Key Points
- •
IgE-producing B cells arise from IgM + or IgG + B cells via the process of class switch recombination (CSR). B cells undergoing CSR undergo somatic gene rearrangements in the immunoglobulin heavy chain locus leading to the assembly of a gene encoding the ε-heavy chain retaining the original antigenic specificity of the B cell clone.
- •
IgE antibody production is regulated by Th2 cells. These provide a combination of signals including secreted cytokine (IL-4 or IL-13) and cell surface molecules (CD40L).
- •
IgE signaling via FcεRI, its high-affinity receptor, on mast cells and basophils by polyvalent antigen leads to the activation of a complex array of signaling pathways resulting in the release of preformed and newly synthesized mediators of immediate hypersensitivity.
- •
The low-affinity IgE receptor, CD23, mediates IgE-facilitated antigen uptake by antigen-presenting cells, transcellular allergen transport in gastrointestinal and airway epithelium and regulation of IgE production.
- •
IgE antibodies regulate numerous aspects of hypersensitivity including IgE-receptor density on mast cells and basophils and mast cell homeostasis.
Normally present at very low levels in plasma, antibodies of the immunoglobulin E (IgE) isotype were first discovered in 1967, decades after the description of IgG, IgA and IgM. IgE antibodies are produced primarily by plasma cells in mucosal-associated lymphoid tissue and their levels are uniformly elevated in patients suffering from atopic conditions like asthma, allergic rhinitis and atopic dermatitis. Production of allergen-specific IgE in atopic individuals is driven both by a genetic predisposition to the synthesis of this isotype as well as by environmental factors, including chronic allergen exposure. The lineage commitment by B cells to produce IgE involves irreversible genetic changes at the immunoglobulin heavy chain gene locus and is very tightly regulated. It requires both cytokine signals (interleukin [IL]-4 and IL-13) and interaction of TNF receptor family members on the B cell surface with their ligands.
IgE antibodies exert their biologic functions via the high-affinity IgE receptor, FcεRI, and the low-affinity receptor, CD23. In the classic immediate hypersensitivity reaction, the interaction of polyvalent allergens with IgE bound to mast cells via FcεRI triggers receptor aggregation, which initiates a series of signals that result in the release of vasoactive and chemotactic mediators of acute tissue inflammation. Clinical manifestations of IgE-induced immediate hypersensitivity include systemic anaphylaxis (triggered by foods, drugs and insect stings), bronchial edema with smooth muscle constriction and acute airflow obstruction in asthmatic patients (following allergen inhalation), angioedema and urticaria. Although best known for their critical function in mediating antigen-specific immediate hypersensitivity reactions, IgE antibodies also exert potent immunoregulatory effects including regulation of mast cell homeostasis, stabilization of IgE receptor expression and enhancement of mast cell-mediated expansion of Th2 responses and suppression of T reg responses to allergens.
Components of the Immune Response
Immunoglobulin E Protein Structure and Gene Organization
Immunoglobulin E (IgE) antibodies are tetramers consisting of two light chains (κ or λ) and two ε-heavy chains ( Figure 4-1 and Box 4-1 ). The heavy chains each contain a variable (V H ) region and four constant region domains. The V H domain, together with the V-regions of the light chains (V L ), confers antibody specificity and the Cε domains confer isotype-specific functions, including interaction with FcεRI and CD23. IgE antibodies are heavily glycosylated and contain numerous intrachain and interchain disulfide bonds. The exons encoding the ε-heavy chain domains are located in the Cε locus near the 3′ end of the immunoglobulin heavy chain locus (IgH) ( Figure 4-2 ). Additional exons, M1 and M2, encode hydrophobic sequences present in the ε-heavy chain mRNA splice isoforms encoding transmembrane IgE in IgE + B cells. In contrast to IgG antibodies, which have a half-life of about 3 weeks, IgE antibodies are very short-lived in plasma ( 1ess than 1 day), but they can remain fixed to mast cells in tissues for weeks or months.

IgE Antibodies, Genes and Receptors
|
IgE protein |
IgE gene arrangement | |
|
Germline transcription |
Structure of the Iε promoter | |
Cytokine regulation of germline transcription | |
CD40/CD154 signaling | |
TACI/BAFF signaling | |
Activation-induced cytidine deaminase | |
DNA double strand breaks and repair | |
|
FcεRI |
CD23 |

The assembly of a functional IgE gene requires two sequential processes of DNA excision and ligation. In the first, which occurs in pre-B cells, individual V H , D, and J H exons randomly combine to generate a V H DJ H cassette encoding an antigen- specific V H domain. In B cells that have undergone ‘productive’ V H DJ H rearrangements (e.g. no stop codons have been introduced during assembly), this V H DJ H cassette is situated just upstream of the Cµ and Cδ exons so that functional µ- and δ-heavy chain transcripts can be produced.
A second DNA excision and ligation process, called class switch recombination (CSR), must occur before B cells can produce antibodies of other isotypes, including IgE. These antibodies retain their original V H DJ H cassette and antigenic specificity but exchange C H cassettes of various isotypes to construct different heavy chains that exert distinct biologic functions. In this tightly regulated and irreversible process, sometimes referred to as deletional switch recombination, a long stretch of genomic DNA spanning from the Sµ region between V H DJ H and Cµ to Sε upstream of the Cε locus is excised (see Figure 4-2 ). The DNA products of this reaction include an extrachromosomal circle of intervening DNA and the contiguous V H DJ H and Cε sequences, joined by Sµ-Sε ligation, to generate a functional IgE gene. A complex series of cytokine signals and cell surface interactions collaborate to trigger deletional switch recombination in B cells destined for IgE production.
Regulation of IgE Isotype Switching
ε-Germline Transcription Precedes Isotype Switch Recombination
Before deletional isotype switch recombination is initiated, cytokine signals provided by IL-4 and/or IL-13 induce RNA transcription in the IgH locus of B cells. This occurs at the unrearranged or ‘germline’ ε-heavy chain locus driven from a promoter 5′ of the Iε exon, located just upstream of the Sε switch recombination region and the four Cε exons ( Figure 4-3 ). This is referred to as ε-germline RNA and the transcripts include a 140-bp Iε exon as well as exons Cε1-Cε4. As Iε contains several stop codons, germline transcripts do not encode functional proteins and have been referred to as ‘sterile’.

Regulation of Germline Transcription, The Iε Promoter
Initiation of germline transcription is regulated by the Iε promoter that contains binding sites for several known transcription factors including STAT-6, NF-κB, BSAP (Pax5), C/EBP and PU.1 (see Figure 4-3 ). Accessibility of the promoter is regulated by the non-histone chromosomal protein, HMG-I(Y). This repression is released upon IL-4-driven phosphorylation of the protein. Translocation of activated STAT-6 to the nucleus is triggered by IL-4 and IL-13 signaling. STAT-6 activation appears to be the key inducible regulator of ε-germline transcription; neither BSAP nor NF-κB nuclear-binding activities are altered by cytokine signaling, but these promoter elements must be present for normal Iε promoter function. CD40 signaling also enhances cytokine-driven germline transcription by activating the NF-κB promoter elements.
BCL-6, a POZ/zinc-finger transcription factor expressed in B cells, is an important negative regulator of the Iε promoter. BCL-6 binds to STAT-6 sites and can repress the induction of ε-germline transcripts by IL-4. BCL-6 is induced by the cytokine, IL-21, which is known to suppress IgE production in B cells and which has been reported to induce apoptosis of IgE + B cells. IL-21 is important in germinal center formation and germinal centers have relatively low levels of IgE production.
Cytokines IL-4 and IL-13 Activate STAT-6
The cytokines IL-4 and IL-13 are potent inducers of ε-germline transcription in B cells. The multimeric receptors for these two cytokines share the IL-4R-αchain. The type I IL-4 receptor, which binds IL-4, is composed of the ligand-binding IL-4Rα and the signal-transducing common cytokine receptor γ-chain γc. The type II receptor, which can bind either IL-4 or IL-13, contains the IL-4R-α chain along with an IL-13 binding chain, IL-13Rα1. IL-4 receptor signaling triggers the activation of Janus family tyrosine kinases Jak-1 (via IL-4Rα), Jak-3 (via γc) and TYK2 (via IL-13Rα). These activated Jaks then phosphorylate tyrosine residues in the intracellular domains of the receptor chain. These phosphotyrosines serve as binding sites for STAT-6, which is, in turn, phosphorylated and then dimerizes and translocates to the nucleus.
CD40/CD154 Provides Second Signal for Isotype Switch Recombination
The cytokines IL-4 and IL-13 are very efficient inducers of ε-germline transcription, and this transcription is an absolute prerequisite for isotype switching. However, cytokine-induced germline transcription alone is not sufficient to drive B cells to complete the genomic deletional switch recombination reaction that gives rise to a functional IgE gene. A second signal, provided by the interaction of the TNF receptor family member CD40 on B cells with its ligand, CD154, on activated T cells, is required to bring the process to completion.
CD154 is transiently expressed on antigen/MCH-stimulated T cells. T cell CD154 induces CD40 aggregation on B cells, triggering signal transduction via four intracellular proteins belonging to the TRAF family of TNF-receptor associated factors. TRAF-2, -5, and -6 promote the dissociation of NF-κB from its inhibitor, IκB, allowing NF−κB to translocate to the nucleus and synergize with STAT-6 to activate the Iε promoter as described above. In addition to inducing TRAF association and signaling, aggregation of CD40 activates protein tyrosine kinases (PTKs) including Jak-3, which play an important role in immunoglobulin class switching. CD154 is encoded on the X chromosome. Boys with X-linked immunodeficiency with hyper-IgM (XHIM) are deficient in CD154. Consequently, their B cells are unable to produce IgG, IgA or IgE.
Alternative Second Signals for Isotype Switch Recombination
Recently, alternative switching pathways have been defined in which the second ‘switch’ signal is provided not by CD40/CD154 ligation but rather by interaction of other TNF-like molecules with their receptors. One such TNF family member, BAFF, binds to its receptor TACI on cytokine-stimulated B cells, inducing isotype switching even in the absence of CD40. BAFF/TACI-driven switching may be of particular importance at mucosal sites, especially IgA production in the gastrointestinal tract. Defects in this pathway underlie some cases of IgA deficiency. Although BAFF can drive IgE switching, its physiologic relevance in IgE regulation remains to be clarified. It has been reported that respiratory epithelium produces BAFF, with elevations of the factor in bronchoalveolar lavage fluid (BAL) of segmental allergen-challenged subjects. In addition, it has been demonstrated that IgE class switch recombination occurs not only in central lymphoid organs but also in the respiratory mucosa of patients with allergic rhinitis and asthma.
Cytokine-Stimulated Germline Transcripts and CD40-Induced AID Collaborate to Execute Switch Recombination
Deletional class switch recombination stimulated by cytokines and CD40/CD154 requires the synthesis of a new intracellular protein, activation-induced cytidine deaminase (AID), which is expressed in activated splenic B cells and in the germinal centers of lymph nodes. AID-deficient mice have elevated IgM levels and a major defect in isotype switching with absent IgG, IgE and IgA. A rare autosomal form of hyper-IgM syndrome (HIGM2), which is associated with striking lymphoid hypertrophy, has now been attributed to mutations in the AID gene.
AID is recruited to sites of active germline transcription where it deaminates deoxy-cytidine residues within the C-rich Sε and Sµ sequences, generating uracils and consequent U : G mismatches (see Figure 4-4 ). Subsequent removal of these uracils by the enzyme uracil glycocylase (UNG) results in the introduction of abasic sites. The enzyme apurinic/apyrimidinic endonuclease 1, APE1, generates nicks at these sites which ultimately lead to double-stranded DNA breaks. In subsequent steps of the process, analogous breaks, located at Sµ between V H DJ H and the Cµ exons, are annealed to generate a functional IgE gene. The heterogeneous nature of the Sµ-Sε junctions suggests a nonhomologous end-joining mechanism such as would be generated by the DNA repair enzymes, Ku70, Ku80 and DNA-PKcs. Consistent with this possibility, B cells lacking Ku70, Ku80 and DNA-PKcs, all of which are involved in nonhomologous end joining, cannot execute isotype switching normally.

Regulation of Allergen-Specific T Cell Responses
The execution of IgE isotype switch recombination in B cells, as detailed previously, requires that cytokine (IL-4 and IL-13) signals and the CD40 ligand, CD154 signal, be delivered in a coordinated fashion. Both these stimuli are provided by Th2-type allergen-specific T-helper cells. Thus, the mechanisms that regulate expansion and survival of Th2 cells are crucial in regulating IgE responses.
Th2 Helper T Cell Development
Naïve CD4 + Th cells have the capacity to differentiate into a number of distinct types of effector helper, each with distinct capacities for induction of cellular immune responses (Th1), antibody production and allergic responses (Th2), inflammatory responses (Th17) and regulation (T reg , see Figure 4-5 ). These Th types are further characterized by the expression of specific transcription factors that maintain their specific lineage commitments and direct their respective cytokine transcription profiles ( Chapter 5 ). Some of the Th lineages can be identified by specific cell surface markers. Th1 cells, which arise under the direction of IL-12 or IL-18, express abundant IFN-γ and IL-2 and are important in immunity to intracellular pathogens. Th1 cells are further characterized by the presence of the transcription factor, T-bet. The Th17 subset is induced in the presence of TGF-β and IL-6 and produces IL-17, TNF-α and IL-1. Th17 cells harbor the transcription factors RORγt and STAT3 and are important in driving neutrophil recruitment and inflammatory responses. As their name implies, T reg , which are generated in the presence of TGF-β and IL-2 (absent IL-6), are important in controlling immune responses via immunosuppressive cytokines including TGF-β and IL-10. The transcription factor associated with this lineage is FoxP3.

The critical Th cells promoting IgE production are Th2, which are induced by IL-4, express the transcription factor GATA-3 and produce IL-4, IL-5, IL-6, IL-9, IL-10, IL-13 and GM-CSF. Th2 cells express cell surface receptors, which target their trafficking to allergic sites and trigger activation in settings of allergic inflammation, including the chemokine receptors CCR3, CCR4, CRTh2 and CCR8 and the IL-33 receptor, T1/ST2.
Genetic Influences on Th2 Development
Both host and environmental factors promote the Th2 shift observed in allergic individuals. Genetic predispositions toward Th1 or Th2 are partly accounted for by T cell autonomous tendencies to transcribe Th1 versus Th2 cytokines, but are also the result of a wide range of influences external to T cells. Perhaps the most potent Th1/Th2-polarizing effect is exerted by the cytokine milieu, particularly tissue levels of IL-4, IL-12 and IFN-γ. IL-4 promotes Th2 responses and suppresses Th1 development. IL-12 drives Th1 differentiation (an effect that is greatly potentiated by the presence of IFN-γ) and can inhibit and even reverse Th2 development. In ongoing immune responses these cytokines can be provided by existing T cells already committed to a particular Th phenotype. In de novo allergen encounters, cytokines produced by cells of the ‘innate’ immune response may tip the balance.
Antigen-Presenting Cell Function in Th Differentiation
Naïve T cells initially encounter antigens as MHC-bound processed peptides on the surface of antigen-presenting cells (APCs). The most potent APCs are dendritic cells (DCs), which reside in tissues as immature sentinels and sample antigens in their milieu. Upon activation, these cells acquire mature APC function and migrate to regional lymphoid tissues, where they efficiently activate antigen-specific T helper cells via MHC-peptide complexes. Dendritic cells obtained from various lymphoid tissues in vivo or cultured ex vivo under a range of conditions all express MHC II and, following activation, express costimulatory molecules, including CD80/86. However, there is some functional heterogeneity among DCs, especially with respect to the ability to induce Th1 versus Th2 T helper responses. DC-derived IL-12 drives Th1 responses; IL-23, TGF-β and IL-6 support Th17 induction; and IL-10 drives both T reg and Th2.
Microbial Products and Dendritic Cell Phenotype
The recent understanding that Th polarity may be determined by DC polarity obviously begs the following question: what determines DC polarity? IFN-γ favors DC1 development, whereas histamine and PGE 2 promote the development of DC2. IL-10 may negatively regulate DC production of IL-12. Conserved microbial structures, which signal via the Toll-like receptor (TLR) family of receptors, can shift DC polarity. Dendritic cells express a range of TLR and the specific effects of ligand binding by each of these receptors on DC phenotype remain to be fully elucidated. The default state of mucosal DC appears to be skewed toward Th2 induction with relatively low basal IL-12 and constitutive production of IL-10.
Non-T Cell Sources of IL-4: Mast Cells, Basophils, NKT Cells and NK Cells
Although allergen-specific T-helper cells committed to the Th2 lineage are a major source of IL-4 in allergic tissues and may predominate during chronic or memory responses to allergen, several other cell types can provide IL-4 and IL-13 and may be more important in initial allergen encounters. Mast cells, which are abundant in the respiratory and gastrointestinal mucosa, are excellent producers of both IL-4 and IL-13 following activation via IgE/FcεRI. IgE-stimulated mast cells appear to be a key early source of IL-4 in Th2-dominant immune responses to food allergens. Basophils are rapidly induced in response to allergens or parasites and constitutively produce large quantities of IL-4. NK1.1 + CD4 + T (NKT) cells are another source of IL-4. These cells express a very restricted repertoire of αβ T cell receptors and interact with the non-classical MHC class I molecule, CD1. The intravenous injection of anti-CD3 in mice induces large amounts of IL-4, derived primarily from these NKT cells. Another recently identified cytokine-producing cell of the innate immune system, the innate lymphoid cell type-2 (ILC2), is commonly found at mucosal sites where its expansion is stimulated by the epithelial cell cytokines, IL-25 and IL-33. The ILC2 lineage is stabilized by the transcription factor RORα, and secretes IL-4, IL-13 and IL-5.
Sites of IgE Class Switch Recombination and Mechanisms of IgE Memory
Studies of IgG production in mice have revealed that high-affinity antibody responses arise in germinal centers of secondary lymphoid tissues in which IgM + B cells are driven by cytokine signals and costimulatory molecules from T follicular helper cells (T FH ) to switch to IgG (µ-γ switch), followed by affinity maturation and generation of long-lived memory B cells. IgE responses may also be induced in germinal centers via direct IgM-IgE switching (µ-ε), but several lines of evidence suggest that affinity maturation is optimized in B cells that have sequentially undergone µ-γ, and then γ-ε switches and that memory resides in the intermediate IgG + B cell compartment. IgE + B cells are short-lived in germinal centers, possibly because of rapid transition to plasma cells. Early, low-affinity IgE responses may arise at extrafollicular sites including in the respiratory and gastrointestinal mucosa where the presence of ε germline transcripts and switch excision circles ( Figure 4-4 ) is readily detected, especially following allergen exposure. Mice unable to generate germinal centers (including BCL-6 −/− and MHC II-deficient mice) are capable of producing abundant IgE. The current understanding of IgE synthesis gleaned from these observations is that early low-affinity IgE responses arise in mucosal sites, but that affinity maturation and memory are optimized in germinal centers.
IgE Receptors
FcεRI Structure
The high-affinity IgE receptor FcεRI is a multimeric complex expressed in two isoforms, a tetrameric αβγ2 receptor present on mast cells and basophils and a trimeric αγ2 receptor expressed, albeit at levels 10-fold to 100-fold lower, by several cell lineages including eosinophils, platelets, monocytes, dendritic cells and cutaneous Langerhans cells ( Figure 4-6 ). The α chain contains two extracellular immunoglobulin-related domains and is responsible for binding IgE. The β subunit of the receptor contains four transmembrane-spanning domains with both N- and C-terminal ends on the cytosolic side of the plasma membrane. FcεRI-β appears to have two functions that result in enhanced receptor activity. β-chain expression both enhances cell surface density of FcεRI and amplifies the signal transduced following activation of the receptor by IgE aggregation. The γ chains (which have homology to the ζ and η chains important in T cell receptor signaling) exist as disulfide-linked dimers with trans-membrane domains and cytoplasmic tails. The β and γ chains perform critical signal transduction functions and their intracellular domains contain immunoreceptor tyrosine-based activation motifs (ITAMs), 18 amino acid long tyrosine-containing sequences that constitute docking sites for SH2 domain-containing signaling proteins.

CD23 Expression and Structure
Although its common designation as the ‘low-affinity’ IgE receptor implies differently, CD23 actually has a fairly high affinity for IgE with a K A of about 10 8 . A wide variety of cell types express CD23 in humans, including B cells, Langerhans cells, follicular dendritic cells, T cells and eosinophils. It is a type II transmembrane protein with a C-type lectin domain, making it the only immunoglobulin receptor that is not in the Ig superfamily. Adjacent to its lectin domain, CD23 has sequences that are predicted to give rise to α-helical coiled-coil stalks ( Figure 4-7 ). As a result, CD23 is known to have a tendency to multimerize and only oligomeric CD23 will bind IgE. CD23 has homology to the asialoglycoprotein receptor, suggesting a role for CD23 in endocytosis. In addition to binding IgE, CD23 binds to a second ligand, the B cell surface molecule, CD21.

Full access? Get Clinical Tree
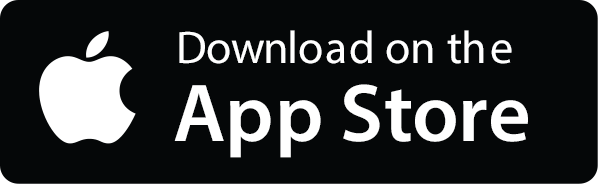
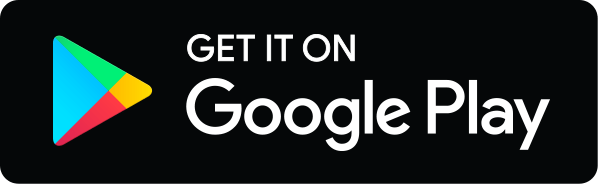