Percutaneous Transtracheal Ventilation
Manoj K. Mittal
Jill M. Baren
Introduction
The importance of maintaining adequate oxygenation and ventilation for the critically ill child cannot be overstated. In most cases, standard airway interventions, such as bag-valve-mask (BVM) ventilation and endotracheal intubation, can be performed successfully without incident. However, physicians responsible for managing critically ill children must also be prepared for those situations in which standard methods unexpectedly fail. Such circumstances require knowledge of alternative techniques for providing respiratory support (see also Chapter 17). Percutaneous transtracheal ventilation (PTV), also called “translaryngeal ventilation,” is one such technique. Although sometimes a point of confusion, PTV should not be mistaken for high-frequency transtracheal jet ventilation, which involves delivering very small volumes of oxygen at extremely high rates. High-frequency transtracheal jet ventilation requires special equipment not generally found in the emergency department (ED).
Initial animal studies in the development of PTV dealt with passive transtracheal oxygenation (1). This method, however, was abandoned in favor of intermittent administration of compressed air via a transtracheal catheter, since this provides both ventilation and oxygenation (2). Successful human studies and various modifications of this technique followed, as well as data showing that transtracheal gas exchange could sustain life for prolonged periods of time (3,4,5). Smith and Ravussin subsequently published reports documenting the successful use of PTV in children aged 4 months to 11 years (6,7). Considering the potential complications associated with surgical cricothyrotomy and tracheotomy in young children, PTV should be considered the invasive (surgical airway) rescue modality of choice in this age group. The advantages of needle cricothyrotomy with PTV over surgical cricothyrotomy include faster performance, easier technique, less equipment required, no need for an assistant, smaller scar formation, decreased bleeding, and less frequent subglottic or glottic stenosis. The disadvantages of PTV include incomplete control of the airway, greater potential for aspiration, increased likelihood of barotrauma, and inability to perform adequate tracheal suctioning (8).
Anatomy and Physiology
The cricothyroid membrane is bound superiorly by the thyroid cartilage and inferiorly by the cricoid cartilage. Bennett et al. studied the anatomy of the cricothyroid membrane in 13 adult fresh cadavers (9). The vertical measurement was 8 to 19 mm (mean, 13.7 mm), and the transverse measurement was 9 to 19 mm (mean, 12.4 mm). Similar information for pediatric patients has not been published. The cricothyroid arteries typically course through the apical portion of the membrane, although aberrant vessels may rarely complicate procedures in this area (10). For young infants, performing PTV can be difficult due to the small size of the cricothyroid membrane. However, this is also true of other invasive airway techniques, and PTV can often be performed more rapidly and with fewer complications. Furthermore, if the cricothyroid membrane of a younger patient cannot be located, the procedure can be performed by introducing the needle through the tracheal cartilage without additional risk to the patient (see below).
The operator must be thoroughly familiar with the anatomy of the anterior neck in order to perform this procedure successfully (Fig. 18.1). Five specific landmarks should be identified: the hyoid bone, the laryngeal prominence of the thyroid cartilage, the cricothyroid membrane, the cricoid cartilage, and the remaining tracheal rings. The laryngeal prominence develops during adolescence and is easily palpable in most adults, whereas the most readily identifiable landmarks
in infants and young children are the hyoid bone and cricoid cartilage.
in infants and young children are the hyoid bone and cricoid cartilage.
Delivered tidal volume during PTV is affected by a number of factors, including lung compliance, airway resistance, size of catheter, inspiratory pressure, and duration of inspiration (11). Jacobs estimated that a maximum 70% of the volume of oxygen delivered to the catheter tip reaches the lower respiratory tract of adults with normal lung compliance (12). The remainder passes up through the glottic opening and out the nose and mouth, decreasing the efficiency of gas exchange when compared with endotracheal intubation (4,12,13,14). Because of the relatively small size of the airways, pediatric patients generally have significantly greater airway resistance than adults. Thus even with a small child, it is important to use a catheter with an adequate luminal diameter and a high-pressure oxygen source to perform this procedure effectively. When used with a low-pressure oxygen delivery system, PTV provides adequate oxygenation but may not provide adequate ventilation, causing retention of CO2 over time. An example of such a system would be a self-inflating bag-valve-mask circuit connected to the catheter via a 3.0-mm internal diameter (ID) endotracheal tube adapter (see “Equipment”). It has been suggested that use of a large-diameter catheter will largely overcome the problem of increasing hypercarbia with a low-pressure system (15), but Marr and Yamamoto recently showed that catheter size does not substantially affect gas flow
rates (16). They found that Poiseuille’s law is not applicable in this situation, because it applies to laminar flow rather than gas flowing in a turbulent fashion, which is what occurs when oxygen is forced at high pressure through a narrow tube. Consequently, these authors recommend use of high-pressure oxygen from a wall source, although they contend that “if a device to perform this is not available, then a self-inflating bag-valve-mask circuit may still be sufficient” for PTV (16).
rates (16). They found that Poiseuille’s law is not applicable in this situation, because it applies to laminar flow rather than gas flowing in a turbulent fashion, which is what occurs when oxygen is forced at high pressure through a narrow tube. Consequently, these authors recommend use of high-pressure oxygen from a wall source, although they contend that “if a device to perform this is not available, then a self-inflating bag-valve-mask circuit may still be sufficient” for PTV (16).
PTV provides oxygen via intermittent bulk flow of gas under high pressure. Exhalation is achieved passively through the elastic recoil of the chest, with gases being expelled through the mouth and nose. The “ball-valve” effect of most partial laryngeal obstructions permits adequate exhalation during PTV, but complete obstruction prevents any egress of gas and may result in a dangerously rapid rise in intrapulmonary pressure (17). With a 50-psi oxygen source, volumes of 950 mL/sec, 1,200 mL/sec, and 1,300 mL/sec can be delivered through 16-gauge, 14-gauge, and 13-gauge catheters, respectively (18). These flow rates are more than adequate for maintaining ventilation and oxygenation in adults. Actual peak inspiratory pressures and intratracheal pressures are relatively low, even with a driving pressure of 50 psi; however, smaller catheters (16- to 18-gauge) and lower driving pressures (25 to 35 psi) must be used for pediatric patients in order to prevent barotrauma. Tran et al. compared the efficacy of PTV in the unparalyzed and paralyzed states using a canine model and showed that gas exchange and lung mechanics were similar. This suggests that it may be unnecessary to induce paralysis when using PTV for emergency ventilation of a sedated patient (19).
The primary drawback to long-term use of PTV is CO2 retention. For this reason, PTV previously has been viewed only as a temporizing procedure. However, when adequate oxygenation is maintained, even relatively high levels of hypercarbia may not be as deleterious as once believed (20,21). In fact, PTV has been shown to be safe for hours at a time, limited only by the ability of the system used to deliver humidified gases. Only in the setting of increased intracranial pressure or complete airway obstruction should PTV be considered solely as a temporizing measure.
Indications
Indications for the emergent use of PTV are similar to those for surgical cricothyrotomy; that is, any patient whose airway cannot be maintained with standard interventions or a “nonsurgical rescue” procedure (such as insertion of a laryngeal mask airway) should be considered a possible candidate. Potential clinical scenarios include severe blunt or penetrating maxillofacial trauma; laryngeal foreign bodies that cannot readily be removed; severe swelling of the upper airway from infection (e.g., epiglottitis), an allergic process (e.g., hereditary angioedema, snakebite), or local trauma (e.g., airway thermal or chemical burns); and congenital anomalies such as Treacher Collins syndrome or Pierre Robin syndrome (22,23,24). PTV has also been used to provide oxygenation and ventilation before potentially difficult intubations as well as for elective procedures such as head and neck surgery (6,7,13,25,26). With younger children, PTV should always be considered before surgical cricothyrotomy because it is associated with far fewer complications and can be completed in a much shorter period of time (see also Chapter 26). PTV should not be performed when there is known damage to the cricoid cartilage or in the setting of tracheal rupture (8). These situations represent the only absolute contraindications for this procedure. Relative contraindications, such as mild to moderate local swelling of the anterior neck or the presence of a hematoma, should be balanced against the potentially devastating complications of failing to provide adequate airway intervention.
Controversy surrounds the use of PTV in the setting of complete upper airway obstruction. A blockage cephalad to the catheter will prevent passive exhalation of delivered gases through the mouth and nose. In a study of animals subjected to total airway obstruction, Jorden et al. reported that standard PTV caused massive distention of the lungs, severe barotrauma, and subsequent death (27). However, additional studies have challenged the dogma that PTV is absolutely contraindicated in the presence of complete upper airway obstruction (28,29,30,31,32). Successful application of PTV in animals with complete airway obstruction using prolonged exhalation times and large-ID catheters has been reported (30). Frame et al. reported a feline study of total airway obstruction using low flow rates (3 to 5 L/min) in which adequate oxygenation and ventilation were maintained without complication (32); they hypothesized that these data might be extrapolated to infants. Without the benefit of further studies to settle this issue, it seems reasonable to use PTV as a temporizing measure for infants and younger children when complete upper airway obstruction is present and other methods have been unsuccessful. In such a situation, prolonged exhalation times, lower oxygen delivery pressures, and the use of larger than normal catheters would all be required. For older children and adolescents, surgical cricothyrotomy remains the procedure of choice for emergent airway management in the presence of complete upper airway obstruction.
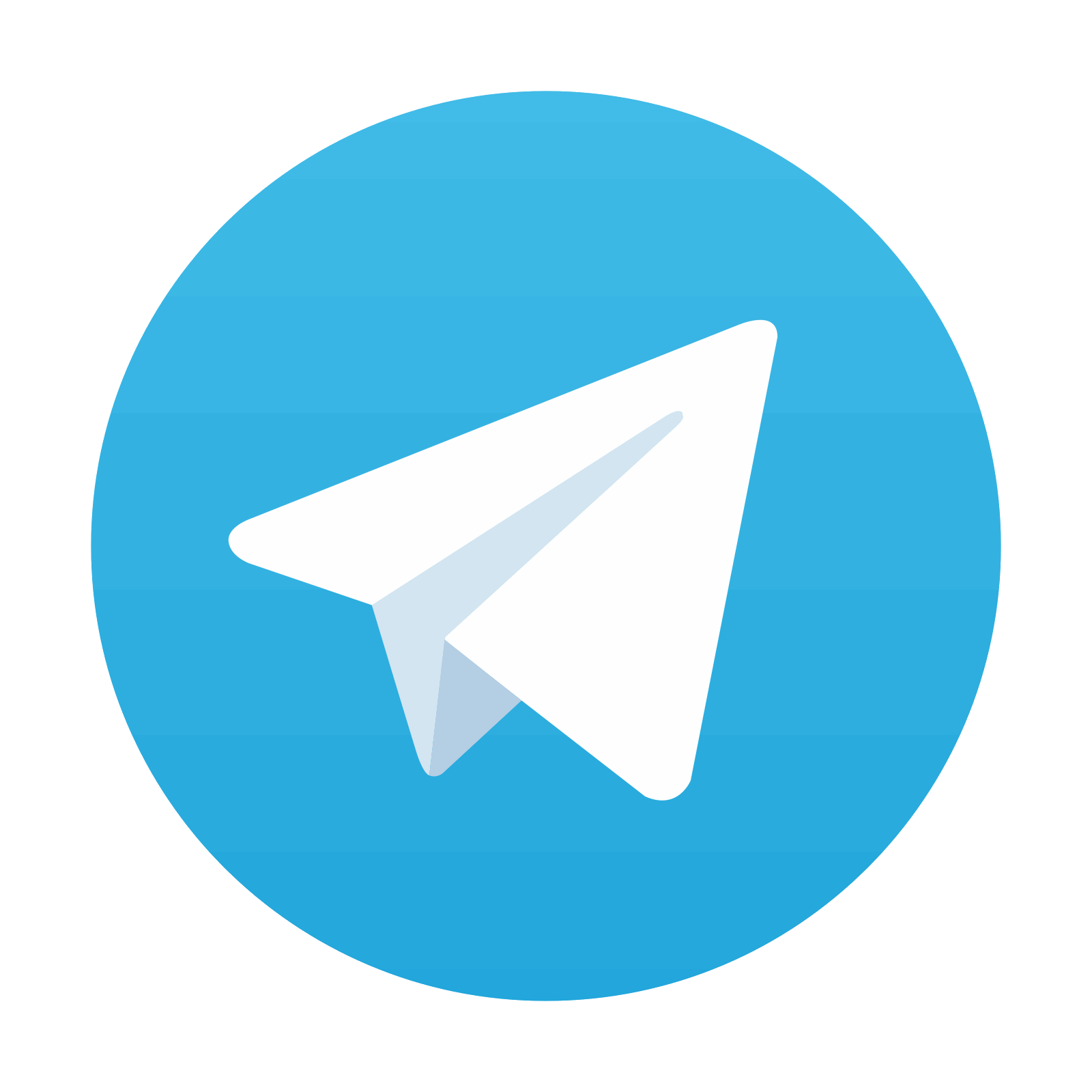
Stay updated, free articles. Join our Telegram channel

Full access? Get Clinical Tree
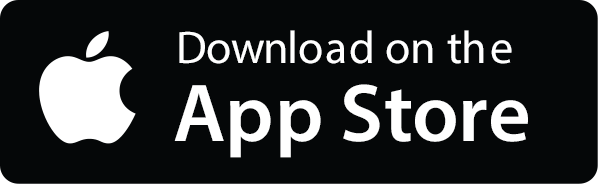
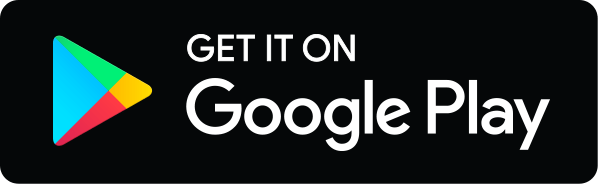