Prasad P. Godbole, Martin A. Koyle and Duncan T. Wilcox (eds.)Pediatric Endourology Techniques2nd ed. 201410.1007/978-1-4471-5394-8_4
© Springer-Verlag London 2014
4. Physiology of Minimally Invasive Surgery Versus Open Surgery
(1)
Department of Pediatric Urology, The Hospital for Sick Children, Toronto, ON, Canada
(2)
Department of Surgery, Division of Urology, The Hospital for Sick Children, Toronto, ON, Canada
Abstract
Endoscopic and minimally invasive surgery (MIS) in pediatric urology affords many benefits when compared to open surgery but also introduces unique physiologic considerations. The surgical team must be aware of the physiologic changes associated with these approaches in order to avoid making MIS detrimental. The knowledge presented in this chapter should bring the anesthetist and the MIS surgeon together to uphold the success of these procedures and ultimately improve the care of children in this setting.
Keywords
Minimally invasive surgeryLaparoscopyPediatric urologyPhysiologyPneumoperitoneumAnesthesiaAbbreviations
CBF
Cerebral blood flow
CO
Cardiac output
CO2
Carbon dioxide
ETCO2
End tidal CO2
GFR
Glomerular filtration rate
HR
Heart rate
IAP
Intra-abdominal pressure
ICP
Intracranial pressure
MAP
Mean arterial pressure
MIS
Minimally invasive surgery
PIP
Peak inspiratory pressure
SV
Stroke volume
SVR
Systemic vascular resistance
Introduction
Endoscopic and minimally invasive surgery (MIS) in urology, by both robotic and pure laparoscopic techniques, affords many benefits when compared to open surgery. These benefits include smaller incisions, less postoperative pain, and earlier oral intake, discharge home, and return to normal function [1–4]. Consequently, the applications of MIS are growing, particularly in the field of pediatric urology, where increasingly complex reconstructive procedures are being performed for smaller children.
While many benefits of MIS are proven, these technologies introduce unique physiologic considerations when compared to their traditional open counterparts. In pediatrics, these effects should not be directly extrapolated from the adult data, since children of varying sizes and ages manifest distinct physiologic responses [5]. For instance, compared to adults, children have baseline lower blood pressure and systemic vascular resistance (SVR), while heart rate (HR), oxygen consumption, and cardiac output (CO) are relatively higher [6]. Furthermore, neonates and infants have particularly unique physiologic factors, such as a rate-dependent cardiac output, a propensity to bradycardia, a shorter trachea, lower chest compliance, largely diaphragmatic respiration, and the possibility of persistent right to left cardiac shunts [7]. Hence, the effects of MIS in very young and small children may be even more pronounced [8, 9].
Broadly, the physiologic changes during MIS result from carbon dioxide (CO2) absorption during insufflation, increased intra-abdominal pressure (IAP), and special positioning requirements. While most of these effects can be controlled with minor modifications in anesthetic management, it is important for the surgeon to understand potential issues to help prevent and manage complications when they occur. In this chapter, we will review the physiologic changes during MIS in the context of pediatric urology. One must additionally consider the option of retroperitoneal insufflation and how its impact may differ from transperitoneal approaches, which will also be reviewed here. The effects of CO2 absorption, increased IAP, and positioning can either compound or mitigate each other significantly, thus it is vital to note that multiple complex and codependent factors interact to produce the effects described. For simplicity of organization, they are discussed individually below.
Physiologic Response to CO2 Absorption
The ideal insufflant is noncombustible, has limited absorption and physiologic effects, and rapid excretion when absorbed [5]. CO2 is currently the gas of choice for most MIS procedures since it is odorless and colorless, has a high solubility in blood, and is readily excreted by the lungs once absorbed [4]. The degree of CO2 absorption is a function of the insufflation pressure, the surface area exposed to gas, and more importantly, patient factors such as age and weight [10, 11]. CO2 absorption is greater in children than in adults and appears more pronounced in younger children [4, 12, 13].
Some studies have compared CO2 absorption during peritoneal and retroperitoneal approaches [14, 15]. General adult studies have suggested more CO2 absorption during retroperitoneal insufflation and a more prolonged effect after desufflation [16, 17]. These results have been mirrored in pediatric urology, where one prospective study demonstrated increased CO2 levels for approximately 10 min after desufflation of the retroperitoneum [18]. The most recent prospective study comparing the two approaches in children found that end tidal CO2 (ETCO2) increases more progressively and gradually during retroperitoneoscopy, unlike the more rapid increase and plateau effect that occurs during peritoneal insufflation [19]. This observation might be explained by the smaller absorptive surface of the retroperitoneum combined with the lack of barrier effect from the peritoneal lining [17, 19].
In healthy children, CO2 absorption is well tolerated by compensatory mechanisms and ventilatory adjustments [7, 11], but its excess or uncompensated absorption can lead to metabolic disturbances, such as a decrease in serum pH levels, as well as neurologic, respiratory, and cardiovascular effects. These are summarized which are summarized in the subsequent sections [5].
Neurologic
Hypercarbia causes hemodynamic changes in the brain, such as cerebral vasodilation, increased cerebral blood flow (CBF), and increased intracranial pressure (ICP) [5]. These effects are only partially reversed with anesthesia-induced hyperventilation [4]. When ETCO2 is kept relatively constant, CBF still increases, suggesting an additional neurohormonal factor [20]. Karsli et al. found that the middle cerebral artery blood flow velocity increased proportionally to the mean arterial pressure (MAP) and ETCO2 during the first 8 min of pneumoperitoneum, while HR remained the same [19]. After 20 min, ETCO2 continued to increase, whereas the middle cerebral artery blood flow velocity and MAP reached a plateau and then decreased progressively. Conversely, during retroperitoneoscopy, both CBF velocity and ETCO2 increase progressively throughout the procedure but show parallel decreases towards baseline within 5 min of desufflation. It therefore seems that CBF and ETCO2 increase more gradually during retroperitoneal insufflation, compared to the more rapid increase and plateau effect observed during pneumoperitoneum. It is hypothesized, again, that this is due to the smaller absorptive surface of the retroperitoneum and absence of the peritoneal barrier which induces the plateau effect eventually [19]. These changes may have no clinical sequelae except in long cases, where the anesthetist and the surgeon should be aware of differential absorption rates depending on the approach.
Respiratory
Absorbed CO2 imposes an increased load on the respiratory system also, where hypercarbia and respiratory acidosis can result. ETCO2 is often used as a surrogate measure of absorbed CO2. Increases in ETCO2 are noted up to 33 % over baseline values despite ventilator adjustments in one study examining both thoracoscopy and laparoscopy in neonates [4, 8]. To clear excess CO2 and maintain normocarbia, minute ventilation may need to be increased by up to 50–75 % along with intermittent positive pressure ventilation [7]. Studies in neonates found that an increase of 22.6–40 % in minute ventilation with positive airway pressure was needed to help restore normocarbia [8, 21]. Indeed, Bannister observed that 95 % of patients required at least one ventilator adjustment during surgery to restore ETCO2 to within 10 % of their baseline value [2].
During prolonged procedures, large amounts of CO2 are also buffered in the muscle and fat, which must be eliminated by the lungs postoperatively [7]. Respiratory acidosis can persist postoperatively in conditions of poor respiratory function or when respiratory drive remains suppressed [7]. Overall, however, when compared to traditional open surgery, the postoperative respiratory benefits of MIS, such as improved rates of extubation and shorter chest physiotherapy requirements, likely outweigh the physiologic changes observed during laparoscopy [3].
Cardiovascular
CO2 absorption independently influences cardiac function with its direct depressive effects on the myocardium and secondary effects mediated by the autonomic nervous system. In adults, catecholamine release helps maintain CO by increasing HR and stroke volume (SV), which counteracts the effects of increased IAP [5, 22]. Hypercarbia similarly counteracts some of the effects of increased IAP by decreasing SVR and potentiating tachyarrhythmias, unlike the possible bradycardia that can occur with insufflation [7, 22]. Overall, these changes are well tolerated, and the compensatory ability improves with increasing age and size of children.
Physiologic Response to Increased Intra-abdominal Pressure
Pneumoperitoneum is a critical component to MIS techniques. Generally, children have increased abdominal wall laxity compared to adults, and lower insufflation pressures are often required [23]; however, working space remains limited by virtue of their more compact anatomy. While pneumoperitoneum facilitates surgery by expanding the working cavity, it has several physiologic effects resulting directly from the increase in IAP.
Neurologic
Increased IAP may affect the perfusion dynamics of the brain by causing a prompt and sustained increase in intracranial pressure (ICP) due to decreased jugular venous return. This finding is enhanced by the vasodilatory effects that occur with CO2 absorption. Intracranial venous stasis causes decreased resorption and drainage of cerebrospinal fluid, thereby increasing ICP further. Overall, these effects are proportional to the degree of IAP [4].
Two animal studies have evaluated the effects of increased IAP on ICP [24, 25]. Josephs et al. found that at an IAP of 15 mmHg, ICP increased from 13 to 18.7 mmHg, independently of arterial pH and CO2 levels. Bloomfield et al. found that an IAP of 25 mmHg increased ICP from a mean of 7.6–21.4 mmHg. Cerebral perfusion pressure fell from 82 to 62 mmHg but was partially restored with volume expansion. The implications of these findings in normal children are unclear, but extra caution should be used in conditions of decreased intracranial compliance, such as head injuries and ventriculoperitoneal (VP) shunts.
In otherwise healthy children, VP shunts are not currently considered an absolute contraindication to pneumoperitoneum [4]. If there are presumed risks in this population, such as shunt malfunction, infection, retrograde flow, or pneumocephalus, then protective means that include clamping or exteriorizing the shunt, shielding the shunt in an endoscopic bag, or regular exsufflation with pumping of the reservoir may be implemented [26–29]. Uzzo et al. advocated invasive ICP monitoring techniques and intermittent drainage of cerebrospinal fluid in their description of two pediatric cases of laparoscopic bladder autoaugmentation [30]. However, the need for such techniques in healthy children with VP shunts has been questioned by others [31]. The largest series of 18 patients with VP shunts in the pediatric urology literature showed no increased clinical sequelae during major and reconstructive MIS procedures [31]. These authors suggest that the risk of invasive ICP monitoring and shunt manipulation might actually outweigh any presumed benefit and recommended routine anesthetic care as the gold standard for this population.
Data on the infection rates during laparoscopy in children are scarce. One of the largest reviews of various pediatric procedures showed no statistically significant difference in shunt infection rates when comparing open to MIS approaches [32]. With regard to the potential mechanical tubing malfunctions that may occur due to increased IAP, in vitro testing has shown that the one-way valve mechanism of the VP shunt can withstand simulated pressures of up to 80 mmHg without structural distortion [33]. More recently, testing of various VP shunt tubing mechanisms with simulated pneumoperitoneum showed no reflux during insufflation pressures of up to 25 mmHg when the tubing was filled with saline, which simulated the more realistic scenario of a functioning shunt flowing with CSF [34]. In addition to the descriptions above, a number of case reports and series have described successful laparoscopy in this population without complication, and MIS is generally considered safe and feasible with heightened awareness from the surgeon and anesthetist [26, 35–38].
Respiratory
The magnitude of respiratory changes from increased IAP correlates directly with the degree of pneumoperitoneum [2]. With rising IAP, the diaphragm and mediastinum are displaced more cephalad, and chest excursion is restricted. This results in decreased functional residual capacity (FRC), decreased total lung compliance, increased peak inspiratory pressure, and decreased tidal volume (TV) [4, 5, 39]. As such, the ventilation perfusion mismatch expected in routine mechanical ventilation is enhanced, and atelectasis, oxygen (O2) desaturation, and hypercarbia can occur [2]. These effects are more pronounced in smaller children and neonates with an already low FRC and high oxygen consumption [21]. In healthy children, these changes usually have no clinical sequelae and can be counterbalanced by anesthetic adjustments. Indeed, the large majority of infants require at least one intervention by anesthesia to restore baseline TV and end tidal CO2 (ETCO2) [2].
With regard to retroperitoneal MIS, peak inspiratory pressures increase upon retroperitoneal space insufflation, with a resultant increase in respiratory rate and decrease in O2 saturations [12, 40]. In their prospective evaluation of 18 children undergoing retroperitoneal laparoscopy, Lorenzo et al. found that while there was a statistically significant increase in airway pressure during retroperitoneal insufflation, there was a strong trend towards normalization after completion of the procedure [18].
Cardiovascular
The cardiovascular effects of increased IAP are complex and depend on the interplay between preload, systemic vascular resistance (SVR), and cardiac contractility [4]. Positioning and hypercarbia also independently influence these factors, and consequently the prevailing combined circumstances determine the response in each patient.
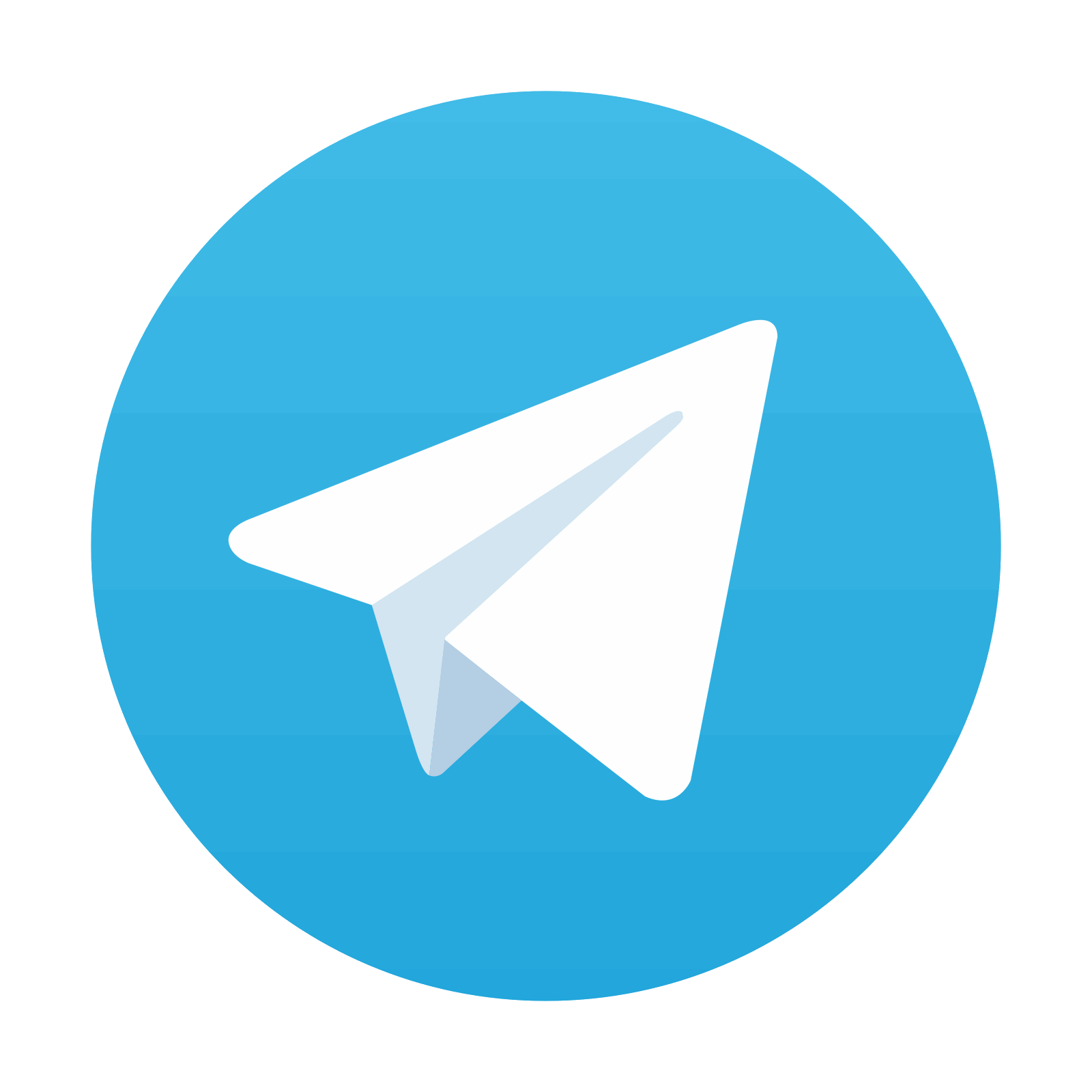
Stay updated, free articles. Join our Telegram channel

Full access? Get Clinical Tree
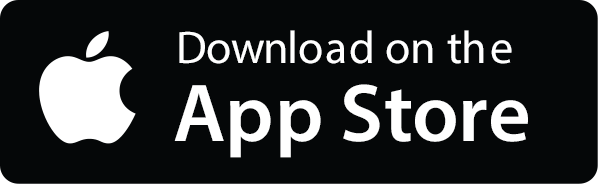
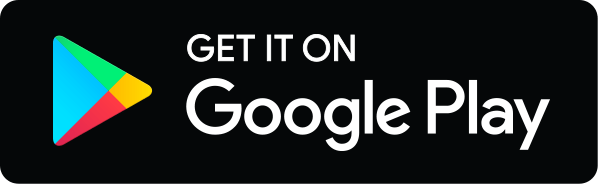