Potential benefits
Potential risks
Decrease lung injury
Cerebral vasodilation/loss of cerebral auto regulation/intraventricular hemorrhage
Neuroprotection in hypoxia-ischemia
Increase pulmonary vascular resistance
Reduction of adverse effects of hypocapnia including periventricular leukomalacia and cerebral palsy
Decrease myocardial contractility
Improved cardiac output
Increase retinopathy of prematurity
Decrease duration of assisted ventilation
We will review the physiologic rationale behind permissive hypercapnia, the experimental and clinical data assessing its potential use in neonates, and the trials that have evaluated this strategy in this vulnerable population.
27.2 Physiologic Rationale
27.2.1 Potential Advantages of Hypercapnia and Hypercapnic Acidosis
A ventilator management strategy that permits hypercapnia has several physiologic effects on gas exchange, cardiac output, and respiratory drive that may be beneficial. The increase in alveolar CO2 that occurs during permissive hypercapnia increases CO2 elimination for the same minute ventilation. This is because CO2 elimination is governed by the following equation:


In this equation, K is a constant, VCO2 is CO2 elimination, PACO2 is alveolar CO2, and VA is alveolar ventilation. If the PACO2 is allowed to rise and alveolar ventilation is kept constant, CO2 elimination will increase. Therefore, a clinician can use lower tidal volumes and lower peak inspiratory pressures (decreasing alveolar ventilation) and allow the CO2 to rise, yet maintain effective CO2 removal. The hyperbolic relationship between PaCO2 and minute ventilation permits effective CO2 removal at a lower-than-expected amount of alveolar ventilation (Boynton and Hammond 1994). Thus, for example, if alveolar ventilation decreases by half, PaCO2 will increase twofold but the increase in alveolar CO2 tends to maintain a constant CO2 elimination.
Aside from increasing the efficiency of CO2 removal, hypercapnia (achieved with inspired CO2 in animal studies) can improve ventilation-perfusion matching in the lung (Swenson et al. 1994; Brogan et al. 2004; Sinclair et al. 2006). In addition, hypercapnic acidosis permits more unloading of oxygen to the tissues due to the rightward shift of the oxygen dissociation curve (Bohr effect). Another physiologic advantage is stabilization (or increase) in respiratory drive, resulting in less apnea (Kondo et al. 2000) that can facilitate weaning an infant off the ventilator (Mariani et al. 1999; Søvik and Lossius 2004). Permissive hypercapnia also has beneficial effects related to cardiac function. Hypercapnia causes intracellular acidosis in the cardiac myocyte because of the high permeability to CO2 (Laffey and Kavanagh 1999). In animals and humans, both therapeutic hypercapnia and permissive hypercapnia have been shown to decrease cardiac contractility but increase overall cardiac output (Walley et al. 1990; Weber et al. 2000). The increase in cardiac output is believed to be due to a fall in peripheral vascular resistance following hypercapnic acidosis (Cardenas et al. 1996). Furthermore, as tidal volume and peak inspiratory pressure are lowered, mean airway pressure decreases, which also may improve preload and cardiac output (Weiner et al. 1987; Gullberg et al. 1999, 2004).
27.2.2 Potential Disadvantages of Hypercapnic Acidosis
Possible negative effects of hypercapnia on gas exchange include a small reduction in alveolar O2 concentration caused by the increased alveolar CO2. This can be overcome with slight increases in FiO2 to increase alveolar oxygen concentration. Hypercapnic acidosis may also cause an increase in pulmonary vascular resistance in some infants although this has not been observed in clinical trials. In addition, while a higher PaCO2 might be good, if the tidal volume is too low, the alveoli may collapse, resulting in right to left intrapulmonary shunting.
Hypercapnic acidosis also increases metabolic demand through an increase in work of breathing (Thome et al. 2006), but because oxygen delivery and tissue oxygenation is increased, this may not be clinically relevant. Hypercapnia may play a role in the development of retinopathy of prematurity (ROP) through retinal vessel vasodilatation, increased oxygenation, and subsequent formation of oxygen-derived free radicals (Holmes et al. 1998; Leduc et al. 2006). However, the randomized trials in neonates that tested for the presence of ROP or long-term visual outcomes reported no difference in the control versus the hypercapnia groups (Mariani et al. 1999; Thome et al. 2006; Carlo et al. 2002).
There is a particular concern in neonates of potential increased risk of IVH because of loss of cerebral autoregulation when exposed to high PaCO2. However, this is not supported by the clinical trials (see later).
27.3 Experimental Research
27.3.1 Supportive Data for Hypercapnic Acidosis
Permissive hypercapnia allows a clinician to manage a ventilated patient with the intent of reducing lung injury resulting from volutrauma. Furthermore, the resulting hypercapnic acidosis itself may be advantageous to the injured lung and to other organs. It is important to distinguish between permissive hypercapnia (the presence of hypercapnia in the setting of a ventilated patient being managed with low minute ventilation) and therapeutic hypercapnia (deliberate induction of hypercapnia by adding CO2 to inspired gases, usually done experimentally) (Rotta and Steinhorn 2006). Each intervention produces hypercapnic acidosis, but therapeutic hypercapnia allows researchers to test experimentally the independent effects of hypercapnic acidosis. For example, in an in vivo rabbit model of high-tidal volume, ventilator-induced lung injury (25 mL/kg tidal volume in both experimental groups), therapeutic hypercapnia (PaCO2 80–100 mmHg versus 40 mmHg) attenuated various measures of lung injury (Sinclair et al. 2002). Similarly, in a rat model of endotoxin-induced lung injury in which lower volumes were used (4.5 mL/kg), therapeutic hypercapnia either before or after instillation of intratracheal endotoxin also reduced lung injury indices (Laffey et al. 2004). It is interesting to note that buffering hypercapnic acidosis to maintain “normal” pH may abrogate the protective effects of hypercapnic acidosis (Nichol et al. 2009). These studies demonstrate some of the potential benefits of hypercapnic acidosis per se in adult animal models in vivo. This is important because permissive hypercapnia, while decreasing the risk of lung injury due to mechanical reasons (lung stretch), also may be beneficial because of the additive effects of hypercapnia and of acidosis. Recent experimental research suggests that the beneficial effects of hypercapnic acidosis in the adult lung may translate to the preterm lung. Strand et al. (2003) showed that in preterm lambs with ventilator-induced lung injury, those exposed to high inspired PCO2 had less lung injury by histopathology than control lambs. In newborn rats, hypercapnic acidosis had beneficial effects on pulmonary hypertension and vascular remodeling induced by chronic hypoxia (Kantores et al. 2006). Exposure to 10 % CO2 attenuated increased oxidant stress induced by hypoxia, as quantified by 8-isoprostane content in the lung, and prevented upregulation of endothelin-1, a critical mediator of pulmonary vascular remodeling. Thus, CO2 may have antioxidant properties on the lung and consequent modulating effects on the endothelin pathway. Vannucci et al. (1995) studied an immature rat model of cerebral hypoxia-ischemia, finding that mild hypercapnia was associated with less severe brain damage than normocapnic animals.
Li et al. (2006) investigated the effect of hypercapnia on overall growth characteristics, lung structure, and global gene expression in lungs of newborn mice exposed to 8 or 12 % CO2 for 2 weeks. Their research demonstrated a substantial number of genes with altered expression in mice exposed to 8 % CO2 but not in those exposed to 12 % CO2. Of particular interest was an increase in transcription of the genes encoding surfactant-associated protein A and B (Sftp-A and Sftp-B). These proteins, expressed in bronchial epithelial and type II alveolar cells, may contribute to host defense mechanisms in the lung. Overall growth (body weight), hemoglobin concentration, and lung alveolar development were normal in both the hypercapnia and control groups. Recent preliminary data demonstrate that hypercapnia may accelerate alveolar development and attenuate hyperoxia-induced fibrosis in neonatal mice (Ryu et al. 2010; Das et al. 2009). These data suggest that hypercapnic acidosis may enhance host defense mechanisms through altered gene expression and promote alveolar development in the developing lung.
One of the benefits of hypercapnic acidosis demonstrated by in vivo animal studies is reduced neutrophil influx into the lung and reduced inflammatory cytokine production (Sinclair et al. 2002; Laffey et al. 2000, 2004). Such anti-inflammatory effects of hypercapnic acidosis also have been studied in vitro using neutrophils and endothelial cells. Hypercapnia can affect various neutrophil functions such as chemotaxis by altering the ability of neutrophils to regulate intracellular pH (O’Croinin et al. 2005). Hypercapnic acidosis also can alter neutrophil adherence to pulmonary endothelial cells by decreasing endothelial expression of the adhesion molecule ICAM-1 and of the chemoattractant interleukin-8 (Takeshita et al. 2003). Furthermore, hypercapnic acidosis can decrease lipopolysaccharide (LPS)-stimulated secretion of the acute proinflammatory cytokine tumor necrosis factor alpha in isolated rat macrophages (Lang et al. 2005a). The proposed mechanism of decreased expression of ICAM-1 and inflammatory cytokines appears to be related to decreased activation of nuclear factor kappa beta, a key early regulator of gene expression in the innate immune response (Watterberg et al. 1996). The ability of hypercapnic acidosis to modulate the innate immune response has important implications for lung development. Antenatal exposure to inflammation, such as chorioamnionitis, is a frequent cause of preterm birth and increases the risk of developing BPD (Watterberg et al. 1996; Jobe 2003). Furthermore, innate immune signaling can inhibit structural lung development in experimental animal models (Prince et al. 2005; Benjamin et al. 2007). Thus, hypercapnic acidosis also may be beneficial to the developing lung via its anti-inflammatory and immune-modulating capabilities. However, more experimental research is needed to determine how hypercapnic acidosis can be optimized to protect the preterm lung.
27.3.2 Neutral or Negative Data for Hypercapnic Acidosis
Even though most of the data indicate that hypercapnic acidosis is beneficial, some studies have reported neutral or negative results. Lang et al. (2005b) used intravenous LPS injection to mimic sepsis-related lung injury in adult rabbits and demonstrated that permissive hypercapnia contributed to lung injury via amplified oxidative injury. Other researchers have shown that hypercapnia may impair cell repair mechanisms after injury (Doerr et al. 2005) and induce injury to alveolar epithelial cells by potentiating tissue nitration (Lang et al. 2000). In an adult rabbit lung injury model using saline lavage to cause surfactant deficiency, therapeutic hypercapnia did not improve oxygenation or attenuate lung injury (edema, compliance, airway pressure, or cytokine production) after high-volume ventilation (12 mL/kg versus 5 mL/kg of tidal volume) (Rai et al. 2004). In eight adult patients with ARDS exposed to hypercapnia, there was a decrease in the Qp/Qs ratio, with deterioration in gas exchange (Feihl et al. 2000). Although a recent preliminary report suggested that therapeutic hypercapnia (5.5 % inspired CO2) could protect the neonatal rat lung from hyperoxic injury (Masood et al. 2009), other investigators who used higher CO2 concentrations (8 % CO2) did not report this benefit (Das et al. 2009). Hypercapnia has been associated with increased risk of impaired cerebral blood flow autoregulation and intracranial hemorrhage in neonates (Kaiser et al. 2005). Kaiser et al. (2005) have examined the effects of endotracheal tube suctioning on cerebral autoregulation at various levels of PaCO2. They found a relationship between acute PaCO2 changes and cerebral blood flow. Marked hypercapnia can cause pressure-passive cerebral blood flow and increase risk of IVH. In another study, the same group suggested an association between severe hypercapnia in the first 72 h and IVH (Kaiser et al. 2006). It should be noted that the findings refer to fairly extreme degrees of hypercapnia, not what we typically feel to be appropriate target PaCO2 levels, even in the context of permissive hypercapnia. In their study, the association of PaCO2 and severe IVH involves very high levels (median 73.8, 25–75 % = 62.1, 84.7 for grade 3 IVH and median 73.9 25–75 % 65.4, 90.5 for grade 4 IVH). Even when a multivariate analysis was done, it is still not conclusive that there is a causative relationship, as opposed to an association of poor ventilation and severe IVH in the sickest babies. Furthermore, two large new clinical data suggest that severe hypocapnia and wide fluctuations in PaCO2 in addition to hypercapnia were associated with increased risk of hemorrhage (Fabres et al. 2007; McKee et al. 2009) (see below). Nonetheless, there certainly is a plausible pathophysiologic mechanism and it is likely that very high PaCO2 may be dangerous in the first 4 days of life. Other studies have shown that hypercapnic acidosis increases cerebral oxygen delivery (Hare et al. 2003) and that the CO2-induced alterations in cerebral blood flow appear to be reversible (Hino et al. 2000).
A recent retrospective study of 849 infants weighing 1,250 g or less revealed that severe hypocapnia, severe hypercapnia, and wide fluctuations in PaCO2 were associated with increased risk of hemorrhage (Fabres et al. 2007). The OR for IVH was 1.97 (1.23–3.15) if the maximal PaCO2 was higher than 60 mmHg during the first 4 days of life and 2.51 (1.53–4.12) when the minimal PaCO2 level was below 39 mmHg. Another retrospective study from the same group found that extreme fluctuations in PaCO2 and higher max PaCO2 were associated with worse neurodevelopmental outcomes in extremely low birth weight infants (McKee et al. 2009). They may indicate either a greater severity of illness or contribution of PaCO2 to pathophysiology of adverse neurodevelopment. Most importantly, the randomized, controlled trials of permissive hypercapnia in neonates have not reported an increase in intracranial hemorrhage.
27.4 Clinical Data
Due to the beneficial results in adult randomized trials (Petrucci and Iacovelli 2004) and the association between hypocapnia and poor pulmonary and neurodevelopmental outcomes (Collins et al. 2001), permissive hypercapnia has emerged as a potential strategy to decrease morbidity in neonates. Two retrospective studies in neonates reported that higher PaCO2 values were associated with less lung injury (Kraybill et al. 1989; Garland et al. 1995). Kraybill and colleagues (1989) performed a multicenter analysis on 235 infants whose birth weights were between 751 and 1,000 g before the introduction of surfactant administration. Garland and coworkers (1995) analyzed data on 188 infants weighing less than 1,700 g who received surfactant. Both of these studies concluded that ventilatory strategies leading to early hypocapnia resulted in an increased risk of BPD (odds ratio [OR] of 1.45 and 95 % confidence intervals [CI] of 1.04, 2.01 and OR of 4.3 and 95 % CI of 1.5, 12.0, respectively). Peak levels of PaCO2 greater than 50 mmHg in the first 4 postnatal days and greater than 40 mmHg before the administration of surfactant were associated with a lower incidence of BPD in these two studies. A population-based study of 407 infants younger than 28 weeks or less than 1,000 g using historic controls also suggested that permissive hypercapnia resulted in a decreased rate of BPD (Kamper et al. 2004). The observations that low PaCO2 values during the first days after birth are associated with more lung disease are counterintuitive at first glance. The usual assumption is that infants who have lower PaCO2 values have less severe pulmonary disease and are at lower risk of lung injury. However, low PaCO2 values may reflect ventilator management strategies that result in overventilation in infants who have favorable lung compliance. This mechanism could be an important cause of lung injury, particularly in neonates who have less severe lung disease. Thus, it is possible that ventilatory strategies that target mild hypercapnia or prevent hypocapnia result in reduced incidence or severity of lung injury (Carlo 2007). Retrospective studies in neonates who have congenital diaphragmatic hernia, including the data on 1,210 neonates from 53 centers of the Congenital Diaphragmatic Study Group, reported that permissive hypercapnia resulted in an increase in survival (Bagolan et al. 2004; Dudell and CDH Study Group 2006) and a decreased need for extracorporeal membrane oxygenation (Dudell and CDH Study Group 2006).
There are two situations in which PaCO2 levels could be allowed to rise a little over “normal” values: first, the neonate with respiratory distress who has not been intubated and in whom neonatologists tolerate higher PaCO2 levels precisely to avoid intubation and its associated risks (endotrauma, volutrauma, biotrauma) and, second, the neonate who is already on the ventilator in whom higher PaCO2 levels are accepted to avoid high ventilator settings.
27.4.1 Neonates with Respiratory Distress but Not Intubated
After the report by Avery et al. (1987) on 1987 showing the lowest rate of BPD in the center in which physicians practiced a permissive hypercapnia approach, several investigators have focused on the advantages of an early CPAP strategy. Avery’s publication was a retrospective analysis of 1,625 VLBW infants born between 1982 and 1984 in eight centers. Overall survival was 78–84 %, and BPD rate was significantly lower in Columbia, where 42 % of those infants had received mechanical ventilation and 37 % had been treated with early CPAP. The “Columbia approach,” as it was started to be called, included early nasal CPAP and acceptance of PaCO2 levels as high as 60 mmHg. By that time, the same group was proposing a “gentler” approach to manage term neonates with PPHN (Wung et al. 1985), avoiding the widespread hyperventilation-hyperoxemic strategy. Unfortunately, the approach was not scientifically tested until recent years.
In 2000, Van Marter et al. (2000) published a cohort study including 441 VLBW infants (500–1,500 g) trying to identify variables associated with the development of BPD. The study compared outcomes in three clinical centers, and again, Columbia had a much lower rate of BPD, with lower use of surfactant and higher use of early CPAP. A multivariate analysis showed that intubation significantly increased the risk of BPD (OR 13.4, 95 % CI 5.9–30.7 on the day of birth).
In 2005, Ammari et al. (2005) shared the Columbia experience in a publication that intended to find variables associated with early CPAP failure in VLBW infants. They found the following ORs: GA (each week), 0.53 (0.43–0.65); PPV in the delivery room, 2.37 (1.02–5.52); AaPO2 >180 mmHg, 2.91 (1.30–6.55); and severe RDS by X-ray, 6.42 (2.75–15). It was interesting that 92 % of infants between 1,000 and 1,250 g were successfully managed with early nasal CPAP, while the percentage was 33 % for those infants with BW below 700 g.
It is known that early surfactant administration reduces mortality and respiratory morbidity while the use of mechanical ventilation increases the risk for bronchopulmonary dysplasia. So, over the last few years, one of the controversies in the management of extremely preterm infants has been whether to intubate and give early surfactant or to start early nasal CPAP, avoiding intubation (Finer 2006).
Several observational studies, most with a “before-after” design, suggest that the use of early nasal CPAP reduces BPD rates (Dunn and Reilly 2003; Bohlin et al. 2008). Expert opinions abound on this issue, and even when (following Roman philosopher Seneca) opinions should be weighed and not just counted, they are the “lowest level of evidence.” Neonatologists have learned that the best way to protect neonates from “good ideas” is to test these ideas in randomized control trials.
27.4.1.1 Randomized Controlled Trials
A few years ago, two studies evaluated the potential beneficial effects of prophylactic nasal CPAP in preterm infants beginning in the delivery room. Sandri et al. (2004) performed an RCT in 17 NICUs in Italy, including 230 neonates 28–31 weeks of whom 80 % had received antenatal steroids. One group was managed with prophylactic nasal CPAP and the other one with “rescue” nCPAP if the infant required FiO2 >0.4. Both the use of surfactant (22 %) and mechanical ventilation (12 %) were similar between the two groups. There were no significant differences in other relevant outcomes.
In another multicenter trial, Finer et al. (2004) evaluated the feasibility of implementing prophylactic nasal CPAP in younger infants. This was a pilot study from the NICHD Neonatal Network in which 104 extremely preterm infants (23–27 weeks of GA) were randomized to nCPAP (or use of PPV with PEEP) in the delivery room or to starting nCPAP once in the NICU. Ninety-eight percent of the mothers received antenatal steroids. While the study showed that the very early CPAP approach was feasible, there were no differences between the two groups in any of the major outcomes analyzed. An interested observation of this study was that 20 % of these ELBW infants were never intubated within the first week of life.
More recently, two RCT addressing this issue have been published. The COIN trial (Morley et al. 2008) (Cpap Or INtubation) included 613 neonates of 25–28 weeks of GA who had spontaneous respiration after birth and signs of respiratory distress. They were randomized to a nasal CPAP group (8 cm H2O) or to a group with intubation and mechanical ventilation soon after birth (in the delivery room). Patients in the CPAP group received mechanical ventilation if they had recurrent apneas, a pH <7.25 with PaCO2 >60 mmHg, or FiO2 requirements >0.6 (to keep SpO2 >90 %). The primary outcome was death or BPD (O2 at 36 weeks). The results were that CPAP failure (intubation requirement within the first 5 days) was 46 %, and the rate of death or BPD was similar in both groups (CPAP 34 %; MV 39 %, OR 0.8; 95 % CI 0.58–1.12) but with a trend for improved outcomes in the CPAP group. While there were no differences in most of the secondary outcomes, the incidence of pneumothorax was three times higher in the CPAP group. It has been speculated that either the high CPAP level (8 cm H2O) or the delay in the use of surfactant might be responsible for this difference. In subgroup analyses, the rate of death or BPD was almost the same for the most immature infants (25–26 weeks) with a trend to lower incidence within infants 27–28 weeks in the CPAP group (24.6 % versus 31.3 %; OR 0.72; 95 % CI 0.46–1.11).
The NICHD Neonatal Network designed the SUPPORT (SUrfactant, Positive Pressure, and Oxygenation Randomized Trial) trial to compare early CPAP treatment with early surfactant treatment in extremely preterm infants (SUPPORT Study Group of the Eunice Kennedy Shriver NICHD Neonatal Research Network et al. 2010). This was a multicenter trial with a factorial design, in which there was another intervention that was being tested (different SpO2 target levels). It should be noted that in this study, not only an early CPAP strategy was tested but also a whole ventilator management, as the authors compared a strategy of treatment with CPAP and protocol-driven limited ventilation begun in the delivery room and continued in the neonatal intensive care unit (NICU) with a strategy of early intratracheal administration of surfactant (within 1 h after birth) followed by a conventional ventilation strategy. Extubation criteria, for example, were different for the two groups. In terms of PaCO2 levels, the threshold for the CPAP group was 65 mmHg (both for intubation and for extubation), while it was 50 mmHg for extubation in the control group (showing that even in the standard group, higher-than-“normal” PaCO2 levels are currently being accepted). The primary outcome was death or BPD (O2 at 36 weeks). The study included 1,316 preterm infants 24–27 weeks of GA. The rates of the primary outcome did not differ significantly between groups (48.7 % versus 54.1 %) but again favored the CPAP group. The adjusted relative risk for CPAP was 0.91; 95 % CI 0.83–1.01.
The Neocosur South American Network has recently presented the results of another multicenter RCT (Tapia et al. 2012). This study was designed to determine if a strategy of prophylactic bubble CPAP in VLBW infants could decrease the need for mechanical ventilation during the first week of life without affecting major morbidity/mortality. Two hundred and fifty-six infants (800–1,500 g) were randomized to either bubble CPAP (5 cm H2O) or oxyhood if they were breathing spontaneously at 5 min of age. Intubation was performed if FiO2 >0.35, but in the CPAP group, infants were extubated within 10 min after surfactant administration. Infants were kept on CPAP while PaCO2 was below 60 mmHg and pH >7.20. Mechanical ventilation was received in 30 % of infants in the CPAP group and 52 % in the control group (p 0.001). The combined outcome of death or BPD (O2 at 36 weeks) was 15 % in the CPAP group and 19 % in the control group (RR 0.8, 95 % CI 0.46–1.36). No differences in other outcomes (including gas leaks) were seen between groups.
All these studies compare early CPAP with early surfactant. An interesting “in between” strategy has been proposed originally by investigators in Sweden, combining the beneficial effects of the two treatments (early CPAP and early surfactant) (Verder et al. 1994, 1999, 2009). The “Scandinavian approach” or INSURE method (Intubation, Surfactant, Extubation) consists in intubating the infant for surfactant administration with rapid – within 10 min – extubation to nasal CPAP. Several studies have looked at this strategy, and the results consistently show a reduction in the need for mechanical ventilation. They have been reviewed by the Cochrane Library (Stevens et al. 2007), and the meta-analysis shows a decreased need for mechanical ventilation (RR 0.67; 0.57–0.79), a decrease in BPD at 28 days (RR 0.51; 0.26–0.99), and a decrease in air leak syndromes (RR 0.52; 0.28–0.96).
This systematic revision and meta-analysis has not included the following three recent studies. Rojas et al. (2009) performed a RCT in eight centers in Colombia, including 278 preterm infants (27–31 weeks of GA) with RDS. They were randomized to an INSURE group (with rapid extubation to nasal CPAP) or to a control group, receiving nasal bubble CPAP (6 cm H2O). The primary outcome was the requirement of mechanical ventilation (following specifically defined criteria: FiO2 >0.75 for 30 min, persistent or recurrent SpO2 <80 %, PaCO2 >65 mmHg + pH <7.22, or clinical deterioration). The use of MV was significantly reduced (39–26 %, RR 0.69; 0.49–0.97) and the rate of gas leaks was also reduced from 9 % in the control group to 2 % in the treatment group (RR 0.25; 0.07–0.85). The combined outcome of death or BPD (O2 at 36 weeks) was 54 % in the CPAP group and 63 % in the control group (RR 0.86, 95 % CI 0.7–1.04). There were no differences in mortality or any secondary outcome measure.
Sandri et al. (2010) recently published the CURPAP study, an international randomized controlled trial, designed to evaluate the efficacy of combining prophylactic surfactant and early nasal CPAP in preterm infants. The purpose of this study was to compare the administration of prophylactic surfactant followed by nCPAP versus early nCPAP institution with early rescue surfactant. Two hundred and eight newly born infants (25–28 weeks) with respiratory insufficiency but spontaneous respirations were randomized to a group of prophylactic surfactant and rapid extubation to nCPAP or to an nCPAP group (6–7 cm H2O) with rescue surfactant if needed. The CPAP failure definition was the same for both groups and considered any of the following: FiO2 requirement >0.4 to maintain SpO2 85–92 % for at least 30 min, significant apnea, respiratory acidosis defined as PaCO2 >65 mmHg and pH <7.20, or clinical deterioration. The primary outcome measure was the need for mechanical ventilation within the first 5 days of life. No differences were found in either the primary (31 % versus 33 %) or secondary outcome measures, except in the use of surfactant. The combined outcome of death or BPD (O2 at 36 weeks) was 21 % in the CPAP group and 22 % in the control group. There was a trend over a higher rate of pneumothorax in the INSURE group (6.7 % versus 1 %). In this study, prophylactic surfactant was not superior to early rescue surfactant in decreasing the need for MV and the incidence of main morbidities of prematurity in spontaneously breathing 25–28 weeks preterm infants on nCPAP.
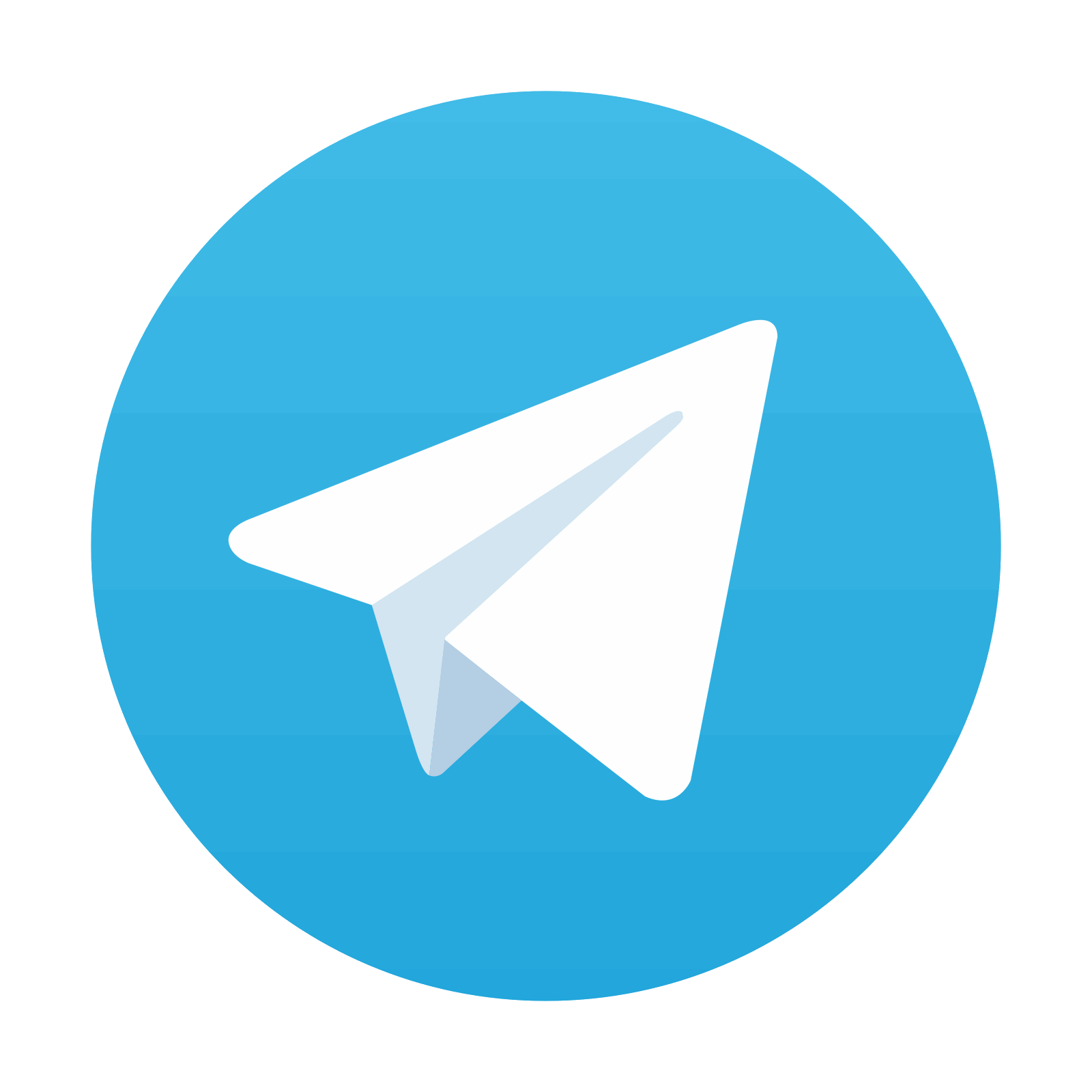
Stay updated, free articles. Join our Telegram channel

Full access? Get Clinical Tree
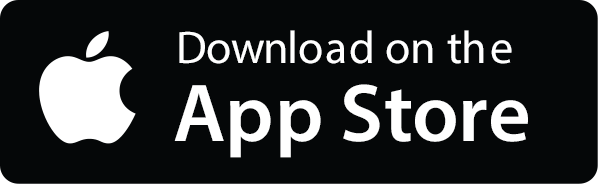
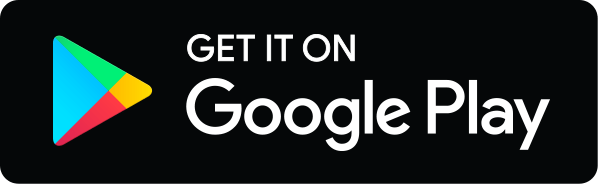