Rapid Sequence Induction
Noel Zuckerbraun
Raymond D. Pitetti
Introduction
A detailed discussion of the various methods for performing emergent endotracheal intubation can be found in Chapter 16. This chapter will review the techniques of pharmacologic facilitation of intubation. The standard procedure of providing sedation and inducing neuromuscular paralysis in preparation for intubation is called “rapid sequence induction” (RSI). The entire process—i.e., RSI and endotracheal intubation—is often called “rapid sequence intubation.” The purpose of RSI is to allow a safe, expedient intubation while reducing the likelihood of aspiration pneumonitis. RSI minimizes or prevents many of the patient’s responses to the noxious stimuli of intubation, including vomiting, coughing, breath-holding, and laryngospasm. This procedure and its modifications can also limit adverse physiologic effects of intubation, such as increased intracranial pressure (ICP), systemic hypertension and hypotension, cardiac arrhythmias, and elevated intraocular pressure. In addition, RSI facilitates laryngoscopic visualization of the airway in situations when this might otherwise be impossible, as with patients who are seizing, unable to cooperate, or combative.
Certain clinical situations require using one of several modifications of the standard RSI procedure. For example, muscle relaxants should not be administered if bag-valve-mask (BVM) ventilation and/or intubation are anticipated to be difficult for patients who are spontaneously breathing. In such cases, paralysis followed by an unsuccessful intubation produces apnea without a patent airway and therefore may be lethal. In this situation, sedation alone may be indicated to facilitate intubation without eliminating respiratory function. The various approaches that can be used in managing the potentially difficult airway are described in Chapter 17. Other modifications of RSI involve the use of alternative agents for sedation and neuromuscular blockade or the addition of adjunctive agents to prevent increases in ICP (e.g., with cerebral edema or a space-occupying intracranial lesion) or circulatory collapse for the patient with impending or existing shock due to hypovolemia, a low cardiac output state, etc. Agents with vasodilating or myocardial depressant effects that can exacerbate pre-existing physiologic derangements must obviously be titrated carefully or avoided. Medications can sometimes be substituted or added that may increase systemic blood pressure or decrease ICP, depending on the clinical situation. In its classic form, RSI involves preoxygenating the patient by providing 100% mask O2 during spontaneous respiration. BVM ventilation is not performed following administration of the induction agents to reduce the likelihood of vomiting before intubation by minimizing the amount of air forced into the stomach (see also Chapter 14). However, patients with pre-existing respiratory compromise and hypoxemia may not tolerate even a brief period of apnea despite preoxygenation. These patients therefore require skilled BVM ventilation with cricoid pressure for 45 to 90 seconds with 100% O2 until the peak effect of the muscle relaxant has been achieved and endotracheal intubation can be attempted. These and other modifications of RSI are described in this chapter.
Depending on the availability and experience of medical personnel, RSI for critically ill or injured children is generally performed by either an emergency physician or anesthesiologist. Because the potential for morbidity is high with this procedure, the physician performing RSI must be skillful with pediatric endotracheal intubation and thoroughly familiar with the risks and benefits of the medications and techniques used. Optimal settings for this procedure are the emergency department (ED), intensive care unit (ICU), and operating room. Above all, practitioners must keep in mind that RSI suppresses all respiratory effort and eliminates the ability of the patient to protect and maintain the airway. Consequently, this procedure should be performed when clinically
indicated with appropriate caution and meticulous attention to proper technique.
indicated with appropriate caution and meticulous attention to proper technique.
Anatomy and Physiology
Important features of pediatric airway anatomy and the physiologic effects of endotracheal intubation are described in Chapter 16. However, additional factors specifically related to the use of pharmacologic agents for facilitating intubation merit discussion. For one, the child with a suspected “difficult airway” represents a particular concern for the operator performing RSI, because the suppression of spontaneous respirations before obtaining a secure airway is especially dangerous with such patients. Identifying such patients whenever possible before initiating RSI is therefore vital. In addition, the physiology of the normal protective airway reflexes and the “full stomach” state have important consequences with regard to preventing aspiration pneumonitis, one of the primary purposes of this procedure. Finally, the operator should fully understand the pharmacologic properties and physiologic effects of the agents used in performing RSI.
Chapter 17 provides a detailed discussion of the methods used in providing respiratory support for the child with a difficult airway. In most instances, such patients can be identified before intubation is attempted. Unfortunately, this is not always possible, even when an intubation is performed on an elective basis. In the limited time available before an urgent or emergent intubation, the operator must make a rapid assessment of the patient and, to the extent possible, ascertain that BVM ventilation and endotracheal intubation will not be unduly difficult. If time permits, obtaining historical information from the parent or caretaker may be helpful in making this judgment. A known congenital abnormality, previous problems with intubation, or a history of difficulty breathing (e.g., while asleep or feeding or with upper respiratory infections) can all indicate a potentially problematic airway. A wide diversity of congenital anomalies has been associated with difficult intubation. Examples include Klippel-Feil syndrome, Down syndrome, achondroplasia, the Pierre-Robin sequence, Treacher-Collins syndrome, Marfan syndrome, Cornelia de Lange syndrome, Moebius syndrome, and familial osseous dysplasia (cherubism). The approach to such patients can only be fully tailored to the specific challenges posed by each syndrome when time is not a concern, as with an elective intubation. A complete discussion of these issues is therefore beyond the scope of this chapter. Readers interested in more information on this subject are referred to standard pediatric and anesthesiology texts. More importantly in the emergent setting, physical examination will usually reveal anatomic characteristics that would pose significant obstacles to successful laryngoscopy and/or manual ventilation, such as micrognathia (best evaluated while viewing the patient’s profile), macroglossia, cleft or high arched palate, protruding upper incisors, small mouth, limited temporomandibular joint mobility, or limited cervical spine mobility (Table 15.1). Difficult laryngoscopy should also be anticipated when the patient has any of the following acquired abnormalities: hoarseness, stridor, drooling, a preferred posture, facial burns, blunt or penetrating injury to the neck, facial bone fractures, oral trauma, epiglottitis, retropharyngeal abscess, and a foreign body in the extrathoracic airway (Table 15.2). For the patient with a potentially unstable cervical spine, laryngoscopy and intubation may be significantly hindered by in-line stabilization and use of a cervical collar, which limit neck flexion/extension and mouth opening. Indeed, this is perhaps the most common clinical scenario for a failed intubation.
TABLE 15.1 Congenital Anatomic Abnormalities indicating a Potentially Difficult Airway | |
---|---|
|
It is important to remember, however, that paralysis (and, to a lesser extent, sedation) is a concern in the setting of a probable difficult airway only if the patient has some degree of adequate respiratory function prior to RSI. In other words, if there is no meaningful respiratory function to “lose” by administering a sedative or paralytic agent, then the decision to perform RSI when needed is not difficult. For example, with a spontaneously breathing child who is maintaining an acceptable oxygen saturation but who nevertheless requires urgent endotracheal intubation (e.g., a combative but otherwise stable trauma patient who needs a head CT scan), the clinician should be circumspect about eliminating respiratory function if the likelihood of a difficult airway is high. Transforming a reasonably stable situation into a “cannot bag/cannot intubate” scenario without a compelling reason for taking such a risk (e.g., rapid and potentially fatal patient deterioration) should obviously be avoided. In such cases, it is advisable to move the patient to a more stable environment (ICU, operating
room) and, when available, obtain the assistance of an anesthesiologist and/or an otolaryngologist prior to performing RSI.
room) and, when available, obtain the assistance of an anesthesiologist and/or an otolaryngologist prior to performing RSI.
TABLE 15.2 Acquired Abnormalities Indicating a Potentially Difficult Airway | |
---|---|
|
By contrast, a child requiring immediate endotracheal intubation who has no significant respiratory function and for whom BVM ventilation alone is inadequate (e.g., an apneic seizure patient with clenched teeth, vomiting, and worsening hypoxia), the presence of a potentially difficult airway must not inhibit the operator from performing the necessary life-saving interventions of an appropriate RSI—despite the attendant danger of a failed intubation. As described in Chapter 17, equipment for alternative methods of securing a definitive airway should be made readily available in such cases should standard endotracheal intubation and BVM ventilation prove impossible, but the RSI must be performed. In such situations, the potential hazards of RSI are outweighed by the likelihood of a patient suffering a rapid respiratory death.
Upper airway reflexes that normally serve to protect against aspiration of foreign material into the trachea and lungs can be a significant impediment to performing endotracheal intubation. Instrumentation of the airway may stimulate gagging, coughing, jaw clenching, increased production of secretions, and laryngospasm. These reflexes must often be suppressed for an intubation to be atraumatic and successful. The physiology of the various mechanisms that mediate these reflexes is complex. To briefly summarize, irritation of upper airway and digestive tract mucosa stimulates the glossopharyngeal (9th) and vagus (10th) nerves, which project to the nucleus of the solitary tract (NTS) in the medulla (1). Gagging and coughing are produced by nerve impulses relayed from the NTS back to the motor efferents of the upper airway, diaphragm, and intercostal and abdominal muscles. In addition, nerve tracts from the NTS to the medulla activate a “vomiting center,” which induces emesis by coordinating such responses as contraction of the abdominal musculature, closure of the glottis, opening of the lower esophageal sphincter, and increased salivation. This medullary vomiting response can also be stimulated by the area postrema of the brainstem, which is sensitive to noxious substances circulating in the bloodstream (1). Sympathetic outflow resulting from stimulation of the NTS can cause an increase in heart rate and blood pressure, which in turn can lead to dysrhythmias and increased ICP. However, the most pronounced response to oropharyngeal manipulation in children is generally transmission from the NTS to the dorsal vagal nucleus, producing marked bradycardia. Because cardiac output in infants and younger children depends more on heart rate than changes in stroke volume, bradycardia can have a significant deleterious effect on systemic blood pressure.
Any patient undergoing emergent intubation is presumed to have a “full stomach.” A full stomach may result from recent ingestion of food (within the past 8 hours) or liquid (within the past 2 hours) or delayed gastric emptying due to intestinal obstruction, trauma, pain, pregnancy, elevated ICP, or shock. Presumption of a full-stomach state means the patient is at risk for emesis and subsequent aspiration of gastric contents into the trachea and lungs. Factors increasing this risk include agitation, persistent gagging or coughing, and elevated intragastric pressure as a result of gas entry into the stomach during BVM ventilation or swallowing of air and/or blood. The normal response of gagging and possibly vomiting provoked by stimulation of the pharynx can be suppressed with pharmacologic agents, yet these same agents also reduce or eliminate closure of the epiglottis over the glottic opening that prevents aspiration should any vomiting or regurgitation of stomach contents occur. Ensuring the proper sequence of events during RSI is therefore vital when managing patients presumed to have a full stomach.
The ideal pharmacologic properties of medications used in RSI are rapid onset and short duration of effect. Anatomic and physiologic characteristics of pediatric patients can have important clinical effects on these properties. Onset and duration depend on the total body compartments of fat, muscle, and water. Total body water is 75% to 80% of body composition in a newborn, decreasing to the adult proportion of 60% at about 6 months of age. This is why infants may require a larger initial weight-based dose of water-soluble drugs than adults. In addition, agents that depend on redistribution to fat and muscle for termination of effect will have a longer duration of action in younger patients, because they have lower body fat and muscle content. With older children, the half-life of a medication will generally be shorter, because a larger proportion of the cardiac output is delivered to the liver and kidneys, causing more rapid metabolism of the drug. As a general rule, drugs have a more rapid onset of effect and a shorter duration of action in children than in adults, and a larger weight-based dose will be required to achieve the same anesthetic effect.
The primary desired effects of pharmacologic facilitation of intubation are sedation, analgesia, and muscle relaxation. Sedation is generally provided by barbiturates, benzodiazepines, and/or opioids. Barbiturates have several actions in the central nervous system (CNS) that produce sedation. Most importantly, they potentiate the neuroinhibitory actions of gamma-aminobutyric acid (GABA), which is accomplished by increasing chloride conductance and decreasing depolarization induced by glutamate. Evidence also exists of depression of the calcium-dependent action potential (2). Etomidate is a carboxylated imidazole compound that induces anesthesia by modulating GABA-mediated neurotransmission. Though not itself a barbiturate, the mode of action of etomidate is similar to that of the barbiturates. Benzodiazepines potentiate GABA-mediated inhibition as well, but little is known about their direct mechanistic effects. Neurologic inhibition by opiates is relatively complex. There are three known major categories of opioid receptors in the CNS. Analgesia is produced by endogenous and exogenous peptides that bind to the μ receptors in the brain, κ receptors in the spinal cord, and δ receptors distributed in both the brain and spinal cord. Inhibition at these sites is accomplished by a reduction in the release of neurotransmitters and inhibition of adenyl cyclase
at μ and δ receptors. Evidence also exists of inhibition of calcium channels at κ receptors (2). Activation of opioid receptors causes a decrease in the sensation of pain as well as modification of the perception of a stimulus as painful. For example, the periaqueductal grey matter in the midbrain contains δ and μ receptors, and agonists at these sites inhibit the processing of nociceptive information from the spinal cord. In addition, an abundance of μ receptors are found in the locus ceruleus, which is responsible for feelings of alarm, panic, fear, and anxiety (2). Inhibition at these receptors increases the tolerance for pain and the associated psychological stress.
at μ and δ receptors. Evidence also exists of inhibition of calcium channels at κ receptors (2). Activation of opioid receptors causes a decrease in the sensation of pain as well as modification of the perception of a stimulus as painful. For example, the periaqueductal grey matter in the midbrain contains δ and μ receptors, and agonists at these sites inhibit the processing of nociceptive information from the spinal cord. In addition, an abundance of μ receptors are found in the locus ceruleus, which is responsible for feelings of alarm, panic, fear, and anxiety (2). Inhibition at these receptors increases the tolerance for pain and the associated psychological stress.
Muscle relaxation is produced at the motor end plate by depolarizing agents or nondepolarizing competitive agents. Normally, release of acetylcholine (ACh) from the presynaptic membrane causes summation of electrical potentials in order to form an action potential, thus stimulating muscle contraction. Depolarizing agents mimic ACh and bind directly to the postsynaptic ACh receptor, causing all skeletal muscles to depolarize. This is often evident (although more commonly in adults than children) as diffuse, random contraction of skeletal muscle fibers known as “fasciculations,” followed by flaccid paralysis that remains until postsynaptic receptors again regain the ability to transmit an electrical stimulus (2). Nondepolarizing neuromuscular blocking agents competitively inhibit the nicotinic postsynaptic receptors for ACh so that depolarization cannot occur. These agents are normally metabolized over time to less active forms, which are then more easily displaced by ACh. Both types of neuromuscular blockers have no direct CNS effects and do not decrease awareness. Using these agents alone without sedation therefore leaves a patient in the terrorized state of being fully awake but unable to move or breathe.
Indications
Rapid sequence induction is performed to facilitate endotracheal intubation. Consequently, the indications for RSI in the acute care setting are in part determined by the indications for emergent intubation. Yet not all patients who require intubation on an emergent basis are appropriate candidates for RSI. An algorithm illustrating the assessments and decisions involved in determining the subset of patients who should undergo RSI is presented in Figure 15.1. In general, RSI is indicated for patients with airway or ventilatory compromise who cannot be intubated without using sedatives and neuromuscular blocking agents or who would be at increased risk for complications without these drugs and techniques. Indications for one of the modifications of RSI depend on the specific clinical circumstances (see below). As mentioned previously, even in the best of situations RSI is a challenging and potentially dangerous procedure, and it should therefore be performed only when the operator presumes with reasonable certainty that the trachea can be intubated with reasonable rapidity. If intubation is delayed or impossible, subsequent positive pressure ventilation (PPV) with a BVM circuit may increase intragastric pressure, causing decreased total lung compliance and a greater likelihood of aspiration. In the worst-case scenario, BVM ventilation may be ineffective and therefore adequate respiratory support impossible to provide. For these reasons, RSI should be performed only after the airway has been evaluated as thoroughly as possible under the circumstances and a judgment made that endotracheal intubation, or at minimum BVM ventilation with cricoid pressure, can be successfully accomplished on a timely basis.
The first question asked in determining whether a standard RSI is indicated should be, Does the child require immediate intubation? As described in Chapter 16, there are seven primary indications for emergent intubation: (i) maintenance of the airway, (ii) protection of the airway (e.g., before gastric lavage for the obtunded patient), (iii) administration of PPV, (iv) oxygen administration, (v) delivery of resuscitation drugs through the endotracheal tube, (vi) access to the trachea for suctioning, and (vii) respiratory support when other systems are failing in the unstable patient. Numerous disease processes and injuries can cause sufficient respiratory compromise in pediatric patients to require emergent endotracheal intubation. However, in certain instances intubation will be necessary—but not immediately. Some patients with impending respiratory failure will be stable enough to transfer to a more controlled setting such as the operating room or ICU if this substantially increases the safety of an endotracheal intubation. In such cases, temporary respiratory support can be provided as necessary by assisted or controlled ventilation using a BVM circuit.
If intubation is necessary and cannot be delayed, the second question that must be asked is, Can the patient be safely intubated without sedation or neuromuscular blockade? Many intubations in the ED are performed on patients who are moribund, and therefore drugs are not needed to prevent movement or induce unconsciousness. Furthermore, many physicians prefer to make one attempt at an “awake” intubation (i.e., no
muscle relaxant but possibly some degree of sedation) whenever this can be performed without additional risk to the patient. However, many situations exist in which either an awake intubation is hazardous and/or the likelihood of success with this method is clearly low from the outset. For example, when the patient is struggling forcefully and restraint is necessary or the patient’s jaw is firmly clenched, an awake intubation is likely to be impossible. In such cases, attempting intubation without neuromuscular blockade may cause trauma and bleeding, which make subsequent attempts more difficult while increasing the likelihood of aspiration. Consequently, for the patient who requires immediate intubation but for whom this cannot be accomplished safely without sedation and muscle relaxation, a standard RSI is indicated.
muscle relaxant but possibly some degree of sedation) whenever this can be performed without additional risk to the patient. However, many situations exist in which either an awake intubation is hazardous and/or the likelihood of success with this method is clearly low from the outset. For example, when the patient is struggling forcefully and restraint is necessary or the patient’s jaw is firmly clenched, an awake intubation is likely to be impossible. In such cases, attempting intubation without neuromuscular blockade may cause trauma and bleeding, which make subsequent attempts more difficult while increasing the likelihood of aspiration. Consequently, for the patient who requires immediate intubation but for whom this cannot be accomplished safely without sedation and muscle relaxation, a standard RSI is indicated.
As discussed previously, coughing, gagging, and vomiting as a result of airway manipulation are all known to cause an increase in ICP. For this reason, a modification of the RSI procedure often called a “neurologic induction” (or “neurologic intubation”) should be considered with any patient, including infants, who may have elevated ICP. A neurologic induction simply refers to special measures taken to avoid further increases in ICP, which can produce cerebral hypoperfusion, cerebral swelling, and, in extreme cases, herniation. These patients present with such conditions as severe head trauma, ventriculoperitoneal (VP) shunt dysfunction, and large CNS tumors. For a neurologic induction, thiopental is usually used as the sedating agent because it is reliable in protecting the brain by preventing a rise in ICP during noxious stimulation. In addition, intravenous lidocaine can be administered because it also may attenuate the rise in ICP. Neuromuscular blockade should be provided to suppress the airway and autonomic reflexes, although the agent of choice in this situation is somewhat controversial (see “Procedure”). Prolonged apnea after administration of the muscle relaxant must be carefully avoided, as cerebral hypercarbia may exacerbate an elevated ICP, and these patients are often exquisitely sensitive to the deleterious effects of cerebral hypoxia. It should be noted that thiopental has a potent myocardial depressant effect and therefore must be administered in small, frequent doses—or avoided altogether—in patients suspected of having hypovolemia or a low cardiac output state. For the patient who is presumed to have a significantly elevated ICP and is also in a shock state, a different sedative (e.g., etomidate or midazolam) should be used.
Other indications that require special consideration relate to specific clinical presentations. For example, the patient with a possible open globe injury should not receive succinylcholine or ketamine, because both of these agents increase intraocular pressure and may cause extrusion of the vitreous. In addition, as with thiopental, other medications may induce profound hypotension in patients with cardiovascular compromise and should be avoided in such situations. For the patient with severe asthma who requires intubation, ketamine may be substituted as the sedation agent because it both supports the systemic circulation and may act as a bronchodilator. A more detailed discussion of the use of RSI with patients who require these types of specific adaptations is provided later in this chapter.
Many physicians prefer to intubate neonates without paralysis and often without sedation. This is usually more appropriate in the delivery room, where the degree of urgency and lack of intravenous access may preclude administration of intravenous medications. Although paralysis may seem unnecessary because an infant is more easily and effectively restrained, a darting tongue can sometimes prove a formidable barrier to rapid and successful laryngoscopy, and RSI should therefore be considered for patients of all ages when indicated in the ED. At the least, a sedating agent should be considered even in young infants.
Although relatively few, there are some important contraindications to RSI. For example, any child who is apneic and pulseless at presentation should undergo endotracheal intubation early in the resuscitation without undue delay. Pharmacologic agents are unnecessary in this situation. Similarly, a patient who is moribund as a result of an overwhelming disease process (e.g., sepsis) may gain no benefit from undergoing RSI. In such situations, performing RSI would add needless complexity to the management of the patient, potentially prolonging the period of inadequate oxygenation and ventilation without enhancing the safety of intubation. As mentioned previously, standard RSI is relatively contraindicated when the patient has one or more conditions associated with a potentially difficult airway and is breathing spontaneously or requires only 100% oxygen, continuous positive airway pressure, or minimal assistance with BVM ventilation. Should endotracheal intubation become necessary, an awake procedure (with judicious sedation as needed) should be attempted. In such cases, muscle relaxants should be withheld at least until effective BVM ventilation can be ensured. This is done after careful titration of sedative agents permits first assisted, and then controlled, BVM ventilation without eliminating protective airway reflexes. Additionally, nondepolarizing muscle relaxants should generally be avoided when the patient has a neuromuscular disease (e.g., Werdnig-Hoffman, myasthenia gravis, or Duchenne muscular dystrophy), because paralysis can persist for an excessively long period of time. In such cases, benzodiazepines may be used exclusively or in conjunction with an opiate. Succinylcholine, a depolarizing muscle relaxant, is absolutely contraindicated in patients with pre-existing hyperkalemia, susceptibility to malignant hyperthermia, and denervating neuromuscular diseases because of the risk of inducing an often fatal cardiac arrest. Finally, RSI is contraindicated for any spontaneously breathing patient suspected of having a tension pneumothorax, pericardial tamponade, or a mediastinal mass (e.g., lymphoma) compressing the heart, great vessels, or large airways. With such patents, sedative agents may directly or indirectly cause vasodilation and myocardial depression that could precipitate circulatory collapse. In patients with anterior mediastinal masses, neuromuscular blocking agents will also remove intrinsic muscle tone, which helps to maintain intrathoracic airway patency. Collapse of the airway resulting from compression by the mass
can make endotracheal intubation ineffective for providing respiratory support because obstruction occurs below the tip of the endotracheal tube. With large masses, this catastrophic situation can be precipitated by even judicious use of sedative agents. Consequently, such children should be intubated with utmost caution and only when necessary, optimally with the patient awake and sitting in a partially upright position and given a topical anesthetic as the only medication.
can make endotracheal intubation ineffective for providing respiratory support because obstruction occurs below the tip of the endotracheal tube. With large masses, this catastrophic situation can be precipitated by even judicious use of sedative agents. Consequently, such children should be intubated with utmost caution and only when necessary, optimally with the patient awake and sitting in a partially upright position and given a topical anesthetic as the only medication.
Equipment
The equipment necessary for performing RSI is listed in Table 15.3. Patients should have at least one reliable peripheral or central access site verified as intravenous. The appropriate pharmacologic agents should be drawn in labeled syringes. The general classifications of medications used to perform RSI are described below, with particular attention given to the most commonly used agent in each category.
Sedative Agents
Barbiturates (Thiopental)
Barbiturates are potent sedatives. From this class of drugs, thiopental is used most frequently in performing RSI. It is a rapidly acting agent, producing its peak effect in 10 to 30 seconds (2,3). The duration of effect depends on the rate of redistribution to fat and muscle but is generally between 5 and 30 minutes (3,4). Thiopental decreases the metabolic demands of the brain, thereby decreasing cerebral blood flow (3). It is therefore an excellent agent to use when the patient has a known or presumed elevated ICP (5). Thiopental also has anticonvulsant effects (6). Another barbiturate used in RSI is methohexital. Methohexital has similar characteristics to thiopental (3), although one important difference is that methohexital can produce seizure activity (3,6,7).
TABLE 15.3 Equipment for Rapid Sequence Induction | |
---|---|
|
Precautions
Thiopental is both a myocardial depressant and a peripheral vasodilator. In patients with pre-existing myocardial depression or hypovolemia, it can cause hypoperfusion associated with hypotension. Normally, baroreceptors detect a low blood pressure and compensate by producing a reflex tachycardia. However, hypovolemic patients with tachycardia may be incapable of mounting any further increase in heart rate and are therefore likely to become hypotensive. Patients with underlying cardiovascular disease may not produce adequate coronary and/or cerebral perfusion under these conditions (5,8). Consequently, any patient suspected of hypovolemia or cardiovascular disease should not receive thiopental or, if no appropriate substitute is available, should only receive it in small doses (1 to 3 mg/kg) at a decreased rate as tolerated based on blood pressure (5,6).
The physician choosing thiopental should also be aware that it has no analgesic effects (3). Patients intubated with thiopental as the sole sedative agent may have laryngospasm or cough in response to airway manipulation (9). The lack of analgesia when using thiopental alone may also allow a catecholamine response leading to systemic or intracranial hypertension in response to intubation or other noxious interventions. For these reasons, it is often beneficial for thiopental to be administered in conjunction with an analgesic, particularly when the patient is at risk for elevated ICP. In addition, extravasation or intra-arterial injection of thiopental can cause severe tissue necrosis (7). Extra care must therefore be taken to inject into an access site that recently has been verified as intravenous. It is also important to use only a dilute solution (2.5%) for injection (7).
Other precautions relate to the respiratory effects of this agent. It causes dose- and rate-dependent respiratory depression (8). Patients may have precipitous onset of apnea, particularly if there is associated hypovolemia or head trauma (7). Thiopental has minimal direct effects on bronchomotor tone, but bronchospasm may occur due to light anesthesia during noxious airway manipulation and may also result from histamine release (6). This effect is of particular concern in asthmatic patients.
How Supplied
Thiopental comes as powder for reconstitution as sodium in 250 mg, 400 mg, 500 mg, and 1 g. It is supplied with diluent for reconstitution immediately prior to use. Methohexital comes as powder for reconstitution as sodium in 500 mg, 2.5 g, and 5 g.
Dose
For normotensive patients with a normal or elevated cardiac output, the recommended dose of thiopental is 3 to 6 mg/kg
given rapidly as an intravenous bolus. If the patient is hypotensive but thiopental is nonetheless the selected agent (e.g., the patient has a markedly elevated ICP), a lower dose of 1 to 3 mg/kg may be used if blood pressure remains acceptable (6). For methoxital, the recommended intravenous induction dose is 1 to 2 mg/kg.
given rapidly as an intravenous bolus. If the patient is hypotensive but thiopental is nonetheless the selected agent (e.g., the patient has a markedly elevated ICP), a lower dose of 1 to 3 mg/kg may be used if blood pressure remains acceptable (6). For methoxital, the recommended intravenous induction dose is 1 to 2 mg/kg.
Benzodiazepines (Midazolam, Diazepam)
The benzodiazepines produce sedation, amnesia, and hypnosis. They also have anxiolytic and anticonvulsant effects. Midazolam and diazepam are the two most commonly used benzodiazepines for RSI. However, midazolam is the preferred agent for RSI in this class due to its rapid and consistent rate of onset, shorter duration, and lack of pain with injection (6). Midazolam has a rapid onset of approximately 30 to 60 seconds and a short duration of 5 to 20 minutes, while diazepam has a variable onset of action (1 to 3 minutes) and a longer duration (10 to 30 minutes) (3,5,10). Diazepam is oil based and can cause pain and occasionally phlebitis with intravenous administration (7). Midazolam is water soluble and does not cause pain with injection (7). Both benzodiazepines have a cerebral protective effect and do not increase ICP (3).
Precautions
Although the benzodiazepines produce fewer cardiovascular side effects than the barbiturates, they are associated with a slight transient decrease in systemic blood pressure because of a decrease in systemic vascular resistance (3). Evidence suggests that an increase in venous capacitance caused by midazolam is offset by a shift of blood volume out of the splanchnic circulation and a transient increase in heart rate (11). For some patients, a small decrease in blood pressure can be beneficial in attenuating both the pressor response and the rise in ICP that can occur with laryngoscopy (12). However, the patient with increased sympathetic activity compensating for significant hypovolemia is most susceptible to the hypotensive effects of midazolam (13). For these reasons, benzodiazepines should be used with caution in cases of severe cardiovascular compromise such as septic shock or multiple trauma. However, for most patients with cardiovascular disease, cardiac output and coronary blood flow are not adversely affected by midazolam.
Similar to barbiturates, benzodiazepines have a dose- and rate-dependent respiratory depressant effect that may induce apnea (more common with diazepam) (14). This effect is potentiated by the concomitant use of narcotics (3,6). Benzodiazepines do not provide analgesia, nor will they enhance the analgesic effects of other drugs. Consequently, when used for induction, benzodiazepines are normally paired with an agent that has analgesic properties such as fentanyl or ketamine (see below).
How Supplied
Midazolam (Versed) is an injectable solution supplied in concentrations of 1 and 5 mg/mL and is stored at room temperature.
Dose
The recommended dosage range for midazolam in pediatric patients is 0.1 to 0.3 mg/kg. The lower end of the range is often chosen for use in conjunction with potent analgesics or when the patient has cardiovascular compromise, and the higher end is recommended when midazolam is used alone and/or the patient is hemodynamically stable. Attention to appropriate dosing is important, as underdosing may produce suboptimal intubation conditions (15).
Narcotics (Fentanyl and Morphine)
Narcotics such as fentanyl and morphine produce analgesia and some sedation. Fentanyl is more highly lipid soluble than morphine, and thus it crosses the blood-brain barrier more rapidly and has a more rapid redistribution time. As a result, fentanyl has a faster onset of action (about 1 to 3 minutes to peak effect) and a shorter duration (30 to 60 minutes) than morphine (onset of 5 to 6 minutes and duration of 3 to 4 hours) (5,10). The faster onset of fentanyl makes it preferable to morphine as an induction agent for RSI. At higher doses, the duration of effect of fentanyl depends not on redistribution but on hepatic clearance as the drug accumulates in the body. A more prolonged effect of fentanyl can therefore be obtained at higher doses. A second peak can also occur as a result of release from pulmonary or skeletal muscle stores (16). Fentanyl has been shown to inhibit muscle fasciculations caused by succinylcholine (17) and to blunt the hemodynamic effects of laryngoscopy (18,19). Since larger doses of fentanyl are necessary to achieve significant sedative effects, it is often combined with benzodiazepines when used for intubation.
Precautions
All opiate narcotics produce respiratory depression. This effect, which is more common in neonates and infants, is dose dependent and can be reversed with naloxone. A rare but significant additional adverse respiratory effect of opioids is chest wall rigidity, a complication that, though more widely recognized with fentanyl, can occur with all opioids (10). Chest wall rigidity can interfere with spontaneous, assisted, or controlled ventilation (5,20). The incidence of this phenomenon is thought to be dependent on the dose and rate of administration. Chest wall rigidity can be prevented by the concomitant use of muscle relaxants or by administering the medication slowly (16,20). It may also be reversible with naloxone (21).
Morphine produces varying degrees of histamine release that can cause hypotension (although this is less common among pediatric patients than adults), and this effect is not inhibited or reversed by naloxone. One advantage of fentanyl is that it does not cause histamine release (16). Another reason fentanyl is often preferred over morphine is its minimal cardiovascular effects; however, it can cause a transient reduction in heart rate (8,16). For this reason, a vagolytic such as atropine should be used in conjunction with fentanyl when bradycardia would be undesirable. As mentioned previously, narcotics are often combined with benzodiazepines when used
as induction agents for RSI. However, this combination can lead to a significant drop in systemic vascular resistance and should therefore be avoided in patients with cardiovascular compromise (14).
as induction agents for RSI. However, this combination can lead to a significant drop in systemic vascular resistance and should therefore be avoided in patients with cardiovascular compromise (14).
Case reports of generalized tonic-clonic seizures after fentanyl administration have appeared in the literature (22,23,24). However, those undergoing simultaneous EEG during such episodes did not have identifiable seizure activity; thus, it has been suggested that the seizurelike episodes may have instead been myoclonus or severe muscular rigidity (24,25,26).
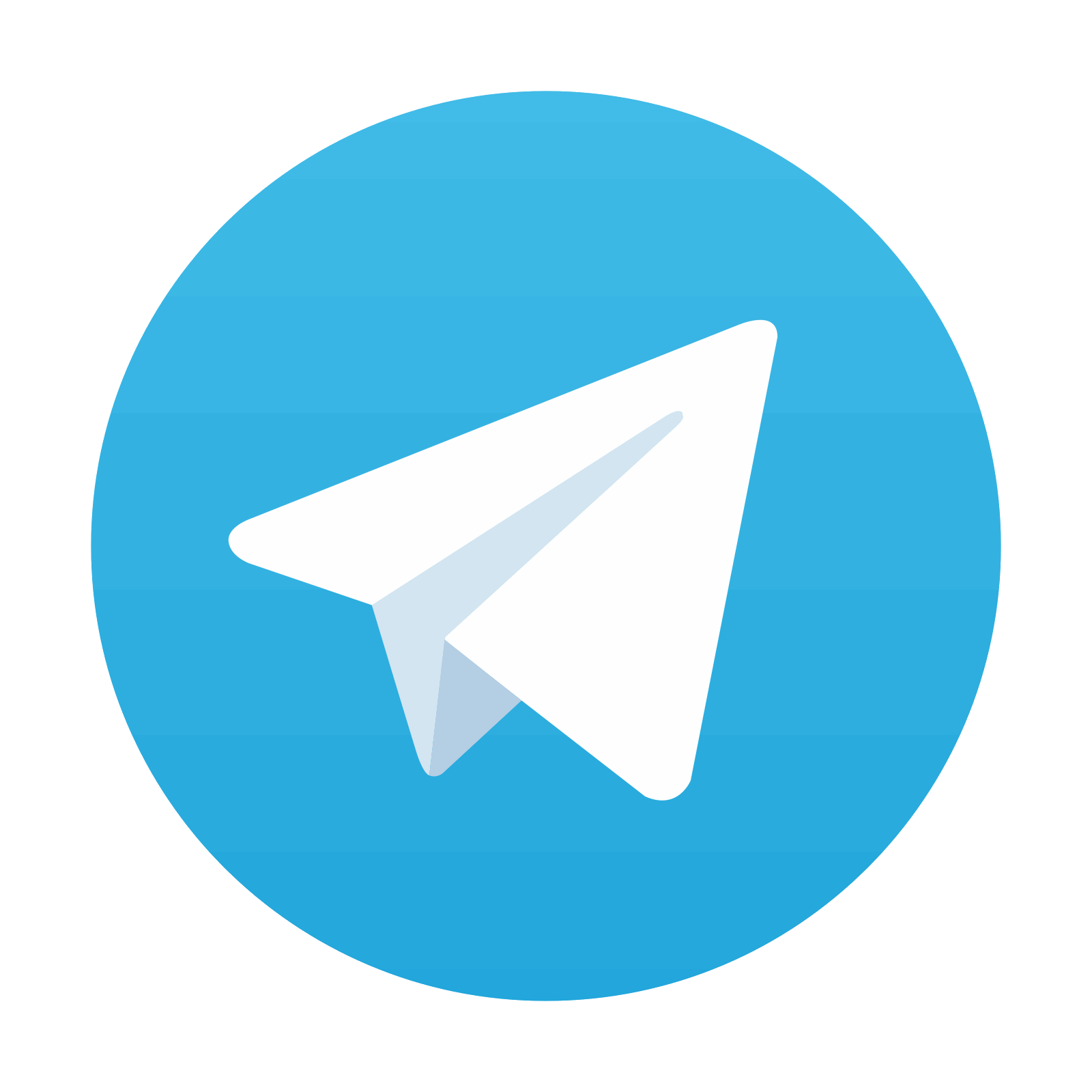
Stay updated, free articles. Join our Telegram channel

Full access? Get Clinical Tree
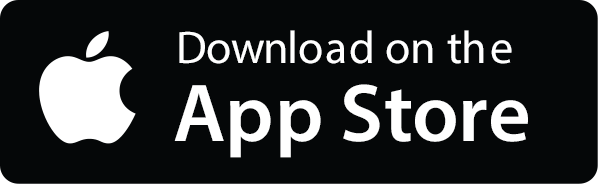
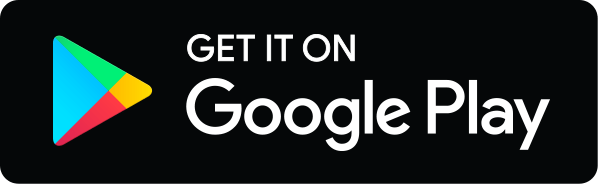
