Objective
The role of posttranscription regulation in preeclampsia is largely unknown. We investigated preeclampsia-related placental microRNA (miRNA) expression using microarray and confirmatory quantitative real-time polymerase chain reaction experiments.
Study Design
Placental expressions of characterized and novel miRNAs (1295 probes) were measured in samples collected from 20 preeclampsia cases and 20 controls. Differential expression was evaluated using Student t test and fold change analyses. In pathway analysis, we examined functions/functional relationships of targets of differentially expressed miRNAs.
Results
Eight miRNAs were differentially expressed (1 up-regulated and 7 down-regulated) among preeclampsia cases compared with controls. These included previously identified candidates (miR-210, miR-1, and a miRNA in the 14q32.31 cluster region) and others that are novel (miR-584 and miR-34c-5p). These miRNAs target genes that participate in organ/system development (cardiovascular and reproductive system), immunologic dysfunction, cell adhesion, cell cycle, and signaling.
Conclusion
Expression of miRNAs that target genes in diverse pathophysiological processes is altered in the setting of preeclampsia.
Preeclampsia, a vascular disorder characterized by hypertension and proteinuria during pregnancy, is a consequence of diverse pathophysiological processes involving impaired implantation, endothelial dysfunction, and systemic inflammation. The placenta, a source and site of action of mediators of these processes, plays a central role in preeclampsia pathogenesis. Placenta-based investigations can potentially enhance our understanding of disease initiation and progression. While substantial evidence supports differential placental gene expression in preeclampsia, the role of related placental posttranscription regulation that may contribute to pathway/network perturbations is largely unknown.
MicroRNAs (miRNAs), small noncoding RNAs, are highly conserved posttranscription regulators of gene expression. Through inhibition of protein translation or promotion of messenger RNA (mRNA) degradation, miRNAs have roles in physiological and pathological processes such as cell differentiation, proliferation/growth, apoptosis, angiogenesis, inflammation, redox signaling, and other endothelial cell functions. Since these processes are disrupted in preeclampsia, miRNAs can potentially play significant roles in preeclampsia pathogenesis. Few investigators have studied placental miRNA expression in relation to preeclampsia and even fewer describe microarray-based global placental miRNA profiling in preeclampsia. To address this paucity of data, we investigated global placental miRNA expression using microarray and confirmatory quantitative real-time (qRT)-polymerase chain reaction (PCR) methodologies among 20 preeclampsia cases and 20 normotensive control subjects. In pathway analysis, we also examined functions and functional relationships of targets of differentially expressed miRNAs.
Materials and Methods
Study population and data collection
Study subjects were selected from participants of the Omega study (a cohort study) and the Placenta MicroArray study (a case-control study). Study populations and data collection procedures, described before, were briefly as follows. Omega study participants were women who initiated prenatal care <16 weeks of gestation and attended prenatal care clinics affiliated with Swedish Medical Center, Seattle, WA. The Placenta MicroArray study participants comprised women who delivered at Swedish Medical Center. Preeclampsia was diagnosed when both pregnancy-induced hypertension and proteinuria were present according to American College of Obstetricians and Gynecologists 2000 guidelines. Pregnancy-induced hypertension was defined as a sustained (≥2 measures 6 hours apart) blood pressure elevation (>140/90 mm Hg) >20 weeks of gestation. Proteinuria was defined as a sustained (≥2 measures 4 hours apart) presence of elevated protein in the urine (>30 mg/dL or >1+ on a urine dipstick). Controls were selected from those women who had normotensive pregnancies uncomplicated by proteinuria. Women who had history of chronic hypertension and/or pregestational diabetes as well as current nonsingleton pregnancies were excluded. Cases (N = 20) and controls (N = 20) were frequency matched for parity, maternal race/ethnicity, and labor status. Medical records were used to obtain information on risk factors, pregnancy history, and perinatal outcome. The institutional review board of the Swedish Medical Center approved study protocols. All participants provided written informed consent.
Placental sample collection
Placental tissue was collected immediately after delivery, weighed, double bagged, and transported in coolers to the placenta-processing laboratory where it was processed within 15 minutes postdelivery. The chorionic plate and overlying membranes were removed and tissue biopsies (∼0.5 cm 3 each) were obtained from 16 sites (8 maternal and 8 fetal sides) using a systematic sampling technique to achieve uniformity and adequate sampling. Briefly, the placenta was laid flat with the fetal side facing up and mapped into quadrants. Two samples were obtained from each quadrant; 1 medial (about 2 cm from the center) and 1 lateral (about 2 cm from the margin). The placenta was then turned over and 8 corresponding samples were taken from the maternal side. For this analysis, biopsy samples taken from the maternal side consisting primarily of the villous tissue, uteroplacental arteries, and some decidua basalis were evaluated. Biopsy samples were placed in cryotubes containing RNAlater (Qiagen Inc, Valencia, CA) at 10 μL per 1 mg of tissue and stored at –80°C.
RNA extraction and quality control
Total RNA was extracted from samples using a modification of the acid guanidinium thiocyanate-phenol chloroform extraction method. Briefly, up to 30 mg of tissue was homogenized in 1000 μL of RNASTAT60 (Tel-Test, Friendswood, TX), followed by addition of 250 μL of chloroform and vortexing for 1 minute. The RNA was precipitated with 2× volumes of isopropanol overnight at –20°C before being pelleted with a 30-minute centrifugation at 12,000 g . The RNA pellets were resuspended in RNAsecure (Ambion, Foster City, CA). Up to 10 μg of RNA for each sample was treated with Turbo DNase (Ambion) for 1 hour at 37°C to remove any residual genomic DNA. All samples were evaluated using ultraviolet spectrophotometry and gel electrophoresis. Total RNA concentration was calculated by determining absorbance at 260 nm (Spectramax Plus 384 spectrophotometer; Molecular Devices Corp, Sunnyvale, CA) in 10 mmol/L Tris-HCl. All samples had A260/A280 ratio >2.0. Low molecular–weight (LMW) RNA (∼0-200 nucleotides) was purified from total RNA by size fractionation on YM-100 ultrafiltration columns (Millipore Filter Corp, Bedford, MA) and further purified on RNeasy MinElute columns (Qiagen Inc) using a small RNA isolation protocol. The LMW RNA samples were 3′-end-labeled with Alexa-647 fluorescent dye using the Rapid Labeling Kit (Invitrogen, Carlsbad, CA). All RNA samples underwent a quality-control check, and were labeled using the same standardized protocols.
Global miRNA expression profiling
Global miRNA profiling was conducted using custom microarrays at Oceanridge Biosciences (Palm Beach Gardens, FL). The microarray, manufactured by Microarrays Inc (Huntsville, AL), consisted of epoxide glass substrates spotted in triplicate with each probe. They contain a total of 1295 probes including 854 probes against human mature miRNA sequences from Sanger 12.0 mirBASE, 379 probes against novel small RNAs from the Invitrogen Ncode Version 3.0 probe set, and 59 control probes. Labeled LMW RNA samples were hybridized to the miRNA microarrays according to the Rapid Labeling Kit manual (Invitrogen). Microarrays were scanned using an Axon Genepix 4000B scanner (Molecular Devices Corp), and data were extracted using GenePix V4.1 software (Molecular Devices Corp).
Data preprocessing
Spot intensities were obtained for the 3906 features on each microarray by subtracting the median local background from the median local foreground for each spot. Detection thresholds for each array were determined by calculating the mean intensity of the negative control spots and adding 5× the SD of the background (nonspot area). The spot intensities and the threshold (T) were transformed by taking the log (base 2) of each value. The normalization factor (N) for each microarray was determined by obtaining the average spot intensity for novel small RNAs probes from Invitrogen (IVGN-Novel). The log2-transformed spot intensities for all features were normalized by subtracting N from each spot intensity, and scaled by adding the grand mean of N across all microarrays. The mean probe intensities for each probe on each of the 40 arrays were then determined by averaging the triplicate spot intensities. Spots flagged as poor quality during data extraction were omitted prior to averaging.
Confirmatory qRT-PCR experiment
A confirmatory qRT-PCR experiment to validate microarray-based measurements was conducted for selected miRNAs. The selection, limited by availability of primers, was based on microarray study findings and previous reports of potential significance in preeclampsia or related pathophysiologic processes. LMW RNA was reverse-transcribed using miRNA-specific primers (Applied Biosystems, Foster City, CA). The complementary DNA was amplified by qRT-PCR using universal Taqman mix and miRNA-specific primers according to the manufacturer’s protocol. Reactions were run on an ABI Step1 Plus Real Time PCR machine (Applied Biosystems) using the default cycling conditions. All reactions were analyzed by using the 2 −ΔΔ C T calculation procedure. For normalization, we used Δ CT values calculated by subtraction of cycle threshold (CT) values for expression of the hsa-miRNA-525-5p, a housekeeping miRNA.
Statistical analysis
Analysis was conducted on normalized and log2-transformed data for the set of probes showing signal above T in at least 10% of the samples. Differences between cases and controls were evaluated by 1-way analysis of variance using National Institute of Ageing (NIA, Bethesda, MD) Array Analysis software. An exploratory principal component analysis (PCA) was performed using the subroutine built in to NIA Array Analysis software. The purpose of the PCA was to reduce multidimensionality of the expression data and identify potential outliers. Absolute fold change >1.5 and P value < .05 were used to identify differentially expressed miRNA(s). In addition, we recalculated P values of differentially expressed miRNAs using the ranking-based Benjamini and Hochberg false discovery rate correction method. Data for the human noncontrol probes were clustered using Gene Cluster 3.0 software (Stanford University, Palo Alto, CA). In the hierarchical clustering, centered correlation was used as the similarity metric and average linkage as the clustering method. TargetScan ( www.targetscan.org ; Whitehead Institute of Biomedical Research, Massachusetts Institute of Technology, Boston, MA), a database that employs both conserved and nonconserved seed pairing algorithms, was used to identify putative miRNA targets of differentially expressed miRNA. Functions and functional relationships of genes that are targeted by ≥2 miRNAs were evaluated using Kyoto Encyclopedia of Genes and Genomes (KEGG) and Ingenuity Pathway Analysis (Ingenuity, Redwood, CA). To identify KEGG pathways enriched with gene targets, we performed the gene set analysis using World Wide Web–based Gene Set Analysis Toolkit (WebGestalt; Vanderbilt University, Nashville, TN). Statistical significance of pathways was determined on the basis of a P value < .05 and the presence of at least 2 target genes in the pathway. In Ingenuity Pathway Analysis, each gene identifier was mapped to its corresponding gene object in the Ingenuity Pathways Knowledge Base. These genes were overlaid onto a global molecular network developed from information contained in the Ingenuity Pathways Knowledge Base. Network enrichment was then assessed using a network score (negative log of P values of Fisher’s tests).
Finally, we investigated the correlation between microarray-based expression measurement fold change differences between cases and controls and similar differences based on the qRT-PCR expression measurements for selected miRNA. The correlation coefficient (R 2 ) of fold changes values was used as an indicator of overall consistency between the 2 measurements.
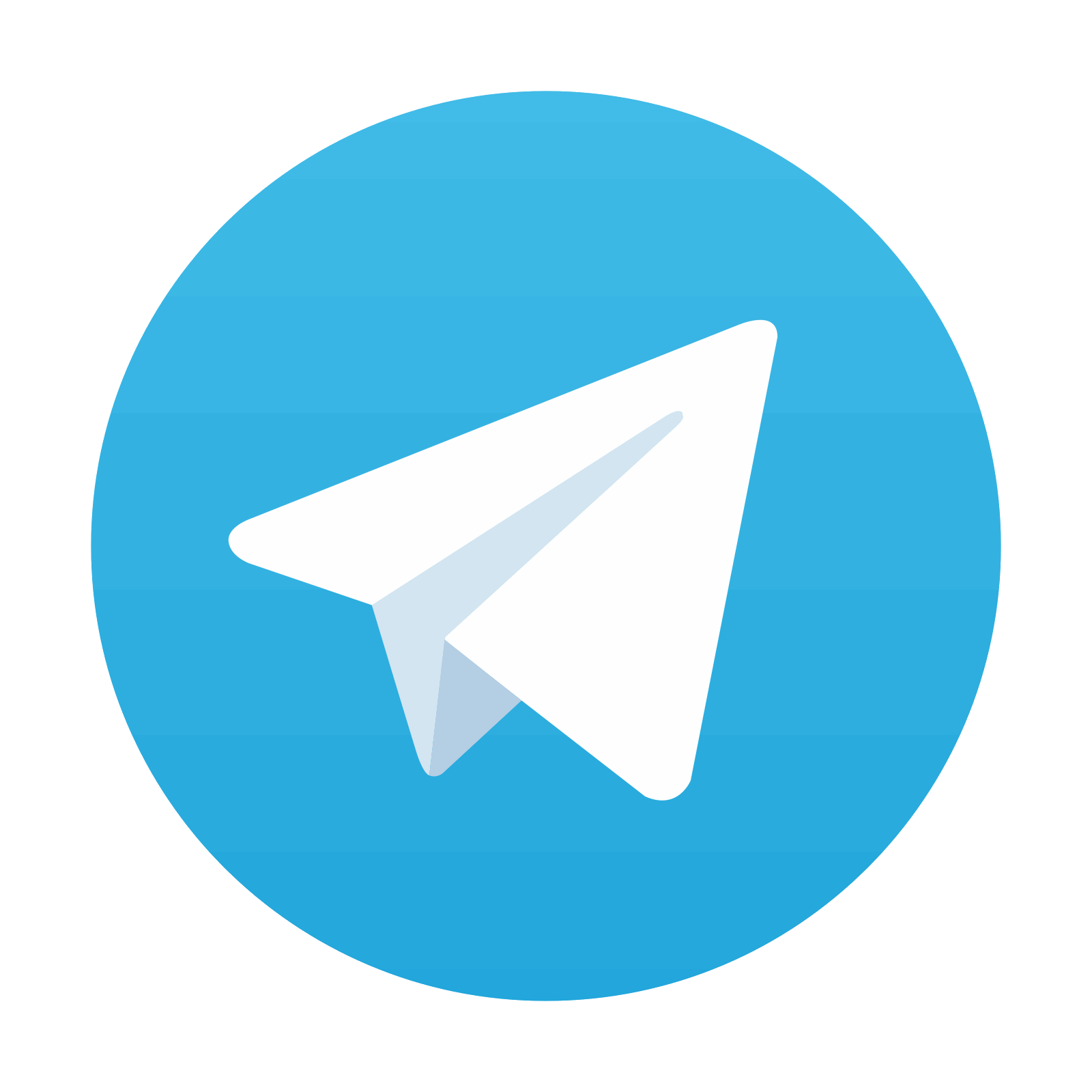
Stay updated, free articles. Join our Telegram channel

Full access? Get Clinical Tree
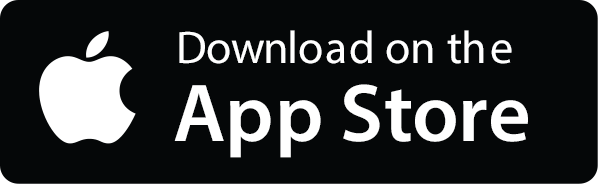
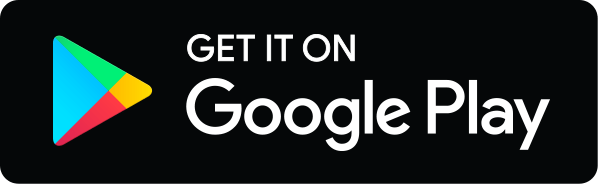