KEY POINTS
- 1.
Persistent pulmonary hypertension of the newborn (PPHN) represents continued high pulmonary vascular resistance (PVR) after birth resulting in extrapulmonary shunting of the blood from pulmonary to systemic circulation, leading to hypoxemia.
- 2.
The disorder can be idiopathic or primary or could complicate respiratory, neurologic, and cardiovascular morbidities. Perinatal asphyxia with meconium aspiration syndrome or pneumonia can result in impaired lung recruitment, suboptimal oxygenation, and pulmonary vasoconstriction. Preterm neonates with severe respiratory distress syndrome could also have persistent high PVR after birth.
- 3.
Pathophysiological mechanisms such as pulmonary vascular remodeling, abnormal pulmonary vascular reactivity, and pulmonary vascular hypoplasia can contribute to PPHN.
- 4.
The molecular mechanisms of PPHN include abnormally high levels of pulmonary vasoconstrictors such as endothelin 1, leukotrienes, thromboxanes, and/or low levels of pulmonary vasodilators such as nitric oxide (NO) and prostacyclin.
- 5.
Neonates with PPHN are often managed using positive pressure ventilation, oxygen to correct hypoxemia, surfactant replacement therapy, vasopressors to maintain systemic pressures, pulmonary vasodilators, and adequate sedation with minimal stimulation.
- 6.
Regardless of treatment intervention, neonates with PPHN have 10% mortality along with poor pulmonary and neurodevelopmental outcomes. In neonates randomized to early inhaled NO with PPHN, 25% had impaired neurodevelopmental outcome and 22% had hearing impairment.
Introduction
Persistent pulmonary hypertension of the newborn (PPHN) is estimated to affect 1.9 per 1000 live births and is characterized by a failure of transition from fetal to newborn circulation. PPHN is secondary to the persistence of high pulmonary vascular resistance (PVR), resulting in extrapulmonary shunting of the blood from pulmonary to systemic circulation and leading to hypoxemia. It is often secondary to respiratory disease, although occasionally, PPHN can present as primary or idiopathic, often associated with “black lungs” on a chest x-ray due to absence of lung disease and pulmonary oligemia. PPHN could complicate the course of a sick newborn infant, leading to respiratory, neurologic, and cardiovascular morbidities and mortality. Commonly identified in term infants, increasing evidence supports the presence of PPHN in preterm neonates. Neonates with PPHN are often managed using positive pressure ventilation (PPV), oxygen to correct hypoxemia, surfactant replacement therapy, vasopressors to maintain systemic pressures, pulmonary vasodilators, and adequate sedation with minimal stimulation. According to the Extracorporeal Life Support Organization, 20% of neonates with PPHN required extracorporeal membrane support (ECMO), although early surfactant and inhaled nitric oxide (iNO) can potentially further reduce the need for ECMO. The focus of this chapter is to discuss the available evidence to guide management of PPHN.
Pathophysiology
Interference in the mechanism of transition during birth could lead to persistent elevation of pulmonary arterial pressures, leading to PPHN. PPHN is characterized by labile systemic arterial hypoxemia secondary to elevated PVR in relation to systemic vascular resistance (SVR), with resultant right-to-left (pulmonary to systemic circulation) shunting through persistent fetal channels such as the ductus arteriosus and foramen ovale, bypassing the lungs ( Fig. 13.1 ).

The transition from fetal to newborn circulation occurs at birth with aeration of the lungs, which drops PVR and increases blood flow to the lungs by 8- to 10-fold. , With clamping of the umbilical cord, there is an increase in SVR, increasing left ventricular afterload, and switching of the shunts at the foramen ovale and ductus arteriosus from right-to-left to left-to-right.
The following pathophysiological mechanisms can alter PVR and result in PPHN: pulmonary vascular remodeling, abnormal pulmonary vascular reactivity, and pulmonary vascular hypoplasia.
Pulmonary Vascular Remodeling
Idiopathic PPHN secondary to pulmonary vascular remodeling in the absence of lung pathology could present with histopathological features of smooth-muscle hypertrophy and extension of the muscular layer to more peripheral vasculature such as the preacinar arterioles. During fetal life, high pulmonary vascular pressures and resistance are maintained by humoral mediators such as endothelin 1, leukotrienes, and thromboxanes along with decreased levels of pulmonary vasodilators such as nitric oxide (NO) and prostacyclin (PGI 2 ). , , NO exhibits its effect on pulmonary vasodilation by increasing cyclic guanosine monophosphate (cGMP) in the smooth muscles, which causes relaxation ( Fig. 13.2 ). Higher endothelin 1 and lower cGMP could contribute to abnormal pulmonary vasculature, in turn contributing to the pathology. Urea cycle defects leading to low levels of arginine, the precursor of NO, could contribute to PPHN. Maternal ingestion of cyclooxygenase inhibitors such as aspirin during late pregnancy may be associated with ductal closure and lead to PPHN, although this association has been recently questioned. Maternal intake of selective serotonin reuptake inhibitors has also been associated with PPHN, possibly due to pulmonary vasoconstrictive effects of serotonin. ,

Abnormal Pulmonary Vasoconstriction
Optimal oxygenation leads to pulmonary vasodilation and also stimulates endogenous NO. Failure to establish adequate lung recruitment as observed in asphyxia with meconium aspiration syndrome (MAS) or pneumonia could lead to suboptimal oxygenation and ventilation, in turn leading to pulmonary vasoconstriction. Preterm neonates with severe respiratory distress syndrome (RDS) and neonates delivered by cesarean section in the absence of labor could have persistent high PVR after birth. An imbalance between pulmonary vasodilators and constrictors can lead to PPHN with increased vascular reactivity, remodeling, and high PVR. Lung parenchymal diseases such as MAS, pneumonia, and RDS can trigger proinflammatory mediators that could stimulate endothelin and thromboxane, leading to pulmonary vasoconstriction. Increased viscosity (such as severe polycythemia) can lead to pulmonary vascular obstruction and can elevate PVR ( Fig. 13.3 ).

Pulmonary Vascular Hypoplasia
Disruption in the development of pulmonary vasculature reduces the cross-sectional area, leading to high PVR. Pulmonary hypoplasia can occur in conditions such as congenital diaphragmatic hernia (CDH), oligohydramnios secondary to prolonged rupture of membrane (PROM), Potter syndrome, and renal disease. The closure or restriction of ductus arteriosus in a fetus secondary to maternal ingestion of nonsteroidal anti-inflammatory drugs may be associated with pulmonary vascular hypoplasia and remodeling (see above). , ,
Pulmonary Venous Hypertension
Anatomic and functional abnormalities in the pulmonary venous system and left heart can lead to pulmonary venous hypertension. These conditions include pulmonary vein stenosis, left ventricular dysfunction, hypoplastic left heart syndrome, and mitral stenosis. These conditions may present with intractable PPHN with poor response to traditional inhaled pulmonary vasodilators such as NO ( Fig. 13.4 )

Clinical Conditions Presenting With PPHN
Based on a Neonatal Research Network study, the primary respiratory illnesses associated with PPHN in the order of descending frequency were MAS (41%), pneumonia (14%), RDS (13%), pneumonia and/or RDS (14%), CDH (10%), and pulmonary hypoplasia (4%).
Meconium Aspiration Syndrome
A distressed fetus is at risk of exposure to meconium-stained amniotic fluid (MSAF), and approximately 5% to 10% of these newborns are at risk for MAS. MAS presents as acute respiratory failure with the need for mechanical ventilation. The chemical pneumonitis and surfactant inactivation secondary to meconium aspiration result in impaired gas exchange. A combination of hypoxemia, hypercarbia, acidosis, and impaired pulmonary vascular transition contributes to PPHN. The delivery room management of a newborn with MSAF has changed with the 2015 International Liaison Committee on Resuscitation recommendations based on available clinical and translational evidence. The current recommendations as of 2015 are to initiate PPV in the nonvigorous neonate and not to perform routine endotracheal suctioning to minimize the interruptions in initiating resuscitative steps. Although MAS is no longer the most common indication for neonatal respiratory ECMO, it continues to be a major contributor to PPHN (often associated with asphyxia) in low- and middle-income countries.
Pneumonia
Underlying parenchymal lung injury, along with elevated PVR, could lead to PPHN. These infants may or may not present with radiologic features of pneumonia. If pneumonia is associated with sepsis, it could lead to a drop in systemic pressures, which may further complicate PPHN.
Respiratory Distress Syndrome
RDS is a condition that primarily occurs in preterm neonates secondary to surfactant deficiency (93% of infants <28 weeks). Approximately 10% of late preterm neonates and 1% of term neonates could also have RDS. Newborns with RDS present with tachypnea, nasal flaring and grunting, intercostal retractions, and hypoxemia, presenting as cyanosis/color change secondary to intrapulmonary (ventilation–perfusion mismatch) and right-to-left extrapulmonary (across the ductus and foramen ovale) shunting. This condition is commonly managed with continuous positive airway pressure (CPAP), invasive mechanical ventilation with optimal mean airway pressure, and rescue surfactant therapy.
Congenital Diaphragmatic Hernia
Characterized by a defect in the diaphragm that leads to herniation of abdominal contents into the thorax, the incidence of congenital diaphragmatic hernia (CDH) ranges anywhere from 0.8 to 5 per 10,000 births. Pulmonary hypoplasia, pulmonary vascular remodeling, and the associated PPHN are the major factors contributing to hypoxemia in CDH. An underdeveloped left ventricle and a hypertrophied right ventricle leading to biventricular dysfunction could further complicate the PPHN in these neonates.
Pulmonary Hypoplasia
Besides CDH, pulmonary hypoplasia secondary to renal dysfunction, oligohydramnios, and/or skeletal abnormalities primarily affecting the thorax could be associated with PPHN.
Prematurity and PPHN
Traditionally a disease of term and late-preterm neonates, PPHN is increasingly diagnosed in extremely premature infants. , , PPHN in preterm neonates has a biphasic presentation: early and late onset. Early-onset PPHN is associated with RDS and occurs within the first few weeks after birth. Although late-onset PPHN is often associated with bronchopulmonary dysplasia (BPD), there are preterm babies with echocardiographic evidence of PPHN without BPD reported. Fetal growth restriction, premature PROM, oligohydramnios, and pulmonary hypoplasia are risk factors for PPHN in preterm neonates. , Preterm neonates who present with both acute and late-onset PPHN are at higher risk of mortality and morbidity.
Miscellaneous Causes
Other conditions such as alveolar capillary dysplasia and congenital surfactant deficiencies may present with PPHN requiring ECMO, and the disease process in these cases may not be reversible. Alveolar–capillary dysplasia with pulmonary vein misalignment could present beyond the neonatal age group and could pose a challenge to diagnose.
Diagnostic Features
PPHN presents with clinical features, which could be evaluated further by blood gas analysis, imaging (x-ray of the chest), and echocardiography. Echocardiography is also required to rule out congenital heart defects (CHDs) and estimate the severity of PPHN. Cardiac catheterizations are rarely performed to grade the degree and determine etiology of intractable PPHN.
Clinical Signs and Symptoms
The classic clinical feature is a newborn is labile hypoxemia with differential oxygen saturation (Sp o 2 ), that is, higher Sp o 2 in the right upper limb (preductal) compared with the lower-extremity Sp o 2 (postductal), which fluctuates with or without the need for oxygen and PPV. PPHN often presents as acute hypoxic respiratory failure (HRF), usually requiring oxygen >50% while on PPV.
Prenatally diagnosed CDH and perinatal factors such as nonreassuring fetal heart tracing, birth asphyxia, meconium-stained amniotic fluid, PROM, and oligohydramnios should prompt a physician to have a high index of suspicion for PPHN. PPHN should also be considered if hypoxemia and the oxygen requirement are disproportionate to the lung pathology. Preductal and postductal saturations (Sp o 2 ) should be monitored for labile hypoxemia and differential cyanosis (lower-limb Sp o 2 is lower than the right-upper-limb Sp o 2 ). The absence of an Sp o 2 difference could occur in PPHN if the shunting occurs at the level of the foramen ovale. A chest exam may reveal a barrel chest in cases of MAS secondary to air trapping, with variable lung sounds on auscultation along with rales and rhonchi. Secondary to PPHN, the cardiac examination could reveal a loud second heart sound and a harsh systolic murmur best heard along the left sternal border secondary to tricuspid regurgitation.
Peripheral Saturations and the Utility of Blood Gas
Hypoxemia could also be secondary to heart defects, and it is important to differentiate PPHN from cyanotic CHD. A higher preductal-to-postductal saturation difference greater than 5% to 10% or an arterial oxygenation (Pa o 2 , in mm Hg) difference of greater than 10 to 20 mm Hg is suggestive of PPHN. In the absence of ductal shunting, both preductal and postductal saturations could remain low. In a condition such as coarctation of the aorta in the presence of ductus, differential cyanosis could be seen. In CHD, the low saturations persist despite oxygen supplementation, unlike in PPHN. In centers without immediate access to echocardiography or a cardiologist, the hyperoxia test could help differentiate CHD, PPHN, and lung disease causing hypoxemia.
Hyperoxia Test
Assessing a change in Pa o 2 in arterial blood gas (ABG) before and after exposing a neonate to 100% supplemental oxygen could help differentiate PPHN from parenchymal lung disease and CHD. After exposing the neonate to 100% O 2 , in a healthy neonate, the Pa o 2 is usually >300 mm Hg. In a newborn with CHD, after a hyperoxia test, the Pa o 2 is <100 mm Hg, and in a newborn with PPHN, it can be >100 mm Hg but is typically <150 mm Hg. In parenchymal lung disease, the Pa o 2 could be between 150 and 300 mm Hg. A hyperoxia test is not a gold standard test and could be falsely negative or positive in cardiac lesions with high pulmonary blood flow. In the presence of easy access to echocardiography, a hyperoxia test should be avoided to limit oxygen toxicity and to avoid ductal closure and an excessive drop in PVR in the presence of ductal-dependent systemic circulation (such as hypoplastic left heart syndrome).
In the past, hyperventilation-induced alkalosis and hyperoxia were used to diagnose PPHN. Both oxygen and alkalosis induce pulmonary vasodilation and improve oxygenation in PPHN and could differentiate it from cyanotic CHD. However, respiratory alkalosis reduces cerebral blood flow and is associated with negative neurodevelopmental outcomes, and it should be avoided.
Severity of Hypoxemia in PPHN
Hypoxemia can be assessed by ventilator settings, ABG parameters, and oxygen saturations. The indices mentioned below can be used to assess severity and response to therapy in PPHN: the oxygenation index (OI); arterial oxygenation, the ratio of the partial pressure of oxygen in arterial blood (Pa o 2 ) to the fraction of inspired oxygen (Fi o 2 ) (P/F ratio); the A-a (alveolar-arterial) oxygen gradient; and the oxygen saturation index (OSI).
Oxygenation Index
The equation to calculate the OI is
FiO2*100*(mean airway pressure[MAP]in cm H2O)÷(PaO2in mm Hg).
Based on OI, HRF could be classified as
- 1.
Mild (OI ≤ 15)
- 2.
Moderate (OI > 15 and < 25)
- 3.
Severe (OI 25–40)
- 4.
Very severe (OI > 40)—an indication for ECMO
P/F Ratio
The severity of the P/F ratio, or the ratio of the Pa o 2 to the Fi o 2 in mm Hg, can be assessed as follows:
- 1.
Mild: >200 to 300
- 2.
Moderate: >100 to 200
- 3.
Severe: 100
Alveolar-Arterial Gradient
The A-a gradient provides an estimate of the oxygen gradient between the alveolar and the arterial side. It is estimated as
A-agradient=(FiO2*[barometricpressure−watervaporpressure]−[PacO2/R]−PaO2),
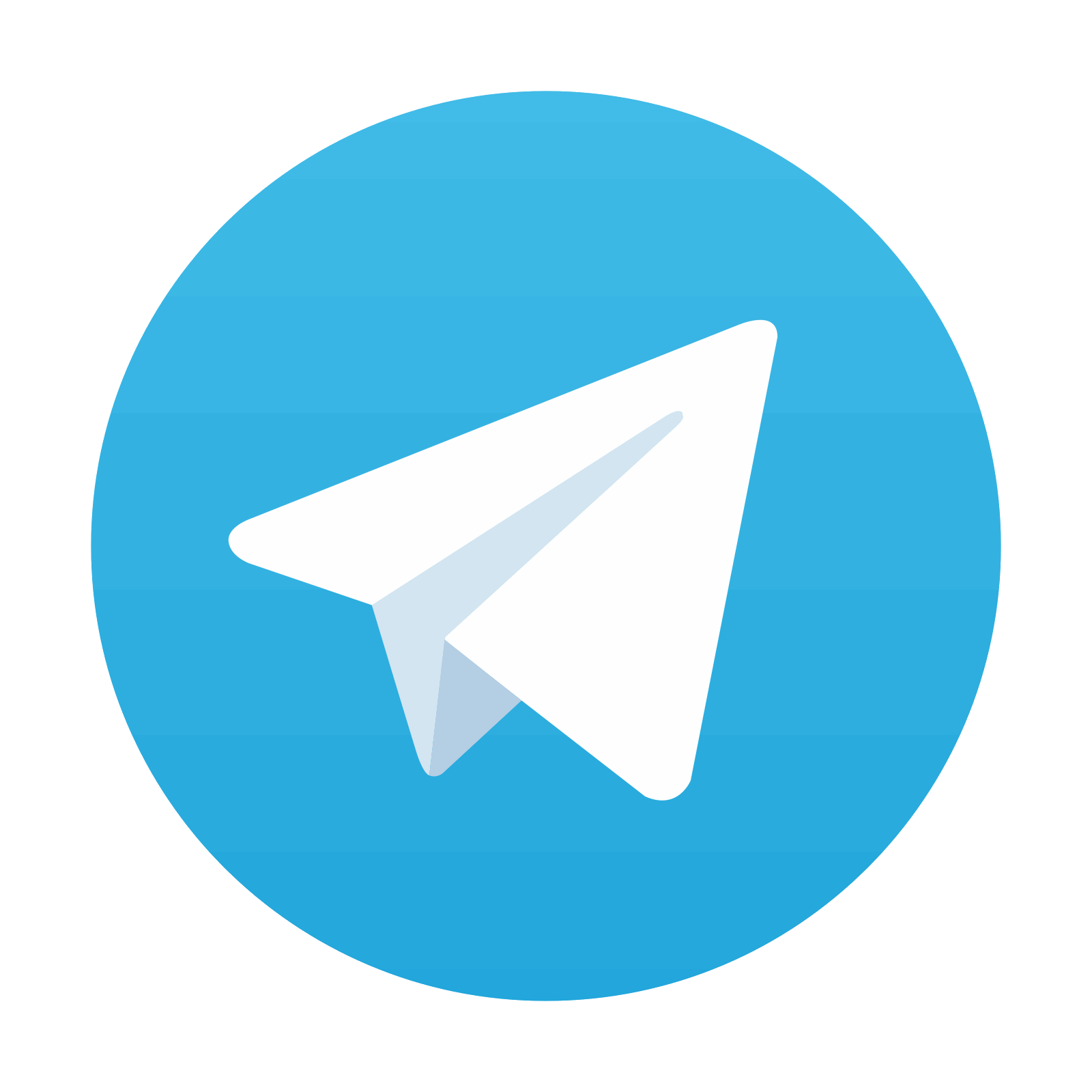
Stay updated, free articles. Join our Telegram channel

Full access? Get Clinical Tree
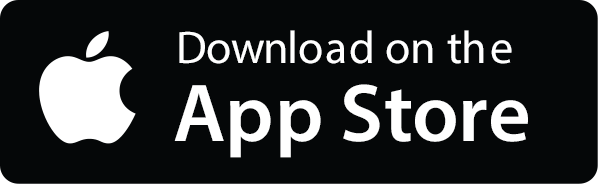
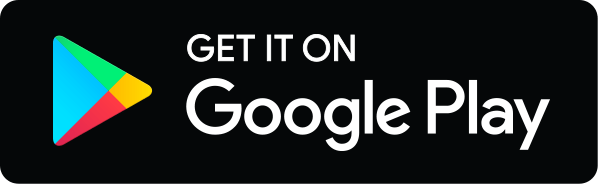
