Oxygen Delivery, Suctioning, and Airway Adjuncts
Richard J. Scarfone
Introduction
Adults typically suffer sudden death as a result of primary cardiac events such as myocardial infarctions or dysrhythmias. In contrast, most pediatric cardiac arrests occur secondary to respiratory failure leading to hypoxemia and acidosis (1). As many as one quarter of hospitalized children experiencing cardiopulmonary arrest can be resuscitated with airway and ventilatory interventions alone (2). Status asthmaticus, bronchiolitis, pneumonia, and laryngotracheobronchitis are among the many pulmonary diseases commonly seen in children that may lead to respiratory failure. In addition, status epilepticus, medication overdoses, and sepsis are just a few of the many clinical conditions that may cause hypoventilation or apnea. It is especially important, then, for those providing emergency care to children to be skillful in the management of respiratory diseases and dysfunction.
The first step in the assessment of seriously ill or injured children is the evaluation of the patency of the airway and the adequacy of ventilation. For children who have mild to moderate respiratory distress and patent airways, simple delivery of oxygen may be the only method of support necessary. For those with airway obstruction, suctioning and/or the introduction of a pharyngeal airway may serve as a temporizing measure prior to endotracheal intubation or as a definitive treatment of the problem. This chapter describes oxygen delivery and the proper methods for performing these adjunctive airway procedures for infants and children with respiratory distress.
Anatomy and Physiology
Airway Anatomy
Beginning at the nose and lips and proceeding down the airway of the infant or young child, several important anatomic differences are encountered as compared with the adult (Fig. 13.1). Many of these differences predispose the child to airway obstruction. For example, the tongue of the infant or young child is relatively large in proportion to the oral cavity. In addition, since the larynx has a more superior position in the neck of a pediatric patient compared with an adult, the tongue has a more rostral location in the hypopharynx (3). Consequently, a child lying supine may develop airway obstruction due solely to posterior displacement of the tongue, a problem that can often be alleviated with a pharyngeal airway. Infants are also obligate nasal breathers until approximately 3 to 5 months of age (3). As a result, swelling of the nasal mucosa, thick rhinorrhea, nasal foreign bodies, or choanal atresia may cause significant respiratory distress in the young infant. Perhaps the most obvious and important difference from the adult patient is that children have smaller corresponding airway luminal diameters. Because the resistance to air flow is inversely related to the fourth power of the radius (Poiseuille’s law), disease states that cause further narrowing of the airways (edema, secretions, etc.) disproportionately increase the work of breathing and the resulting oxygen demand in children compared with adults (Fig. 13.2). Further discussion of pediatric airway anatomy can be found in Chapters 14 and 16.
Physiology of Oxygen Utilization and Delivery
The oxygen consumption index of an infant is roughly twice that of an adult, due primarily to a higher rate of metabolism. As a result, pediatric patients develop hypoxemia more rapidly in response to inadequate oxygen delivery. Most practitioners have witnessed the precipitous decline in oxygen saturation that can occur when oxygen flow to a child in respiratory distress is briefly interrupted.
In general, the rate of oxygen flow delivered should be sufficient to maintain an oxygen saturation of 100% whenever possible. Although the concentration of oxygen delivered to the patient (FiO2) corresponds to the rate of flow, a linear relationship does not exist. A number of other important variables also contribute to the FiO2. These include the patient’s nasal and oropharyngeal resistance as well as factors that may affect the amount of atmospheric (room) air that mixes with the administered oxygen—inspiratory flow rate, minute ventilation, and tidal volume. As a rule, the greater the patient’s inspiratory flow rate (which is influenced by both minute ventilation and tidal volume), the greater the mixing of relatively oxygen-poor atmospheric air with administered oxygen and therefore the lower the FiO2 for any given oxygen flow rate. In other words, when a patient inhales with a greater velocity than the highest possible rate of oxygen flow, atmospheric air must enter the system to make up the difference.
For example, assuming a constant oxygen flow of 3 L/min, a larger patient with a minute ventilation of 12 L/min will breathe more atmospheric air than a smaller patient with a minute ventilation of 6 L/min. Although the rate of oxygen flow is the same, the FiO2 is greater for the patient with a lower minute ventilation. Thus, an older child will need a higher rate of oxygen flow to achieve the same FiO2 as a younger child. In addition, the specific delivery system used will influence the maximal oxygen concentration delivered to the patient. At a given oxygen flow, a nonrebreathing mask will provide a higher FiO2 than a standard mask, because the latter system allows for greater entry of atmospheric air.
Oxygen Delivery
Indications
There are several diseases affecting children that may result in hypoxemia and for which the administration of oxygen is indicated. The most common of these are asthma and bronchiolitis. In these two conditions, bronchoconstriction and airway inflammation increase lower airway resistance, contribute to atelectasis and ventilation/perfusion mismatching, and increase the patient’s work of breathing and oxygen consumption. The degree of hypoxemia may be exacerbated if the child has underlying chronic lung disease such as cystic fibrosis. Pneumonia and laryngotracheobronchitis (croup) also commonly affect children and may necessitate the administration of oxygen. In addition to primary pulmonary processes, other common indications for oxygen administration include status epilepticus and hypoventilation secondary to a medication overdose, such as with a sedative or narcotic. Hypercarbia and acidosis may be relatively well tolerated by a child, but even a short period of oxygen deprivation can lead to bradycardia or cardiac arrest (2). Once it is determined that the spontaneously breathing child with respiratory distress has a patent airway, oxygen should be administered empirically.
Cyanosis is the most definitive physical finding indicating hypoxemia. Typically, the perioral region and distal extremities are the first areas of the body to become cyanotic. Other signs of hypoxemia include agitation, lethargy, and bradycardia. Determination of oxygen saturation by pulse oximetry provides a means of quantifying the degree of hypoxemia (see Chapter 75). Unless adequacy of ventilation is a concern, there is
usually little need to perform a painful and time-consuming arterial puncture for blood gas analysis. Pulse oximetry offers a noninvasive, “real-time” modality that rapidly gives an indirect but generally reliable indication of the partial pressure of oxygen (PaO2). In interpreting the pulse oximeter reading, it is important to remember that the patient will maintain an oxygen saturation over 90% as long as the PaO2 is above 60 mm Hg, even if the PaO2 is decreasing. Physiologically, this corresponds to the initial flat segment of the oxyhemoglobin dissociation curve (Fig. 13.3). Once the PaO2 falls below 60 mm Hg, however, oxygen saturation will drop dramatically as the patient reaches the steep segment of the curve. Therefore, oxygen saturation of 90% is only minimally acceptable in most clinical situations. If an oxygen saturation of at least 90% cannot be maintained with a given method of delivery, a system capable of administering a higher concentration should be employed. In such cases, controlled or assisted ventilation may also prove necessary.
usually little need to perform a painful and time-consuming arterial puncture for blood gas analysis. Pulse oximetry offers a noninvasive, “real-time” modality that rapidly gives an indirect but generally reliable indication of the partial pressure of oxygen (PaO2). In interpreting the pulse oximeter reading, it is important to remember that the patient will maintain an oxygen saturation over 90% as long as the PaO2 is above 60 mm Hg, even if the PaO2 is decreasing. Physiologically, this corresponds to the initial flat segment of the oxyhemoglobin dissociation curve (Fig. 13.3). Once the PaO2 falls below 60 mm Hg, however, oxygen saturation will drop dramatically as the patient reaches the steep segment of the curve. Therefore, oxygen saturation of 90% is only minimally acceptable in most clinical situations. If an oxygen saturation of at least 90% cannot be maintained with a given method of delivery, a system capable of administering a higher concentration should be employed. In such cases, controlled or assisted ventilation may also prove necessary.
Equipment
When administering oxygen, the clinician must first determine the concentration to be delivered and then select an appropriate delivery system. These decisions are affected by a number of patient variables, including age, degree of cooperation, respiratory effort, and extent of hypoxemia. For example, an older child in mild to moderate respiratory distress with minimal hypoxia may receive oxygen via a low-flow system such as a nasal cannula or simple mask. However, many younger children become extremely agitated and uncooperative when such devices are used, increasing oxygen consumption and potentially exacerbating hypoxemia. In such cases, it may be more effective to use a less efficient but better tolerated delivery method, such as a hollow plastic tube held close to the child’s face (“blow-by” method). For the child in greater distress, a nonrebreathing mask may be necessary to maintain adequate oxygen saturation. The clinician should be knowledgeable about the characteristics of each delivery system, the manner in which they are best used, and the advantages and disadvantages of each.
Nasal Cannula
This simple low-flow oxygen delivery system consists of a small-diameter hollow plastic tube. One end of the tube is connected to the oxygen source via an adapter, while two short prongs at the other end are placed into the anterior nares, delivering oxygen into the nasopharynx (Fig. 13.4). This system is most useful when a low oxygen flow (3 L/min or less) is adequate to maintain an acceptable oxygen saturation. As stated earlier, relatively high oxygen concentrations may be delivered to infants and young children using a low-flow system such as a nasal cannula, because the rate of oxygen flow may match or exceed the patient’s inspiratory flow rate and minute ventilation. Its principal advantage is that the light plastic tubing is less bulky and often better tolerated
than a mask. A nasal cannula device is particularly useful for young infants who are obligate nasal breathers and for whom a properly sized mask may not be available or is poorly tolerated.
than a mask. A nasal cannula device is particularly useful for young infants who are obligate nasal breathers and for whom a properly sized mask may not be available or is poorly tolerated.
The main disadvantage of the nasal cannula system is that it is difficult to deliver oxygen in excess of 3 L/min. Higher flow rates cause irritation of the nasopharynx and are therefore not well accepted by most children. In addition, this is obviously not a “closed” system, and the FiO2 may vary widely if the child is mouth breathing or crying or the nares are obstructed. Consequently, a nasal cannula may not meet the oxygen demands of a patient requiring consistent delivery of a higher FiO2.
Simple Oxygen Mask
This delivery system consists of a clear plastic or rubber mask that fits snugly over the nose and mouth (Fig. 13.5). Centers caring for children should have a wide range of sizes available. Transparent masks allow for the visualization of vomitus, blood, or other secretions. Each side of the mask has openings that serve as exhalation ports. These openings also permit inhalation of room air if the oxygen flow rate is less than the patient’s inspiratory flow rate or if the oxygen source becomes disconnected. The simple mask may be used when a relatively low oxygen flow is adequate to maintain an appropriate oxygen saturation. This system can be expected to deliver a higher FiO2 than can be achieved with a nasal cannula, but it may not be as well tolerated. At an oxygen flow rate of 6 to 10 L/min, a simple oxygen mask will deliver an oxygen concentration of between 35% and 60%. The principal disadvantage of this system is that the FiO2 is lowered by entrainment of room air through the exhalation ports. A minimum oxygen flow rate of 6 L/min must be used to maintain a higher oxygen concentration and prevent rebreathing of exhaled carbon dioxide (1).
Partial Rebreathing and Nonrebreathing Masks
A partial rebreathing mask consists of a simple face mask with a reservoir bag attached. With the simple mask, the patient’s inspired air is contaminated by the inhalation of room air through the exhalation ports and the rebreathing of oxygen-poor exhaled gases. The purpose of the reservoir bag is to reduce rebreathing. The first portion of exhaled gas enters
the reservoir bag, and because the source of this gas is primarily the airway rather than the lungs, it is not significantly involved with gas exchange and is therefore relatively oxygen rich (1). Remaining expired gases are vented through the exhalation ports. Assuming adequate oxygen flow into the reservoir bag (the bag should not fully deflate during inspiration), it should contain close to 100% oxygen. The patient therefore inhales oxygen flowing into the system from the oxygen source combined with the oxygen-rich gas that accumulates in the reservoir bag. However, the patient with sufficiently high inspiratory flow rates can still breathe atmospheric air that enters the system through the exhalation ports. Using a partial rebreathing mask with a flow rate of 10 to 12 L/min, an oxygen concentration of 50% to 60% can normally be achieved.
the reservoir bag, and because the source of this gas is primarily the airway rather than the lungs, it is not significantly involved with gas exchange and is therefore relatively oxygen rich (1). Remaining expired gases are vented through the exhalation ports. Assuming adequate oxygen flow into the reservoir bag (the bag should not fully deflate during inspiration), it should contain close to 100% oxygen. The patient therefore inhales oxygen flowing into the system from the oxygen source combined with the oxygen-rich gas that accumulates in the reservoir bag. However, the patient with sufficiently high inspiratory flow rates can still breathe atmospheric air that enters the system through the exhalation ports. Using a partial rebreathing mask with a flow rate of 10 to 12 L/min, an oxygen concentration of 50% to 60% can normally be achieved.
A nonrebreathing mask also contains a reservoir bag and functions in much the same manner as a simple mask but with a few important modifications. The nonrebreathing mask contains rubber seals that serve as one-way valves over the exhalation ports (Fig. 13.6). This allows egress of exhaled gases while preventing entrainment of room air into the mask during inhalation. However, safety standards mandate that only one of the two exhalation ports be sealed so that the patient can breathe room air should the oxygen source become disconnected from the reservoir bag. To further enhance oxygen delivery, a nonrebreathing system also has a second one-way valve located between the bag and mask. This valve diverts oxygen-poor exhaled gases away from the bag and thereby further reduces rebreathing. Assuming that oxygen flow is sufficient to prevent collapse of the reservoir bag on inspiration (10 to 12 L/min) and that the mask fits properly, an inspired oxygen concentration of over 95% can usually be achieved (1). Nonrebreathing delivery systems are also available for infants, with the only modification being that a small plastic tube, rather than a bag, serves as the reservoir.
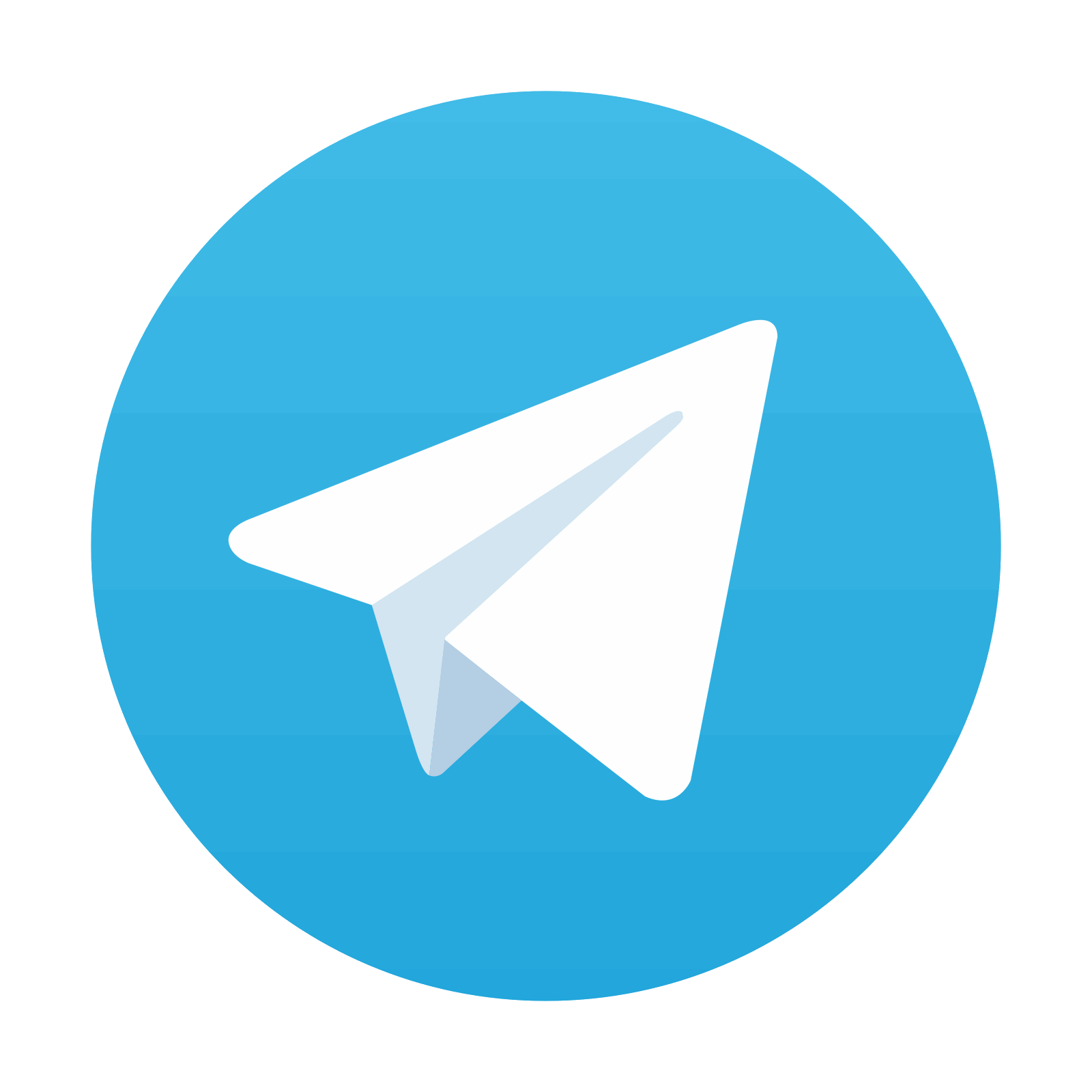
Stay updated, free articles. Join our Telegram channel

Full access? Get Clinical Tree
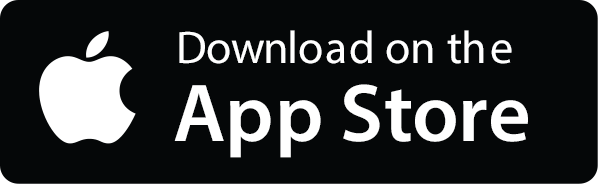
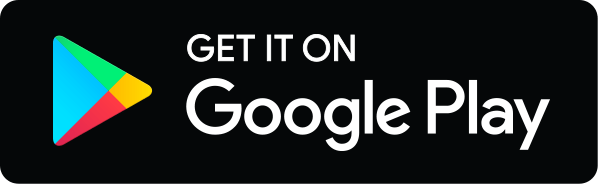
