Introduction
Testing is available for an ever-increasing number of genetic disorders. Although prenatal testing originally focused primarily on Down syndrome, it is now possible to detect a broad range of genetic conditions. Prenatal diagnostic testing is most commonly performed on fetal tissue obtained with amniocentesis or chorionic villus sampling (CVS), although umbilical cord blood obtained through percutaneous sampling is occasionally used. In the early embryo, preimplantation genetic testing (PGT) is also used for genetic diagnosis in families at risk for specific genetic disorders (see Chapter 15 ). The focus of this chapter is on CVS and amniocentesis, as these are the techniques most commonly used for routine prenatal testing.
Prenatal genetic testing is focused on every woman’s values and preferences, which vary greatly. Before undergoing prenatal diagnostic testing, women should understand the benefits and limitations of such testing, the conditions that are being tested for, as well as the conditions that will not be detected, and the risks of the procedure. Prenatal genetic testing can provide reassurance when results are normal, identify disorders for which in utero treatment might be available, improve neonatal outcomes by assuring the best location for delivery and that appropriate pediatric specialists are available to care for affected infants, and can also provide the opportunity for pregnancy termination for women who choose that option.
Fetal genetic disorders that are amenable to prenatal genetic testing include those abnormalities in structure or function that are caused by variants in an individual’s genes, in contrast to those caused primarily by environmental or other disruptive causes; these latter conditions may be best identified by imaging. These distinctions are not always clear, as a genetic predisposition may increase the susceptibility to environmental influences, and some genetic abnormalities may only become apparent under specific environmental conditions or circumstances. Some disorders may have an epigenetic basis; in other words, gene functions may be turned on or silenced by modifications that may depend on the parent of origin or other influences (see Chapter 2 ). With improvements in our ability to study genetics and genomics, it is increasingly appreciated that inheritance and genetics are complex and our understanding is still relatively limited. For this reason, prenatal diagnosis can be complicated, and it is not always possible to predict the outcome based on a prenatal genetic test. It is important that patients understand that prenatal diagnostic testing is possible for many, but not all, genetic disorders.
What Conditions Can Be Diagnosed by Prenatal Genetic Testing?
In general, chromosomal abnormalities and single gene disorders can be identified by analysis of fetal tissue, and it is these categories of conditions that are most often the target of prenatal diagnostic testing. Chromosomal abnormalities are relatively common in pregnancy, and about 1/150 live births has a chromosomal abnormality that causes an abnormal phenotype. About 5% of stillbirths and 5–7% of infant and childhood deaths result from chromosomal abnormalities, and chromosomal abnormalities are commonly associated with structural fetal abnormalities. Copy number variants (CNVs), which can be detected with chromosomal microarray analysis (CMA), are present in about 1–1.7% of structurally normal fetuses and about 6% of those with an abnormality detected by ultrasound.
Chromosomal abnormalities include variations in chromosome number or structure. The most common abnormality of chromosome number found by prenatal testing is aneuploidy, which is the presence of an extra or missing chromosome or chromosomes. It is also possible to have one or more extra sets of chromosomes, as in triploidy or tetraploidy. Abnormalities in chromosome number can be mosaic, in which the abnormal number of chromosomes is not present in all cells (see Chapter 4 ).
In addition to abnormalities of chromosome number, there can also be aberrations in chromosome structure, such as deletions, duplications, translocations, and other rearrangements. In some cases, rearrangements are balanced, meaning the appropriate genomic content is present but rearranged, whereas in other cases, translocations or other rearrangements can result in extra or missing pieces of chromosomes. Balanced translocations most often are associated with a normal phenotype, although they can lead to recurrent miscarriage or an increased risk of abnormal offspring. Deletions and duplications can be quite large, and easily seen by a karyotype, or can be small microdeletions or duplications only detectable with chromosomal microarray analysis (CMA), fluorescence in situ hybridization (FISH), or other specialized methods (see Chapter 6 ) ( Table 14.1 ).
Test | Turnaround Time | Conditions Detected | Comments |
---|---|---|---|
Karyotype | 7–14 d | Chromosomal abnormalities >5–10 Mb | Traditional method for diagnosis of chromosomal abnormalities |
Fluorescence in situ hybridization (FISH)—Interphase | 24–48 h | Rapid assessment of major aneuploidies (typically chromosomes 13,18,21,X,Y) | Less accurate with chorionic villus sampling versus amniocentesis |
FISH—Metaphase | 7–14 d | Microdeletions and duplications | Can be used to test for specific abnormalities when clinically suspected; CMA often preferable |
Chromosomal microarray analysis (CMA) | 3–5 d (direct); 10–14 d (cultured cells) | Copy number variants (CNVs) >50–200 kb | Whole genome screen for CNVs. Detects major chromosomal abnormalities except balanced rearrangements and some triploidies |
Molecular DNA testing | 3–14 d (faster with direct testing than when cultured cells are required) | Genetic variants previously demonstrated to be present in a family or suspected based on ultrasound or other findings | Usually a targeted test focusing on a specific disorder (or category of disorders) suspected to be present in a fetus based on ultrasound findings or family history |
Gene panels | 10–14 days | Sets of genes or gene regions that are associated with common features or phenotypes, such as skeletal dysplasia | Often not as comprehensive as targeted single gene testing |
Whole exome sequencing | Several weeks to months | Sequencing of all or most of the protein-coding genes in a genome | Often difficult to interpret in prenatal cases |
Some genetic disorders, such as cystic fibrosis, hemophilia, and Tay–Sachs disease, are caused by variants in single genes. Diseases caused solely by abnormalities in a single gene are relatively uncommon. The phenotype of some single gene disorders can be impacted by modifying genes, or by combinations of additional genes, as well as by environmental influences and therefore not all individuals who inherit a genetic variant will have the exact same phenotype. Single gene disorders can be detected by prenatal diagnostic testing if the disorder has been diagnosed with certainty and the particular variant within the family has been identified.
The most common congenital anomalies are isolated birth defects, such as heart defects, neural tube defects, and facial clefts. These traits are determined by multiple genes and environmental factors rather than by single genes. Because there is a genetic component, they often recur more commonly within a family. However, because they are not caused by a single gene variant but rather a complex interplay of genetic and environmental factors, prenatal diagnostic genetic testing is not available using specific DNA methods; rather diagnosis is usually made by ultrasound or other imaging. In some cases, an apparently isolated structural anomaly is associated with a cytogenetic abnormality or CNV, therefore karyotyping and/or chromosomal microarray analysis is recommended when one or more structural abnormalities is identified by ultrasound (see Chapter 12 ).
Although most genes are encoded in the nuclear genome, the mitochondria each contain their own genome. Variants can also occur in the mitochondrial DNA, and a number of mitochondrial diseases are due to these disorders. Mitochondria are essential for aerobic respiration, and mitochondrial diseases commonly affect tissues with high-energy requirements, such as the central nervous system, heart, and muscle. Mitochondria are all maternally inherited, and prenatal diagnosis for mitochondrial diseases can be complex and clinical outcomes are difficult to predict, due to variation in the number of abnormal mitochondria and the association with predicted phenotype (see Chapter 2 ).
How Is Prenatal Diagnostic Testing Performed?
Prenatal diagnostic testing refers to genetic testing done on fetal tissue obtained most commonly by amniocentesis or CVS. Less commonly, fetal blood is obtained through percutaneous umbilical blood sampling. Testing of early embryos can also be done in conjunction with in vitro fertilization in a procedure known as preimplantation genetic testing (PGT) (see Chapter 15 ). Finally, fetal cell-free DNA (cfDNA) or potentially nucleated fetal cells can be obtained from the maternal circulation and tested for genetic disorders. However, such techniques at present are considered screening methods and are discussed elsewhere in this book (see Chapter 9 ).
Cytogenetic or karyotype analysis requires viable cells that can be cultured; such cells can be obtained by CVS, amniocentesis, or fetal blood sampling. DNA for molecular testing (including chromosomal microarray analysis) can be obtained from any cell with a nucleus, regardless of viability, including blood lymphocytes, skin, hair, cheek cells or saliva, and paraffin tissue blocks. Cultured amniocytes, chorionic villi, and fetal blood are tissues that can be used for prenatal DNA testing of the fetus; if adequate tissue is obtained, DNA can be extracted without the need for culture. Other fetal tissue biopsy, such as biopsy of fetal skin, muscle, or liver, was done in the past for direct measurement of fetal enzyme activity or other physiologic parameters. Such biopsies have been largely replaced by molecular DNA methods that can directly detect the underlying genetic basis of many congenital disorders (see Table 14.1 ).
Amniocentesis
Genetic amniocentesis is usually performed between 15 and 20 weeks of gestation. Typically, a 22-gauge spinal needle is inserted into the amniotic sac under ultrasound guidance, and approximately 20 mL of amniotic fluid is withdrawn. It is common to avoid penetrating the placenta to decrease the chance of causing bleeding into the amniotic fluid, which can lead to difficulty in culturing cells and can also lead to false-positive amniotic fluid alpha-fetoprotein measurement. In addition, placental penetration can potentially lead to alloimmunization if there is maternal-fetal red cell incompatibility. However, transplacental amniocentesis does not appear to be associated with an increased risk of pregnancy loss.
Chorionic Villus Sampling
CVS is typically performed between 10–13 weeks of gestation. Placental villi may be obtained through either a transcervical or transabdominal approach. With transcervical CVS, a specialized catheter is inserted through the cervical os and guided into the placenta under ultrasound guidance. The transabdominal procedure is more similar to amniocentesis, and a needle (typically 20-gauge) is inserted into the placenta under ultrasound guidance. Typically, 15–25 mg of chorionic villi are obtained.
Although there are limited data comparing transcervical and transabdominal CVS, there does not appear to be a significant difference in fetal loss rates between the two approaches. The primary advantage of CVS over amniocentesis is that results are available earlier in pregnancy, which provides reassurance for parents when results are normal and, when results are abnormal, may allow for earlier and safer pregnancy termination. In addition, for patients in whom specialized direct DNA testing is to be performed, CVS samples are more cellular and provide larger amounts of DNA for direct analysis, allowing a more rapid turnaround time.
What Are the Risks of Different Prenatal Diagnosis Procedures?
The procedure-related loss rate of midtrimester amniocentesis at 16–20 weeks’ gestation has decreased over time, likely because of increasing experience, as well as improvements in imaging. Patients at less than 24 weeks’ gestation have a background loss rate without an invasive procedure, and this needs to be accounted for in calculating the excess loss rate due to amniocentesis or other tests. A recent metaanalysis of miscarriage risk after amniocentesis, including more than 42,000 women who underwent a procedure and 138,000 women who did not, estimated the loss rate because of the procedure at approximately 0.11% (95% CI, −0.04 to 0.26) or 1 in 900. In this large study, the miscarriage rate was not statistically different in patients who did, or did not, have a procedure ( P = .14). These data were collected relatively recently, so represent contemporary statistics. Importantly, these data were collected from high-volume, experienced centers and may not apply to small sites or low-volume providers.
Minor complications from amniocentesis, including transient vaginal spotting or amniotic fluid leakage, occur after approximately 1%–2% of procedures. The outcomes of amniotic membrane rupture after amniocentesis are far better than after spontaneous ruptured membranes; the perinatal survival rate after amniotic fluid leakage following midtrimester amniocentesis is greater than 90%. Direct injury to the fetus during amniocentesis has been reported but is rare when amniocentesis is performed under continuous ultrasound guidance. Amniotic fluid cell culture failure occurs in 0.1% of samples. In numerous studies, it has been reported that pregnancy loss, and complications such as blood-contaminated specimens, leaking of amniotic fluid, and the need for more than one needle puncture are related to the experience of the operator, the use of small-gauge needles, and ultrasound guidance.
As with amniocentesis, determination of procedure-related loss rates after CVS requires consideration of the background loss rate, which is substantially higher in the first trimester during the gestational age window when CVS is performed. As with amniocentesis, the loss rate from CVS has decreased over time. The most recent metaanalysis, including 54,000 women who had CVS and 670,000 who had no procedure, reported a procedure related loss rate of 0.1%. Including only those studies that specifically included a control group, the procedure-related loss rate was 0.22% (95% CI, −0.71–1.16). As in the analysis of amniocentesis, the loss rate in those patients who had a CVS procedure was not higher than the background rate ( P = .638).
Once again, an important caveat is that these data are from experienced, high-volume centers and may not apply to lower volume, less experienced providers. In several studies, it has been shown that there is a significant learning curve associated with the performance of CVS.
Although there have been reports of associations between CVS and limb reduction and oromandibular defects, the risk for these anomalies appears to be low and to correlate with procedures performed at <10 weeks’ gestation. In an analysis by the World Health Organization, an incidence of limb-reduction defects of 6 per 10,000 was reported in infants to women who had undergone CVS, which is not significantly different from the incidence in the general population. An increased risk of hemangiomas in the newborn has also been reported after CVS, primarily after transcervical CVS, in some but not all studies. Hemangiomas are generally benign and resolve without treatment in early childhood. Other complications after CVS include vaginal spotting or bleeding, which occurs in up to 32% of patients after transcervical CVS and at a lower rate after transabdominal CVS. The incidence of culture failure, amniotic fluid leakage, or infection after CVS is less than 0.5%.
Early amniocentesis has been reported between 11 and 13 weeks of gestation using a technique similar to midtrimester amniocentesis. However, early amniocentesis has been found to result in significantly higher rates of pregnancy loss and other complications compared with midtrimester amniocentesis. In a multicenter randomized trial, the spontaneous pregnancy loss rate after early amniocentesis was 2.5%, compared with 0.7% with traditional amniocentesis. In addition, the incidence of club foot was markedly increased, occurring in 1.4% after early amniocentesis compared with 0.1% (comparable with the background rate) after midtrimester amniocentesis. Membrane rupture was also more likely after early amniocentesis, and significantly more amniotic fluid culture failures occurred. For these reasons, amniocentesis at less than 14 weeks of gestation is no longer recommended and is rarely performed as CVS is considered a safer early option. Most data on the safety of amniocentesis are based on studies of procedures performed after 16 weeks’ gestation, and the safety and complication rates of procedures undertaken between 14 and 16 weeks are less certain.
Chromosomal mosaicism, the presence of more than one cell line identified during cytogenetic analysis, occurs in approximately 0.25% of amniocentesis specimens and 1–2% of chorionic villus specimens. After mosaicism is found using CVS, amniocentesis typically is performed to assess whether mosaicism is present in amniocytes. In most cases, approximately 90%, the amniocentesis result is normal, and the mosaicism is assumed to be confined to the trophoblast. Although such confined placental mosaicism (CPM) is unlikely to cause congenital abnormalities in the fetus, it may result in third trimester growth restriction.
Clinical outcomes of fetal mosaicism depend on the specific mosaic cell line(s) and may range from normal to findings consistent with the abnormal chromosome result. Counseling after the finding of chromosomal mosaicism is complex, and referral for genetic counseling may be useful in these cases. In the past, cordocentesis was often performed for further evaluation; more recently, it has been recognized that this adds little to the prediction of outcome. Placental mosaicism can also lead to false-positive results from noninvasive prenatal testing, which is based on testing of DNA thought to arise from trophoblasts.
CPM can be associated with so-called trisomy rescue of an originally trisomic conception. When this occurs, the fetus may be disomic but have uniparental disomy (UPD), a condition in which both chromosomes were inherited from the same parent. Trisomy rescue and UPD can potentially involve any chromosome, but if imprinted genes are present on the particular chromosome involved, this may have consequences on the fetus. Therefore, testing for UPD is indicated as a follow-up to CPM detected by CVS when a chromosome containing known imprinted genes is involved (e.g., chromosomes 6, 7, 11, 14, 15, and 20). If the chromosome involved in the original trisomy does not contain imprinted genes, no phenotypic consequences for the fetus would be predicted (see Chapter 2 ).
Mosaicism can also be suggested when the fetal specimen contains maternal cell contamination. This can be minimized by discarding the first 1–2 mL of the amniocentesis specimen and by careful dissection of chorionic villi from maternal decidua.
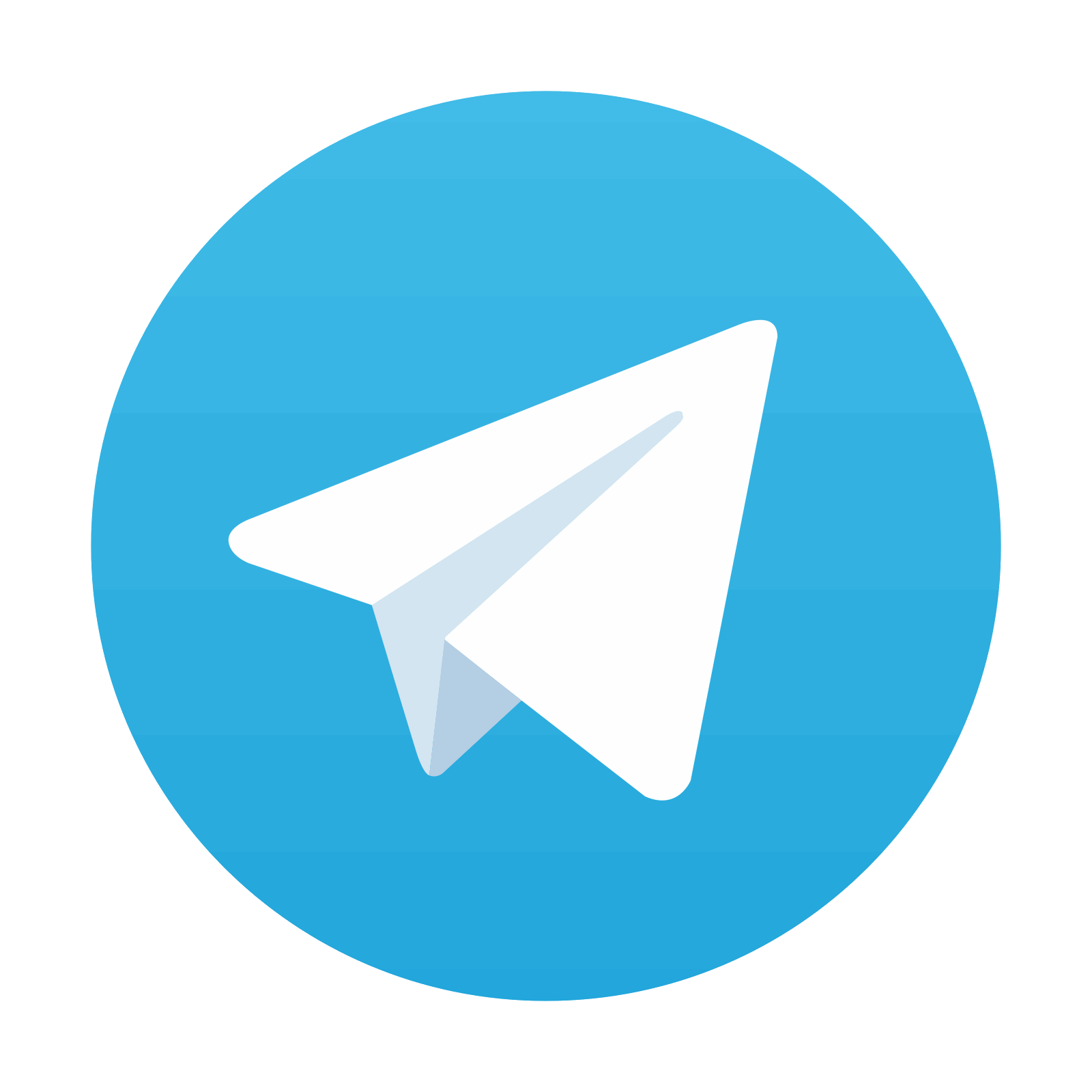
Stay updated, free articles. Join our Telegram channel

Full access? Get Clinical Tree
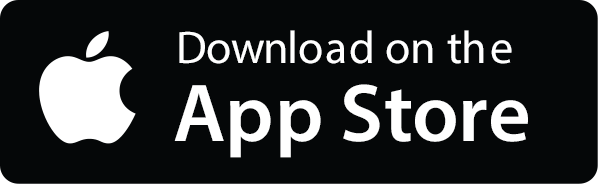
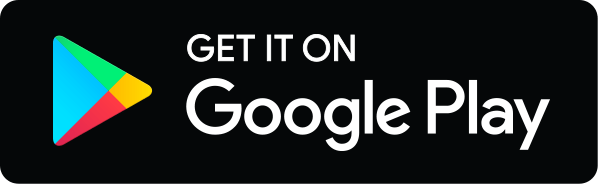