Introduction
For more than half a century, classical cytogenetics was the standard of care in the diagnosis of developmental disabilities and congenital anomalies. More recently, the introduction of microarray technology into clinical medicine has allowed the identification of subchromosomal abnormalities leading to the diagnosis of an increasing number of genetic conditions in both the fetus and the child. A chromosomal microarray analysis (CMA) is a high-resolution genomic technology with the ability to detect the same pathogenic chromosomal imbalances detectable via karyotype as well as smaller submicroscopic deletions and duplications, known as copy number variants (CNVs), which are often associated with congenital anomalies and intellectual disabilities.
In 2010, a consensus statement was published establishing CMA as the first-tier clinical diagnostic test in the pediatric setting for individuals with developmental disabilities, congenital anomalies, or dysmorphic features, given that CMA can identify the cytogenetic etiology in 15%–20% more patients than a G-banded karyotype. Subsequent studies in the prenatal setting found that pathogenic CNVs were present in 1%–1.7% of structurally normal fetuses and 6%–7% of anomalous fetuses.
In 2013, The American College of Obstetricians and Gynecologists (ACOG) and The Society for Maternal-Fetal Medicine (SMFM) jointly recommended that CMA should replace or supplement karyotype in the evaluation of a fetus with anomalies. It is also recommended that CMA be made available to any patient choosing prenatal diagnostic testing for any indication, including anxiety. This recommendation confirms their opinion that all women be offered the option of prenatal diagnostic testing regardless of age and is based on the fact that CNVs occur with equal frequency at all ages. Notably, the rate of pathogenic CNVs is higher than the risk of Down syndrome in women under 36 years of age, and the risk for a pathogenic CNV is four times higher than the risk of trisomy 21 in a woman under 30 years of age.
In the last decade, advances have occurred in aneuploidy screening, including use of cell-free DNA (cfDNA), as well as in the amount of genetic information available through diagnostic testing using CMA. These simultaneous occurrences have created new counseling challenges and the need for increased patient education. Although our ability to reliably screen for a few common aneuploidies (i.e., trisomies 13, 18, 21) has dramatically improved, our ability to diagnose hundreds of additional conditions (e.g., DiGeorge syndrome, Prader–Willi syndrome, and cri-du-chat syndrome) by chorionic villus sampling (CVS) or amniocentesis has also dramatically improved. This has led to some laboratories incorporating a subset of CNVs into their cfDNA screening algorithms. Although this approach is not presently as accurate as diagnostic testing, it demonstrates the increasing emergence of molecular techniques into screening.
What Is a Microarray and How Does the Technology Compare With a Karyotype?
Karyotyping visualizes chromosomes under the microscope and allows detection of large structural alterations such as aneuploidy, large deletions and duplications, marker chromosomes, and rearrangements such as translocations and inversions. In clinical prenatal diagnostic analysis, the G-banded karyotype has a resolution of 7–10 million base pairs (MB). Diagnosis of smaller findings is limited, and there is variation between different pre- and postnatal preparations. In addition, interpretation may be somewhat subjective since the assessment is completed visually, creating a risk for discrepancy between laboratories. The karyotype also requires the use of living, cultured cells, which often take 2 weeks to grow and have a risk for culture failure.
Chromosomal microarray analysis (CMA) uses genomic technology to identify small subchromosomal gains and losses called CNVs. CMA has a much higher resolution than a karyotype, potentially detecting gains and losses as small as approximately 50 kilobases (KB). CMA can be completed using extracted DNA from cultured or uncultured (direct) cells, giving it a quicker turnaround time (potentially 3–5 days), which is particularly important for patients pursuing prenatal diagnosis for pregnancy management decisions. The ability to run a microarray on uncultured cells also allows for testing nonviable tissues from an intrauterine demise or spontaneous loss. Lastly, the CMA is interpreted using computer algorithms in combination with laboratory director expertise, allowing a less subjective interpretation. Two different CMA technologies exist with many laboratories using both in combination.
Array comparative genomic hybridization (aCGH) arrays use short (25–50 base pairs) laboratory-made sequences of single-stranded DNA called oligonucleotides and are designed to detect copy number alterations (gains or losses of genetic information) compared with a reference control at specific genomic locations (see Fig. 12.1 ). In this method, patient and control DNA samples are cut into fragments, labeled with fluorescent colors (typically green and red), mixed together, and placed on an array, which contains millions of oligonucleotide probes from multiple human genome reference sequences. The mixture of DNAs hybridize (bind) to complimentary sequences on the array and the intensity of fluorescence is measured, allowing for the detection of a gain or loss of genetic material in the sample compared with the control.

Single-nucleotide polymorphism (SNP) arrays do not use a reference sequence; rather, they harness the power of polymorphisms in the genome. SNPs are differences in a single nucleotide within a stretch of DNA that are present in the population at a frequency of greater than 1% and are the most common form of genetic variation between individuals. An example of an SNP is the nucleotide thymine (T) replacing the nucleotide cytosine (C) at a specific location of DNA in a subset of the human population. Because SNPs occur normally in about every 300 nucleotides on average, and there are about 10 million SNPs in the human genome, they are an excellent target for the detection of gains or losses of genomic blocks of information (see Fig. 12.2 ).

The aCGH CMA is more limited than an SNP CMA because it can only detect gains and losses (CNVs), whereas SNPs allow the identification of a unique “genetic fingerprint,” which is important for numerous reasons. Because each individual inherits a paternal and a maternal SNP at each location, a long contiguous stretch of homozygosity (LCSH), in which SNPs from only one parent are seen, can be identified and may represent either uniparental disomy (UPD) for a segment or a whole chromosome, or consanguinity. UPD is associated with genetic disease when an imprinted chromosome is involved (chromosomes 6, 7, 11, 14, 15) or if a pathogenic variant in a recessive disease gene is included in the region of homozygosity, so that the proband is then homozygous for that variant. When an individual has consanguineous parents, the number of LCSHs will directly correlate with the degree of relationship. The use of SNPs also allows for the detection of triploidy, which is not detectable by aCGH as all chromosomes are duplicated. SNP microarrays can be used for the detection of parentage (maternity and paternity) compared with an alleged parents’ DNA and can determine zygosity when testing samples from multiple gestations (see Table 12.1 ).
Technology | Aneuploidy | Balanced Translocations and Inversions | Unbalanced Translocations | Triploidy | Long Contiguous Stretch of Homozygosity, Consanguinity, Zygosity, and Parentage | Copy Number Variants | Culture Required |
---|---|---|---|---|---|---|---|
G-banded karyotype | Yes | Yes | Yes | Yes | No | No | Yes |
Comparative genomic hybridization array | Yes | No | Yes | No | No | Yes | No |
SNP array | Yes | No | Yes | Yes | Yes | Yes | No |
What Is the Difference Between a Targeted Microarray and a Whole Genome Microarray?
Many laboratories offer a “targeted” CMA and “whole genome” CMA, sometimes termed a “prenatal” versus a “postnatal” microarray, respectively. The ability of a CMA to identify CNVs depends on the number of unique probes on the array platform. Some laboratories use assays only targeting known genetic conditions and small CNVs that are known to be clinically relevant. Targeted arrays have probes restricted mainly to the genes known to be associated with those conditions. These targeted arrays will often have additional coverage (called the backbone) with probes spread equidistant across the genome (most commonly every 1 MB) in addition to more closely placed probes in the targeted regions. Incorporation of the backbone allows detection of larger and potentially significant deletions or duplications regardless of where they occur. Others have chosen to use whole genome arrays with dense coverage for a wider range of known conditions and a denser backbone to detect CNVs that may be clinically relevant because of size and/or Online Mendelian Inheritance in Man (OMIM) gene disease content, even if the CNV is not part of a well-described microdeletion/microduplication syndrome. Some labs perform the same density array in all cases but may mask some portions of the data for prenatal interpretation in an attempt to reduce findings of uncertain significance. Although there are no standardized guidelines governing prenatal cases, many laboratories will maintain a CNV minimum size cutoff for reporting variants of uncertain significance (VUSs) (i.e., 1 MB or greater) and some may limit reporting of LCSH regions to imprinted chromosomes. The clinician should be familiar with the difference in assays and/or reporting standards for their laboratory of choice and, at times, be prepared to discuss the differences with a patient.
The American College of Medical Genetics and Genomics (ACMG) guidelines recommend that whole genome microarrays have a minimum detection of gains and losses of 400 kb or larger and an enrichment of probes in regions with known dosage-sensitive genes with strong correlation to congenital anomalies or neurocognitive impairment. A whole genome array was previously thought to increase the likelihood for a laboratory to report a VUS, but studies assessing this have shown this not to be the case.
What Can a Microarray Detect That a Karyotype Cannot?
CMA has a higher resolution than a karyotype, allowing for the detection of smaller CNVs in addition to aneuploidies and large chromosomal deletions and duplications. (See Table 12.2 for a list of common microdeletion/duplication syndromes). When a cryptic result is reported on a karyotype, such as a marker chromosome, CMA may be used to discern the origin; this can be helpful in determining the clinical significance and prognosis. CMA can also be used when apparently balanced rearrangements are identified on a karyotype to determine if it is balanced or if there is a small deletion or duplication at the breakpoint(s). As outlined above and in Table 12.1 , the SNP CMA additionally has the power to detect regions of homozygosity highlighting consanguinity and UPD, which may present an increased risk for recessive disease. SNP arrays can also determine parentage and in the case of twins, zygosity.
Condition; Genomic Location | Incidence | Major Phenotypic Features |
---|---|---|
16p11.2 duplication | 1/1900 | Normal to DD, ASD, ADHD, microcephaly, psychiatric conditions |
16p11.2 deletion | 1/2300 | ID/DD, ASD, ADHD, macrocephaly, psychiatric conditions |
16p13.11 deletion | 1/2300 | ID/DD, seizures, schizophrenia |
1q21.1 duplication | 1/3300 | Normal to motor skill and articulation difficulty, ID/DD, ASD, ADHD, scoliosis, abnormal gait, macrocephaly, short stature, psychiatric conditions (schizophrenia, anxiety, depression), CHD (especially Tetralogy of Fallot) |
22q11.2 deletion syndrome (DiGeorge, VCFS) | 1/4000 | CHD (most commonly conotruncal), palate abnormalities, characteristic facies, ID/DD, immune deficiency, hypocalcemia, psychiatric conditions in early adulthood (schizophrenia, depression, bipolar disorder) |
22q11.2 duplication | 1/4000 | Normal to ID/DD, growth retardation, hypotonia |
1p36 deletion syndrome | 1/5000 | ID/DD, hypotonia, seizures, structural brain abnormalities, CHD, vision and hearing issues, skeletal anomalies, characteristic facies |
Charcot–Marie–Tooth type 1A; 17p12 duplication | 1/5000–1/10,000 | Slowly progressive neuropathy causing distal muscle weakness and atrophy, sensory loss, and slow nerve conduction velocity first noticeable in the first or second decade |
X-linked ichthyosis; Xp22.31 deletion | 1/6000 | ID/DD, ichthyosis, Kallmann syndrome, short stature, ocular albinism |
Williams syndrome; 7q11.23 deletion | 1/7500 | ID/DD, cardiovascular disease, characteristic facies, connective tissue abnormalities, specific personality, growth anomalies, endocrine abnormalities |
7q11.23 duplication | 1/7500 | DD, normal to ID intellectually, speech problems, hypotonia, problems with movement and walking, behavioral abnormalities, seizures, aortic enlargement |
Prader–Willi syndrome; 15q11.2 paternal deletion | 1/10,000 | ID/DD, hypotonia and feeding difficulties in infancy, excessive eating, obesity, behavioral difficulties, hypogonadism, short stature |
Angelman syndrome; 15q11.2 maternal deletion | 1/12,000 | ID/DD, severe speech impairment, gait ataxia, inappropriate happy affect, microcephaly, seizures |
17q12 deletion | 1/14,500 | Kidney/urinary abnormalities, diabetes, ID/DD, ASD, psychiatric conditions |
Sotos syndrome; 5q35 deletion | 1/15,000 | ID/DD, overgrowth, characteristic facies |
Smith–Magenis syndrome; 17p11.2 deletion | 1/15,000–1/25,000 | ID/DD, characteristic facies, sleep disturbances, behavioral issues including self-injury and self-hugging and aggression, characteristic facies, reduced sensitivity to pain and temperature |
Cri-du-chat; 5p15 deletion | 1/15,000–1/50,000 | High-pitched cry, microcephaly, hypotonia, characteristic facies, ID/DD, CHD |
Koolen de Vries; 17q21 deletion | 1/16,000 | ID/DD, sociable personality, hypotonia, seizures, distinct facial features, CHD, kidney anomalies, foot deformities |
Potocki–Lupski syndrome; 17p11.2 duplication | 1/20,000 | ID/DD, ASD, hypotonia, CHD |
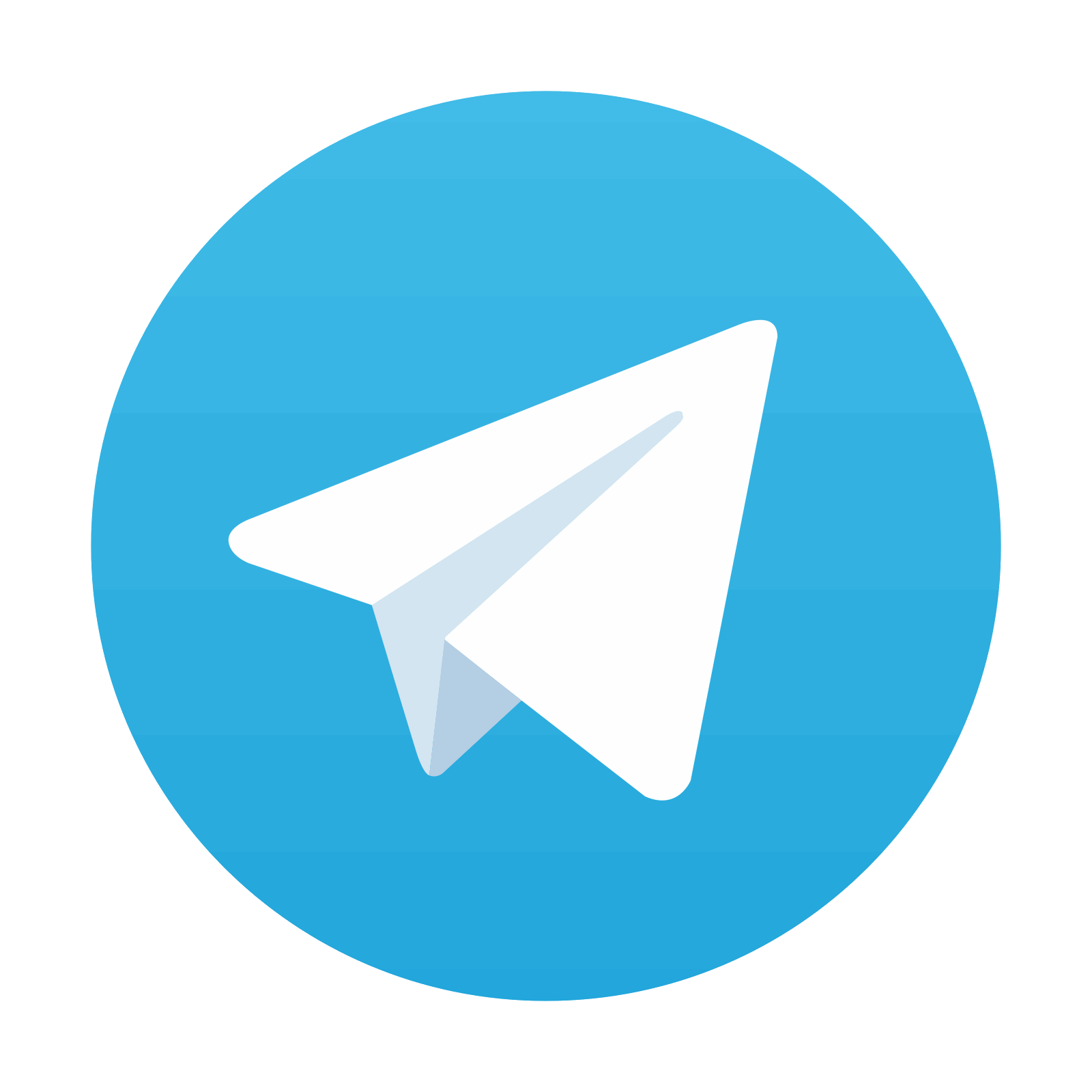
Stay updated, free articles. Join our Telegram channel

Full access? Get Clinical Tree
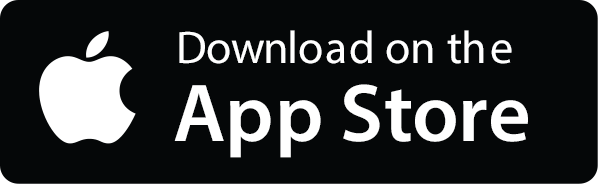
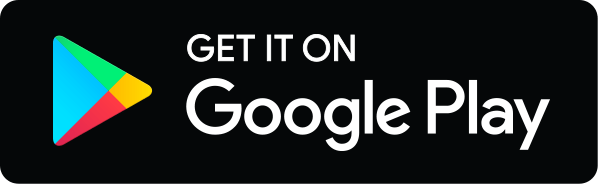
