Jozefowicz 2002). On the other hand, arterial blood gas analysis needs to be carefully followed to avoid hyperventilation which could result in hypocapnia and subsequent respiratory alkalosis which would lead to a “confusional” mental status (Cohen 2003). Moreover, sudden drops in CO2 could result in stupor and coma as well, secondary to decreased cerebral blood flow and consequent ischemia (Faden 1976). In regard to oxygenation, metabolic alkalosis is known to shift the oxyhemoglobin disassociation curve to the left which results in less oxygen release to cells; this is known as the Bohr effect (Levitzky 1986).
The cardiac effects of CMV and IMV have been described in adults. In adults who have undergone aortocoronary bypass but otherwise have normal heart function, CMV decreased cardiac index and stroke volume compared to IMV suggesting a negative impact on preload from CMV. However, CMV was superior in patients with poor left ventricle function due to the observation that both pulmonary artery pressures and right atrial pressures increased during IMV indicating that higher metabolic demands were imposed in the setting of poor reserve (Mathru et al. 1982). Nevertheless, the negative cardiac effects of IMV in patients with poor ventricular function was ameliorated with the application of 5 cmH2O PEEP (Mathru et al. 1982). This experience was corroborated by another group looking at the effects of PEEP at different ranges of pulmonary artery wedge pressures (PAWP) where there was a tendency for improvement in cardiac output with PEEP even at higher PAWPs (Grace and Greenbaum 1982). An approach called phasic high intrathoracic pressure support in adult patients in shock states (e.g., septic shock and cardiac infarction) where patients had their chest walls and abdomens binded resulted in improvements in cardiac output that was thought to be a result of decreasing left ventricular afterload (Pinsky and Summer 1983). For further details on cardiopulmonary interactions, see Part XVIII, Chap. 53.
21.1.5 Clinical Experience, Advantages, and Limitations
21.1.5.1 In the Neonatal Patient
Assist control can be used in both the acute and weaning phase of respiratory failure. In A/C, adjustment of PIP is primarily aimed at providing adequate V T. Following the initial phase of respiratory failure, PIP should be gradually weaned by targeting 3–5 mL/kg as lung mechanics improve and the spontaneous inspiratory effort contributes more to the generation of V T of the assisted breath. The backup ventilator rate is particularly important in preterm infants with inconsistent respiratory drive to avoid hypoventilation during apnea. However, a backup rate that is near or above the infant’s spontaneous rate can take over ventilation by inhibiting the spontaneous respiratory drive.
Intermittent mandatory ventilation is used during the acute phase of respiratory failure as well as during the weaning and chronic phases of respiratory disease. During IMV, the total V E is determined by the V T generated by the ventilator and the cycling rate plus the contribution of spontaneous breathing. As the ventilator PIP and rate are decreased, the infant’s inspiratory effort plays a larger role in the maintenance of ventilation. Inconsistency of the infant’s respiratory drive resulting in periods of absent spontaneous breathing, a respiratory effort insufficient to produce an adequate V T, or significant mechanical loads imposed on the respiratory pump by the underlying lung disease often prolong the course of IMV. In general, V T’s in the range of 3–5 mL/kg are considered appropriate in the neonate with lung disease. Ventilator rate is adjusted depending on spontaneous breathing and range of arterial CO2 tension (PaCO2) considered adequate. This is usually 40–50 mmHg, although higher PaCO2 levels are often tolerated to facilitate weaning in infants with chronic lung disease.
The management of the ventilator settings during IMV is relatively simple which is particularly appealing to many clinicians and may be the reason why IMV became the mainstay in many neonatal intensive care centers. In older IMV devices, volume monitoring was not available and PIP was adjusted based on sufficient chest expansion and blood gas status. Inadequate or excessive lung inflation, reduced ventilation that could lead to progressive atelectasis, and the occurrence of gas trapping could not be identified whereas in newer ventilators graphic V T monitoring can be used for PIP titration to maintain V T within the desired range.
Asynchrony between the ventilator and the infant’s spontaneous breathing is frequently observed during IMV. Ventilator cycles of fixed T i and T e can occur at different time points of the spontaneous breath. Spontaneous breathing frequency and duration of spontaneous inspiration and exhalation in preterm infants are very variable, which contributes to the asynchrony (Greenough et al. 1983a, b, 1984). When the mechanical breath is delivered midway or near the end of the spontaneous inspiration, it often extends into what should have been exhalation. By activating stretch inhibitory reflexes, the additional lung inflation or prolonged volume plateau produces an inspiratory hold and can limit the spontaneous respiratory rate. This could increase V T above that generated by the spontaneous inspiration resulting in greater risk for volutrauma. A mechanical breath delivered during the spontaneous expiratory phase can, by the same mechanism, prolong its duration and in some instances cause the infant to actively exhale against the positive pressure, which can lead to marked intrathoracic pressure swings. This ventilator asynchrony during IMV has been associated with air leaks and increased risk of intraventricular hemorrhage (IVH) (Perlman et al. 1983; Stark et al. 1979; Greenough and Morley 1984).
Assistance of every spontaneous inspiration in A/C produces a reduction in breathing effort compared to modes such as IMV or SIMV that assist only some spontaneous breaths (Hummler et al. 1996a; Kapasi et al. 2001). Therefore, many believe A/C prevents fatigue and produces a better V T to dead space (V D) ratio than the V T produced by spontaneous breaths in IMV or SIMV. In randomized clinical trials, A/C was shown to facilitate weaning and reduce the duration of mechanical ventilation compared to IMV, but these differences were not observed when compared to SIMV (Chan and Greenough 1993, 1994; Donn et al. 1994; Baumer 2000; Beresford et al. 2000; Dimitriou et al. 1995). In A/C, PIP should be titrated carefully because an excessive V T can lead to periods of hyperventilation with inhibition of spontaneous drive and apnea with the backup ventilator rate. On the other hand, if PIP is too low, ventilation may be insufficient during periods of apnea when the backup ventilator rate only delivers breaths of small V T. Autocycling due to leaks around the endotracheal tube and water condensation in the circuit are common. None of the currently available ventilators have an actual upper cycling rate limit in A/C; therefore, autocycling is more serious in A/C than with SIMV because of the potential risk for hyperventilation leading to hypocapnia or gas trapping and inhibition of the spontaneous respiratory drive. Mechanical cycles with a T i that exceed what is required for lung inflation or the duration of spontaneous inspiration are frequently observed in preterm infants (Dimitriou et al. 1998). Because these cycles are delivered at every spontaneous inspiration, they can disrupt spontaneous breathing pattern (Beck et al. 2004; Upton et al. 1990).
21.1.5.2 In the Pediatric Patient
The assist-control modality is used in pediatric patients who are either experiencing or recovering from deep sedation or anesthesia. Furthermore, the mode is used when supporting children with lung disease that requires moderate ventilator settings. Moderate ventilator settings defined as parameters used to support patients who generate PIP of 22–26 cmH2O while delivering 6–8 mL/kg of V T (maximum of 350 mL). Ideally, a patient considered a candidate for extubation should generate a PIP less than 22 cmH2O while creating a V T less than 8 mL/kg (maximum of 350 mL), a PEEP of 5 cmH2O and an oxygen source of less than 40 % with an intact respiratory drive, ability to protect his airway, control his secretions, and exhibit easy work of breathing (e.g., no diaphoresis, tachycardia, or persistence of condition that resulted in intubation). The advantage of A/C ventilation is that the practitioner can continue to support patients with relatively poorly compliant lungs while allowing them to still breathe spontaneously. Potentially, analgesic and sedative requirements can be decreased if the ventilator’s inspiratory demand mechanism operates with a high fidelity. The limitations of the A/C mode is that while the intention is to allow patients to breathe above the ventilator settings if desired, there may still be considerable pressures delivered by the ventilator that can be uncomfortable for the patient. Thus, some patients will not tolerate decreases in their analgesics or sedatives. Positive pressure ventilation in general may be detrimental in patients who are hemodynamically unstable with decreased intravascular volume and have tenuous pulmonary hypertension and increased intracranial hypertension.
21.2 Synchronized Intermittent Mandatory Ventilation
The idea of IMV was first described in the mid-1950s by Bjork and Engstrom (1955). This form of ventilation was successfully implemented in pediatric practice by Kirby and colleagues in the management of infants with respiratory distress (Kirby et al. 1971, 1972; Kirby 1977). Synchronized intermittent mandatory ventilation is a mode of ventilator support in which a preset number of breaths (mandatory) are delivered over time (usually over a minute) and are targeted to a specific parameter [e.g., V T (volume control) or pressure control] in a given breath cycle (typically 1 min). During SIMV, the mandatory breaths are either patient initiated or ventilator initiated. Furthermore, patients can initiate additional breaths if they desire above and beyond the set number prescribed. The principle difference in SIMV compared to other ventilator modalities where patients can initiate breaths is that in SIMV, the patient can breathe spontaneously and control the depth of his breaths. Classically, this mode was designed to “synchronize” a patient’s breathing effort with a ventilator’s response of support, but this is now a feature of other modes of ventilation as well (American Association for Respiratory Care 1992).
In SIMV, synchronous triggering is achieved by opening consecutive time windows where a mechanical breath is triggered by the first spontaneous inspiration in every new window. In the absence of spontaneous breathing, a mandatory mechanical breath is delivered at the end of the window. In this way, the interval between mechanical breaths is not constant as in IMV, but the total number of mechanical breaths delivered per minute is preset and constant. In SIMV, synchronous delivery of the mechanical cycle with the spontaneous inspiratory effort produces a greater transpulmonary pressure which results in an often larger and more consistent V T compared to IMV cycles delivered at different points of the spontaneous breath as in IMV.
Various methods of synchronization have been used in neonatal ventilation. These include the Graseby pressure capsule that detects outward motion of the abdomen during inspiration, esophageal pressure balloon to detect negative deflection during inspiration, thoracic respiratory impedance, changes in airway pressure produced by spontaneous inspiration, and flow sensors that detect gas flow produced during spontaneous inspiration. Most new generation ventilators utilize flow sensors for breath triggering in preterm infants. This is due to the greater sensitivity of these devices compared to other methods (John et al. 1994; Nikischin et al. 1996; Hummler et al. 1996b; Dimitriou et al. 2001). Flow sensors also allow monitoring of V T and V E produced by synchronized mechanical breaths and unassisted spontaneous breaths. Nonetheless, flow sensors have limitations related to autocycling due to leaks around uncuffed endotracheal tubes used in the neonate, or water condensation artifacts, and increased dead space (Figueras et al. 1997; Claure et al. 2003).
21.2.1 Pressure and Flow Triggering
The SIMV mode is designed to deliver a set amount of breaths as dialed into the ventilator. In addition, the patient can trigger breaths as sensed by the ventilator’s demand mechanism. Inspiratory efforts are typically sensed by either a pressure-triggered (PT) or flow-triggered (FT) mechanism. The PT mechanism detects a fall in pressure, whereas the FT strategy senses a change in flow before delivering a breath (Sassoon 1994). Consequently, the operator must set a threshold sensitivity in order for either a flow or PT breath to be delivered.
In regard to patient comfort, the work of breathing has been shown to be improved utilizing flow triggering in adults receiving either continuous positive airway pressure (CPAP) or SIMV (Branson et al. 1994; Sassoon et al. 1994). This a characteristic of the efficiency of patient-ventilator interaction as well as the flow delivery attributes (e.g., bias flow which provides some pressure support prior to breath sensing compared to the pressure trigger mechanism) (Sassoon et al. 1994). In contrast, no difference in the work of breathing was appreciated by other investigators evaluating FT and PT in adults receiving pressure support (Goulet et al. 1997). In the SIMV mode in children, no significant decrease in the work of breathing was seen in spontaneously breathing subjects using the FT by Thiagarajan et al., though these patients were deemed ready to extubate at the time of the assessment (Thiagarajan et al. 2004). Thus, the effects of PT and FT may not be discernable until managing children with lung disease.
The management of SIMV is in many aspects similar to that of IMV during the different phases of respiratory disease. Flow settings during SIMV, as in IMV, should be sufficient to maintain PEEP and flush exhaled gases during the mechanical expiratory phase and enable generation of the desired PIP within the allotted T i.
Cycle time settings during SIMV are for the most part similar to those for IMV. However, because every mechanical cycle is synchronized with the spontaneous inspiration, insufficient trigger sensitivity (delayed triggered) or a too long T i can prolong the cycle into the spontaneous exhalation phase in a consistent basis, whereas in IMV, these occur only in some of the mechanical cycles. Since V T is more consistent and larger with SIMV, a slightly shorter T i is often sufficient to achieve lung inflation.
Many ventilators provide the ability to cycle off the mechanical breath when inspiratory flow declines towards zero near the end of inflation or the spontaneous inspiration. This technique is coined flow cycling and consists of terminating the ventilator cycle when flow declines below a preset fraction of the peak flow measured during inspiration. A separate control is available in some ventilators by which the operator sets the fraction of the peak inspiratory flow at which inspiration ends. With this, the actual T i setting becomes a maximum duration limit and the effective T i is automatically adjusted during each breath.
21.2.2 Cycle Time Settings
Conceptually, the breathing cycle is partitioned into two phases; phase one is characterized by the delivery of the preset mandatory breath. Again in this phase, the patient can trigger the breath, but if he fails to, then the ventilator will deliver the breath before this phase ends. In the second phase, only the patient can trigger a breath and oftentimes there is some support provided (e.g., pressure support) to lessen the effort required. Thus, the actual cycle time setting is determined based on the number of breaths that the practitioner wishes to have over a 1-min period. The number of breaths is used to divide 60 s (1 min) in order to define the “mandatory breath period.” For instance, if one wants a patient to be guaranteed at least 20 bpm, then this would result in a cycle time of 60 s divided by 15 or 4 s per cycle time. In other words, if a patient takes a breath at the beginning of the 4-s period, then he may take more breaths during the remaining time if he desires until the next 4-s window occurs. Nevertheless, if the patient does not initiate a breath within that 4-s period, then the ventilator will deliver a breath.
21.2.3 Expected Physiologic Effects
By far, the most anticipated physiologic effect of SIMV is the preservation of spontaneous breathing while minimizing the imposed work of breathing from the ventilator circuitry and demand valve mechanism by means of a responsive ventilator with a trigger feature that functions at high fidelity (Sanders et al. 2001). In addition, the ability to breathe spontaneously in this mode would enable the patient to augment their respiratory delivery to meet their metabolic demands if desired and perhaps direct airflow to areas that have better ventilator: perfusion ratios. Nevertheless, it is important to understand that despite the intent to allow the patient to breathe as much as tolerated, the subject is still receiving positive pressure ventilation. Thus, certain congenital heart lesions that may benefit physiologically in regard to cardiac preload, afterload, or pulmonary blood flow from a negative pressure respiratory situation would not necessarily do better in the SIMV mode compared to other modes of positive pressure ventilation from a physiologic perspective.
21.2.4 Clinical Experience, Advantages, and Limitations
21.2.4.1 In the Neonatal Patient
Synchronized intermittent mandatory ventilation was one of the synchronized modes of neonatal ventilation that was more rapidly adopted in neonatal centers because it maintained the general management features of IMV. Synchronized intermittent mandatory ventilation is commonly used during the acute phase of respiratory failure throughout the weaning as well as chronic phases of respiratory failure in preterm infants. During SIMV, the infant’s inspiratory effort contributes to the generation of V T of the synchronized mechanical breath. Peak inspiratory pressure is gradually reduced as lung mechanics improve and the infant’s contribution to maintain V T increases. Tidal volume in the range of 3–5 mL/kg is generally considered adequate for the neonate with lung disease. In SIMV, total V E is determined by the V T of the assisted breaths and the ventilation produced by the non-assisted spontaneous breaths occurring between SIMV cycles. As the ventilator PIP and rate are decreased, the infant’s inspiratory effort plays a larger role in the maintenance of V E.
In the acute phase of respiratory failure, SIMV rate ranges between 40 and 60 breaths per min (bpm) which is likely to assist almost every spontaneous inspiration. The ventilator rate is gradually weaned as the spontaneous breathing becomes more consistent to achieve acceptable levels of PaCO2 depending on the condition of the infant. In most cases, acceptable PaCO2 levels range between 40 and 50 mmHg, but in infants with chronic lung disease higher PaCO2 are often tolerated.
In preterm infants, SIMV produces a more consistent and larger V T compared to IMV which leads to improved gas exchange and a more efficient ventilation requiring a lower spontaneous breathing frequency (Bernstein et al. 1996; Smith et al. 1997; Cleary et al. 1995). In clinical trials, SIMV has been shown to accelerate weaning, shorten mechanical ventilation, and reduce the incidence of severe bronchopulmonary dysplasia in infants weighing <1,000 g at birth (Bernstein et al. 1996; Chen et al. 1997). A recent report indicates less spontaneous fluctuations in oxygenation during SIMV vs. IMV (Firme et al. 2005).
Inadequate function or setting of the synchronization mechanism can lead to delay or lack of triggering and autocycling during SIMV. Delayed triggering with respect to the onset of the spontaneous inspiration, usually caused by insufficient sensitivity of the trigger setting, can prolong the inspiratory phase and disrupt the breathing pattern (Beck et al. 2004). Lack of triggering during SIMV, which usually occurs when the spontaneous inspiration is not sufficient to reach the trigger threshold level, results in the ventilator cycling at the set mandatory rate as if the infant was supported in IMV. On the other hand, a too sensitive trigger setting or a continuous gas leakage can produce autocycling at the set ventilator rate, which also results in a ventilator pattern that resembles IMV.
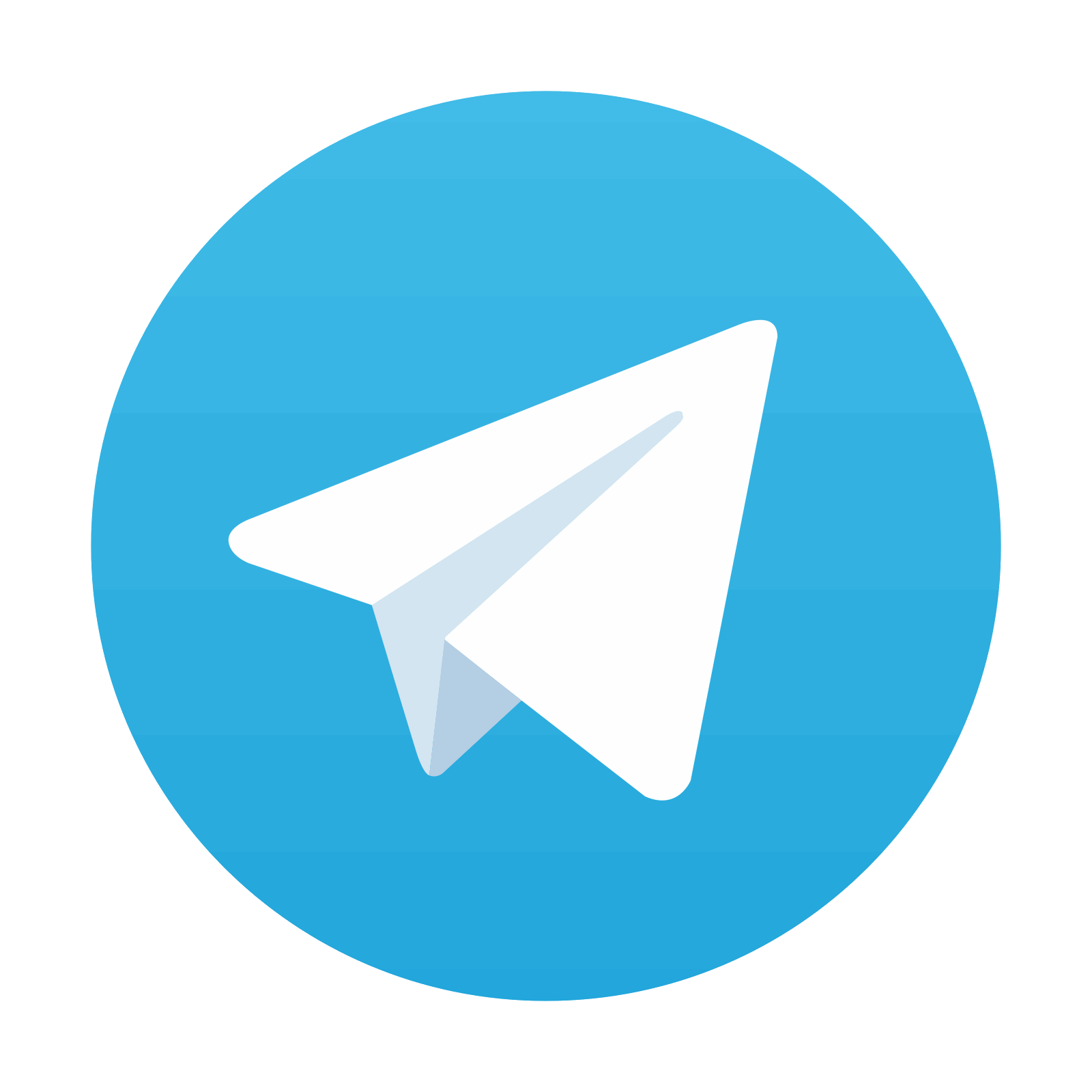
Stay updated, free articles. Join our Telegram channel

Full access? Get Clinical Tree
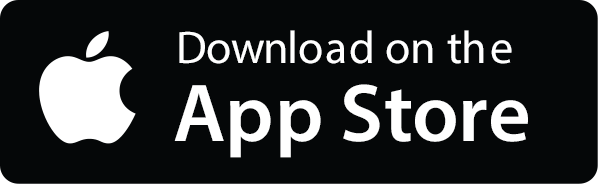
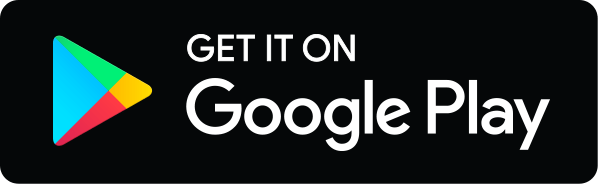