Prenatal Diagnosis and Consultation
THE INCREASINGLY COMPLEX LANDSCAPE OF PRENATAL DIAGNOSIS AND CONSULTATION
Advances in prenatal diagnosis, imaging, and prenatal maternal-fetal and postnatal neonatal care have led to dramatically expanded opportunities and challenges for fetal management and prenatal counseling. Over the recent decades, medical and surgical treatment options for newborns with even the most complex congenital anomalies have substantially increased. Concurrently, prenatal screening and diagnostic capabilities have expanded, leading to early diagnosis of fetal problems. Maternal and family anxiety have been shown to be significantly reduced after antenatal consultation by an experienced, specialized staff.1,2 Nevertheless, depending on the specific fetal finding, the physician and family may still be left with significant uncertainty regarding outcomes. If the pregnancy is continued, management strategies for the expectant mother and fetus range from those narrowly focused on maternal or fetal well-being to pursuing prenatal interventions, which may now be offered for some fetal indications. How these strategies are presented, and by whom, may strongly affect an expectant mother’s decisions.3,4 Furthermore, incomplete communication and collaboration among medical and surgical subspecialists in complex fetal cases may lead to incorrect information dissemination, confused message framing for the family, and a muddied picture of maternal, fetal, and neonatal risk-benefit and outcomes.5 Finally, learning that an eagerly awaited child may be born with congenital anomalies can be the most emotionally stressful and difficult period in the life of an expectant mother or father; for some, the experience constitutes a true psychiatric trauma.6 Therefore, experienced personnel in support services, including medical social services, palliative care, and biomedical ethics, must be available for expectant mothers and families in these circumstances.
For all of these reasons, a multidisciplinary approach to complex fetal diagnosis, management, intervention, and family consultation is critical to presenting as balanced and accurate a view as possible and to protect the interests of both mother and fetus.7 The expertise and input of each member is crucial to inform plans for the pregnancy management, delivery mode and venue, and immediate postdelivery neonatal requirements. The team composition in a multidisciplinary fetal center may vary depending on the cases evaluated and managed at a particular institution. Genetics, maternal-fetal medicine, fetal and pediatric diagnostic imaging, and neonatology expertise, as well as a range of medical and surgical subspecialists and social service support, are important for building a strong foundation in complex fetal care and prenatal counseling.
This chapter is too brief to provide a comprehensive presentation of all aspects of prenatal diagnosis and counseling. We therefore focus on fundamental principles of three areas: genetic prenatal screening and diagnosis; imaging of fetal anomalies; and the benefits of a prenatal consultation by a neonatologist in complex fetal cases.
GENETIC PRENATAL SCREENING AND DIAGNOSIS
Over the last quarter century, prenatal genetic screening and diagnosis have become mainstays of the medical care of women who are pregnant or contemplating pregnancy. Maternal serum α-fetoprotein (AFP) measurement and fetal ultrasound examination make it possible to identify neural tube defects (NTDs) in up to 95% of affected pregnancies.8 First- and second-trimester screening for aneuploidies such as trisomy 21 and 18 detect 65%–90% of these disorders.9 Improvements in the acceptability, availability, and reliability of such testing have resulted in substantial changes in obstetric and pediatric practice. New technology has improved the sensitivity and positive predictive value of screening tests, as well as the safety and accuracy of tests used for prenatal diagnosis. These tests have the potential to be highly beneficial for prospective parents, and pediatricians and others caring for children need to be aware of the significance of the results.
Screening for Neural Tube Defects
Neural tube defects, including spina bifida and anencephaly, represent some of the most common birth defects in humans, occurring in 1–2/1000 pregnancies. When isolated, the etiology is considered to be multifactorial, involving a combination of genetic predisposition and environmental influences. One such environmental influence is folic acid, which prevents both occurrence and recurrence of NTDs.
In 1977, Wald et al reported on the efficacy of measuring the concentration of AFP in the serum of pregnant women to screen for the presence of NTDs.10 Maternal serum AFP alone detects 75%–90% of open NTDs and more than 95% of anencephaly, with a false-positive rate of 5% or less. Currently, maternal serum AFP testing is offered to all pregnant women between 15 and 20 weeks gestation. When ultrasound examination is performed in conjunction with maternal serum AFP measurement, the detection rate is further improved, although some pregnancies require evaluation of amniotic fluid AFP and acetylcholinesterase levels for confirmation.8
Elevated maternal serum AFP is associated with other conditions, including abdominal wall defects such as gastroschisis and omphalocele,11 supporting the role of ultrasound as a first-line evaluation in screen-positive cases. Adverse pregnancy outcomes, including fetal loss, preterm delivery, intrauterine growth restriction, and placental abruption are also associated with elevated maternal serum AFP.12
First-Trimester Screening for Aneuploidy
First-trimester screening uses a combination of maternal serum analytes and ultrasound measurement of fetal nuchal translucency (NT) to provide an age-adjusted risk for trisomy 21 and 18. The maternal serum markers pregnancy-associated plasma protein A (PAPP-A) and human chorionic gonadotrophin (hCG) are measured at 9 to 14 weeks gestational age, and NT is measured at 11 to 14 weeks gestational age. Using such a protocol, about 80%–90% of Down syndrome pregnancies are detected at a 5% screen-positive rate.9,13,14 The detection rate for trisomy 18 is slightly lower. PAPP-A levels are decreased and hCG levels are increased in trisomy 21; levels of both analytes are decreased in trisomy 18.
Nuchal translucency is present in virtually all fetuses and consists of a space of varying size behind the fetal neck. The size of the NT is correlated with fetal size as measured by crown-rump length. NT alone detects up to 70% of trisomy 21 pregnancies and a similar proportion of those with other chromosomal abnormalities, such as trisomy 13, trisomy 18, and 45,X,15 but is not used as a stand-alone test. Some researchers, however, suggest that an NT of 4.0 mm or greater should serve as an indication for invasive diagnostic testing because the use of serum markers offers little in the way of further risk adjustment for trisomy 21.16,17 In addition to its utility in detecting aneuploidy, increased NT size is associated with a wide range of other fetal abnormalities, such as cardiac defects, diaphragmatic hernia, and a number of single-gene disorders, including skeletal dysplasias.18,19 In general, the risk for an adverse outcome is positively correlated with the size of the NT. The American College of Obstetricians and Gynecologists (ACOG) recommends that in pregnancies with a NT measurement of 3.5 mm or greater, fetal echocardiography and targeted ultrasound examination should be offered for detection of specific malformations or malformation syndromes.20
Second-Trimester Screening for Aneuploidy
Multiple maternal serum markers are used to assess the risk for trisomy 21 and trisomy 18 in the second trimester, usually at about 15–20 weeks gestational age. The most effective strategy uses maternal serum AFP, hCG, unconjugated estriol (uE3), and inhibin A. Often called the “quad screen,” this test detects about 75% of trisomy 21 pregnancies at a 5% screen-positive rate.9 Typically, the AFP and uE3 levels are lower and the hCG and inhibin A levels are higher in trisomy 21 pregnancies. The detection rate for trisomy 18 is slightly lower. In most screening programs, the quad screen has replaced the triple screen, which uses only AFP, hCG, and uE3. The quad screen detects about 10% more cases of trisomy 21 than the triple screen, with a similar screen-positive rate. Second-trimester screening does not generally detect other aneuploidies, such as trisomy 13, Klinefelter syndrome (47,XXY), or Turner syndrome (45,X).
Combined First-Trimester and Second-Trimester Screening for Aneuploidy
Protocols for use of varying combinations of first-trimester and second-trimester screening have been investigated. Integrated screening provides a single adjusted risk estimate after all testing is completed and detects about 95% of trisomy 21 fetuses.9 This method requires that first-trimester results be withheld until completion of second-trimester screening. To mitigate this problem, 2 sequential screening strategies have been developed to provide first-trimester risk information. Stepwise screening discloses results of first-trimester screening for high-risk pregnancies and defers those at lower risk for final risk assessment after the second-trimester results have been received.21 Contingency screening uses first-trimester screening results to offer invasive testing for women at high risk, to defer risk assessment until completion of second-trimester screening for women at intermediate risk, and to suggest no further testing for women at low risk.22 Each of these strategies detects about 90% of trisomy 21 fetuses, but their complexity makes them more difficult to implement than either first- or second-trimester screening alone.23
Fetal Renal Pelvis Dilation
As described in the next section, prenatal ultrasound may identify fetal markers of unknown clinical significance such as renal pelvis dilation (RPD) or short femurs. Communication with the neonatologist or pediatrician is important, as he or she will need to be aware of the implications of the prenatal findings, perform newborn and follow-up examinations, and decide if postnatal evaluation is warranted. Although most cases of RPD either resolve or remain stable and require no active treatment,24 when the RPD is greater than 15 mm, the risk of serious renal disease is high. There is no consensus on how best to evaluate and treat those with mild or moderate dilation. Many follow-up protocols recommend that a single postnatal ultrasound examination demonstrating RPD less than 15 mm does not require follow-up studies, although in practice it appears common to perform at least one additional ultrasound examination to confirm resolution.25 These and other authors have questioned the need for invasive studies such as voiding cystourethrogram (VCUG) in this group of patients.26
Prenatal Diagnosis by Amniocentesis
Amniocentesis for diagnosis of chromosome abnormalities has been performed since the 1960s and is usually carried out between 15 and 18 weeks gestation. Using ultrasound guidance, amniocentesis has a procedure-related pregnancy loss rate of about 1 in 300 to 600.27,28 In the recent past, amniocentesis was offered only to women who had an increased risk for fetal aneuploidy based on abnormal screening results or to women more than 35 years of age based on the rationale that the risk for having a child with a chromosomal abnormality approached the procedure-related pregnancy loss rate. However, current guidelines from the American College of Medical Genetics (ACMG) and ACOG call for amniocentesis or chorionic villus sampling (CVS) to be made available to all pregnant women, after appropriate counseling regarding its risks and benefits, and that maternal age should no longer be used as a threshold to determine who is offered invasive testing.27,29 In addition to diagnosis of fetal aneuploidy, amniocentesis is also a useful procedure for diagnosis of many single-gene disorders, either by mutation analysis of cultured amniocytes or through biochemical analysis of analytes diagnostic of inborn errors of metabolism. Amniocentesis may also be performed to measure amniotic fluid AFP levels and acetylcholinesterase for confirmation of open NTDs.
Prenatal Diagnosis by Chorionic Villus Sampling
Chorionic villus sampling gained acceptance in the United States in the 1980s and 1990s as an alternative to amniocentesis and is usually performed from 10 to 13 weeks gestation. The pregnancy loss rate after CVS is greater than the rate for midtrimester amniocentesis, but much of this increase may be related to an increased background loss rate between 10 and 16 weeks gestation.20 The major advantage of CVS over amniocentesis is that it can be performed earlier in pregnancy and provide earlier reassurance, earlier knowledge of a fetal abnormality and a longer period of preparation for care and management of an affected infant, or an opportunity for an earlier pregnancy termination, which may be associated with fewer maternal complications. Like amniocentesis, CVS is also a useful procedure for obtaining fetal tissue that can be used for mutation analysis for diagnosis of a known genetic disorder. Because amniotic fluid is not collected by CVS, measurement of analytes that can diagnose inborn errors of metabolism or confirm the diagnosis of open NTDs is not possible. Therefore, maternal serum AFP screening between 15 and 20 weeks gestation is still recommended. Also, because fetal anatomy is not well visualized at 10–12 weeks gestation, a high-resolution ultrasound examination is recommended between 16 and 18 weeks gestation.
Chromosome Analysis: Cytogenetic analysis versus Array-Based Comparative Genomic Hybridization
Conventional cytogenetic analysis is used to detect fetal chromosome abnormalities after amniocentesis or CVS. In pediatric practice, array-based comparative genomic hybridization (aCGH), also known as chromosomal microarray (CMA), is now recommended as the first-tier clinical diagnostic test for individuals with developmental disabilities or congenital anomalies.30 The utility of aCGH for prenatal diagnosis has been investigated in several large case series and found to have some benefits over conventional karyotyping,31,32 but concerns about its high cost, its inability to detect unbalanced chromosome rearrangements, and its detection of copy number variants of unknown clinical significance has led the ACOG to recommend against its routine use in prenatal diagnosis at present.33
Cell-Free Fetal DNA in Maternal Plasma
Approximately 5% of cell-free DNA in maternal blood is of fetal origin, and massively parallel shotgun sequencing (MPSS) has been used to detect trisomy 21 prenatally. One recent study of high-risk pregnant women identified Down syndrome with a 98.6% detection rate, a 0.2% false-positive rate, and a 0.8% false-negative rate.34 This technology shows promise in improving screening for trisomy 21 as well as other aneuploidies; however, validation studies in non-high-risk populations is necessary before this technique will be considered standard of care.
Imaging of Fetal Anomalies
Fetal imaging with high-resolution ultrasound and rapid acquisition magnetic resonance imaging (MRI) is central to accurate diagnosis of congenital anomalies. Imaging has enhanced our understanding of the natural history of many prenatally diagnosed fetal anomalies, allowed for improved prediction of outcome and counseling and for pregnancy planning and newborn management. Communication of the prenatal findings to the postnatal caregivers is critical to ensure that the newborn is optimally managed, with appropriate postnatal imaging studies and correct treatment protocols.35
Ultrasound is the primary imaging modality for the diagnosis of most fetal anomalies. MRI can provide incremental information compared with ultrasound by defining lesion extent or detecting additional abnormalities. Studies have shown that ultrasound and MRI can be powerful, effecting a change or adding a diagnosis in 57% of cases.36 MRI has advantages over ultrasound when the position of the fetal head is low in the pelvis or is difficult to see because large maternal body habitus, oligohydramnios, or the presence of another fetus precludes detailed examination (Figure 7-1). Furthermore, MRI provides a large field of view in three spatial planes—axial, sagittal, and coronal—which is helpful for understanding anatomic relationships of larger anomalies (Figure 7-2). A thorough discussion of fetal anomaly imaging is beyond the scope of this chapter. We discuss the role of imaging for fetal anomalies, focusing on chest and central nervous system (CNS) findings.
FIGURE 7-1 Expectant mother carrying fetus at 23 weeks gestational age. A, Axial plane sonogram through the fetal abdomen shows severe image degradation secondary to maternal body habitus (asterisks = subcutaneous fat; arrows = fetal abdomen). B, Axial magnetic resonance imaging (MRI) through the maternal pelvis shows a large amount of maternal subcutaneous fat (asterisks = fat; arrows = fetus). C, Coronal plane MRI of the fetus shows no significant image degradation despite maternal obesity (arrows = fetal brain; black asterisks = fetal lungs; arrowheads = fetal kidneys; white asterisks = placenta; circle = amniotic fluid).
FIGURE 7-2 Lymphatic malformation involving fetal neck and chest. A, Axial sonogram through the lower fetal neck shows a large multicystic mass (arrows = lymphatic malformation; arrowheads = fetal spine). B, Coronal plane magnetic resonance imaging (MRI) depicts anatomic relationship of the lymphatic malformation (large arrows) to the fetal neck, chest, and airway (arrowheads) (asterisks = fetal lungs; S, stomach; B, bladder). Fetal neck mass deviates fetal neck to the left, but there is no evidence for airway compromise.
Fetal Brain Anomalies
The majority of fetal brain MRIs are performed to further evaluate ventriculomegaly, a posterior fossa cyst, or absence of the cavum septum pellucidum (CSP) diagnosed on ultrasound. Associated diseases identified with MRI and not detected with ultrasound are for the most part abnormalities of the cerebral/cerebellar parenchyma, such as neuronal migration disorders, delayed sulcation and gyration, small periventricular cysts, and intraparenchymal hemorrhagic foci.37,86 (Figure 7-3). In a study of 145 fetuses with fetal central nervous system abnormalities diagnosed on ultrasound, Levine et al noted that MRI led to a change in diagnosis in 32%, a change in counseling in 50%, and a change in management in 19% of cases.
FIGURE 7-3 Cortical heterotopia. A, Axial sonogram demonstrates mild dilation of the lateral ventricle (calipers = 12 mm). B, Axial plane magnetic resonance imaging (MRI) confirms mild ventriculomegaly (asterisks) and subependymal nodularity (arrows), consistent with cortical heterotopia.
Fetal ventriculomegaly is a relatively common abnormality identified in approximately 1/1000 births and often represents a counseling dilemma for predicting outcome.38 Ventriculomegaly is a nonspecific dilation of the lateral ventricles, which can result from many different types of brain abnormalities or insults. Prognosis for ventriculomegaly depends on the severity and the presence or absence of associated cerebral or extracerebral anomalies. Ventriculomegaly is usually categorized as mild (atrial diameter 11–15 mm) or severe (atrial diameter > 15 mm). The most guarded outcomes occur in cases of ventriculomegaly associated with abnormal karyotype or additional anomalies by fetal imaging.38,39 Several studies have reported an increased detection rate by MRI compared with ultrasound for both CNS and non-CNS anomalies associated with ventriculomegaly.40 However in a subset of isolated mild ventriculomegaly (10-12 mm), MRI provided additional findings to ultrasound in 19.5% of cases, and changed the clinical prognosis in 1.1% of cases.40
Beeghly et al reported only 60% live delivery and survival through the neonatal period in fetuses with ventriculomegaly associated with other CNS findings.39 Approximately 60% of ventriculomegaly cases are classified as isolated on ultrasound and present a counseling dilemma because of heterogeneous outcome. Up to 23% of isolated mild ventriculomegaly cases are reported to have an abnormal outcome because of associated cerebral malformations, infection, or aneuploidy.41 When a fetal patient has isolated mild ventriculomegaly diagnosed on ultrasound and MRI shows no additional cerebral or extracerebral malformations, the prognosis is very good; greater than 90% have been reported to have a normal outcome (Figure 7-4).38 Mild asymmetrical ventriculomegaly diagnosed on ultrasound and confirmed on MRI has also been reported to be associated with neurodevelopmental delay.42,43
FIGURE 7-4 Mild ventriculomegaly on ultrasound with normal postnatal outcome (20 weeks gestational age). A, Axial ultrasound demonstrates mild ventriculomegaly (calipers = 12 mm). Brain parenchyma (asterisks), particularly in the near field, was difficult to visualize. B, Axial magnetic resonance imaging (MRI) confirms mild ventriculomegaly (asterisks) but otherwise normal brain parenchyma, including the frontal horns (arrowheads), anterior corpus callosum (long arrow), and brain mantle (short arrows).
Sonographic diagnosis of a posterior fossa cyst raises concern for the Dandy Walker spectrum: mega cisterna magna, inferior vermian dysgenesis, and Dandy Walker malformation. Fetal MRI can differentiate between these entities and search for additional associated brain or extracerebral anomalies. The distinction is important because isolated mega cisterna magna is associated with an excellent clinical outcome, whereas inferior vermian dysgenesis and Dandy Walker malformation may have more guarded outcomes (Figures 7-5 and 7-6).44,45 The vermis undergoes rapid development prior to 22 weeks gestational age, and the inferior vermis in particular may lag in development, which may lead to overdiagnosis of vermian hypoplasia on MRI.
FIGURE 7-5 Mega cisterna magna (29 weeks gestational age) with normal postnatal outcome. A, Axial sonogram shows enlargement of the cisterna magna (calipers = 12 mm). B, Sagittal plane magnetic resonance imaging (MRI) confirms normal cerebellar vermis (arrows) and brain stem (arrowheads) (asterisk = enlarged cisterna magna).
FIGURE 7-6 Inferior vermian dysgenesis (25 weeks gestational age). A, Axial sonogram demonstrates 12-mm posterior fossa cyst (arrowheads) (short arrows = cerebellar vermis). B, Vermian hypoplasia. Sagittal plane magnetic resonance imaging (MRI) confirms normal superior vermis (arrow) but under development of the inferior vermis (asterisk = cisterna magna).
The CSP is a normal midline structure that separates the anterior horns of the lateral ventricles, and its presence has a high correlation with normal midline brain formation. The CPS should be identified on all second- and third-trimester fetal ultrasound examinations. Absence or abnormal configuration of the CSP is associated with agenesis of the corpus callosum, holoprosencephaly, and septo-optic dysplasia (SOD). The corpus callosum represents the fiber tracks that connect the two hemispheres of the brain. The corpus callosum may be partially or completely absent (agenesis), which can occur as an isolated finding or in combination with other cerebral abnormalities. Partial or complete agenesis of the corpus callosum can be diagnosed by ultrasound by demonstrating separation of the frontal horns of the lateral ventricles on a coronal view of the brain or colpocephaly, often associated with a teardrop configuration to the posterior lateral ventricles. MRI is useful in confirming the diagnosis in equivocal cases on ultrasound and in evaluating for associated anomalies (Figure 7-7). Absence or partial agenesis to corpus callosum has been reported as an incidental or isolated finding, which can have a normal neurologic outcome; however, associated abnormal CNS or extracerebral findings are associated with substantially increased risk for neurodevelopmental impairment.46
FIGURE 7-7 Agenesis of the corpus callosum (22 weeks gestational age). A, Axial sonogram shows borderline ventriculomegaly (calipers = 10 mm). B, Axial plane magnetic resonance imaging (MRI) demonstrates colpocephaly (arrows) (dilation of the posterior body of the lateral ventricles) and parallel orientation of the ventricles. C, Coronal plane MRI through the region of the frontal horns shows absence of the anterior corpus callosum and a prominent interhemispheric fissure (asterisk) extending inferiorly (short arrows = frontal horns).
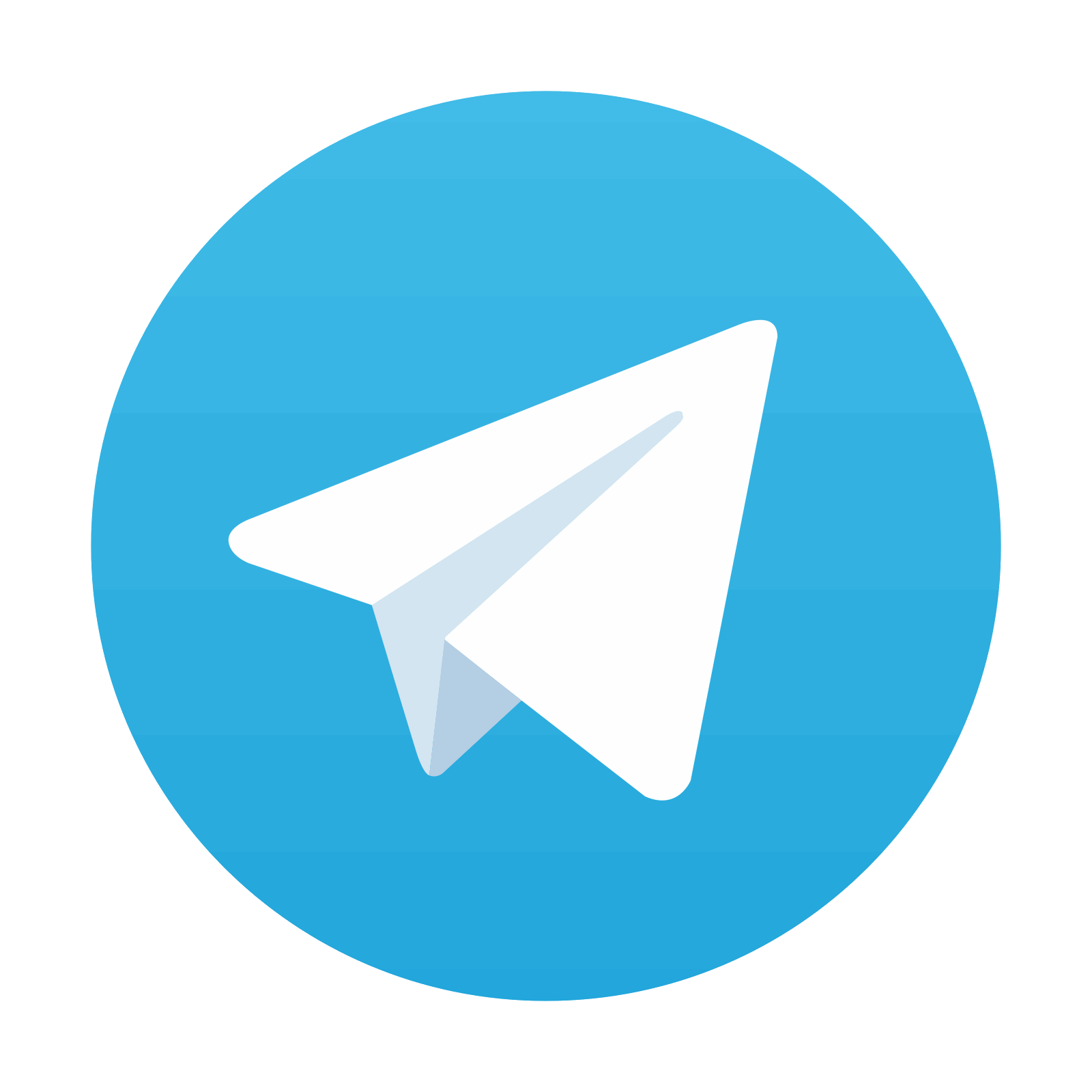
Stay updated, free articles. Join our Telegram channel

Full access? Get Clinical Tree
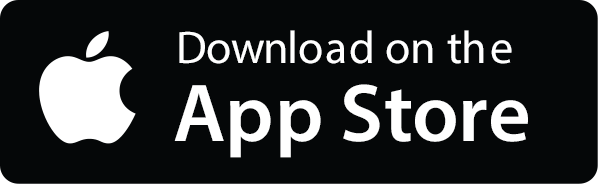
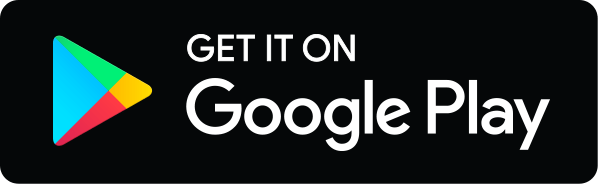