Fig. 1
Normal adult CT (a) and 22-month-old MRI (b) sagittal landmarks. A nasion, B tuberculum sellae, C dorsum sellae, D basion, E opisthion, F posterior margin of the hard palate, G anterior arch of C1, H posterior arch of C1, I odontoid process, J body of C2
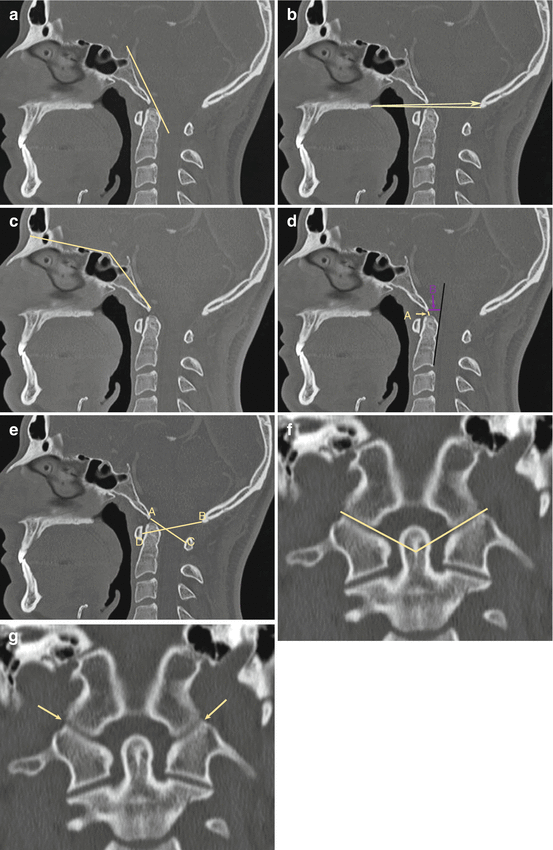
Fig. 2
Normal lines, angles, and interspaces. (a) Wackenheim’s clivus baseline; (b) Chamberlain’s line (drawn from the tip of the hard palate to the opisthion); McGregor’s line (drawn from the tip of the hard palate to the lowest occipital squamosal surface); (c) basal angle (minimum = 121°, maximum = 149°); (d) A: basion-dens interval (normal is <12 mm); B: basion-posterior axial interval (normal is <12 mm); (e) Powers’ ratio (normal AC/BD ratio is <1.0); (f) atlanto-occipital joint axis angle (normal 124–127°); (g) normal occipital-C1 joints (normal joint space is <2 mm)
Measurements seen on the midline sagittal image include Wackenheim’s line, Chamberlain’s line, McGregor’s line, the basal angle, the basion-dens distance, the basion-posterior-axial line interval, and the Powers’ ratio. Measurements assessed in the coronal plane include the atlanto-occipital joint axis angle and the widths of the occipital-C1 joints [56, 105]. Wackenheim’s clivus baseline is a line drawn along the posterior aspect of the clivus which should fall tangent to the posterior portion of the apex of the dens, irrespective of a flexed, extended, or neutral position. It is useful in assessing congenital or developmental conditions leading to basilar impression or basilar invagination, as any intersection of the line with the dens is abnormal, and in the traumatic setting, particularly if dynamic flexion-extension radiography is required [56, 105, 114] (Fig. 2a).
Chamberlain’s line [11] and McGregor’s line [66] are drawn from the tip of the hard palate to the opisthion and the lowest point of the occipital squamosal surface respectively (Fig. 2b); projection of the dens 5 mm above Chamberlain’s line and 7 mm above McGregor’s line is considered abnormal. These lines are useful in determining the degree of basilar impression or invagination. The basal angle can be measured as the intersection of the nasion-tuberculum sellae and tuberculum sellae-basion tangents [105]. The minimum angle is 121° and the maximum 149° (Fig. 2c).
Harris and colleagues defined the normal basion-dens interval (or measurement from the basion to the tip of the dens) on a lateral radiograph of the cervical spine, as less than 12 mm [40, 41] (Fig. 2d). Although this measurement was performed in adults and children over 13 years of age, it is generally used in our clinical practice for evaluation of lateral radiographs as well as midline sagittal cross-sectional images of the cervical spine in younger children. Harris et al. also defined the basion-posterior-axial interval or distance between the basion and a line drawn tangential to the posterior border of the dens as abnormal if more than 12 mm anterior and 0 mm posterior to the posterior axial line in children (12 mm anterior and 4 mm posterior in adults) [41] (Fig. 2d).
The Powers’ ratio is defined as the ratio of the distance from the basion to the posterior arch of the atlas and the distance from the opisthion to the anterior arch of the atlas [92] (Fig. 2e). A ratio of greater than 1.0 is considered abnormal. While the Powers’ ratio may be used for assessing the presence of anterior atlanto-occipital dissociation in the traumatic setting, it is unable to diagnose the rare posterior variant [56].
In the coronal plane, the tangents drawn parallel to the atlanto-occipital joints should intersect at the center of the odontoid process if the condyles are symmetric (Fig. 2f). The average angle measures between 124° and 127° [113], and a more obtuse angle suggests occipito-condylar hypoplasia.
Pang and colleagues determined that the widths of the normal occipital-C1 joints should be less than 2 mm and that there is strong left-right symmetry [90, 91] (Fig. 2f). Occipital-C1 joint distances greater than 4 mm were considered strongly suggestive of atlanto-occipital dislocation [91].
Trauma
The four major injury patterns of the pediatric craniocervical junction include longitudinal subluxation with failure of the major unit stabilizers across the occiput-C2 unit more commonly referred to as atlanto-occipital subluxation, translational atlantoaxial subluxation, atlantoaxial rotatory fixation, and fractures [56].
Evaluation of cervical spine trauma typically begins with plain radiographs (Fig. 3). It is important to note, however, that radiographic evaluation has significant limitations and low sensitivity. Woodring and Lee found that “trauma series” cervical spine radiographs including lateral, anteroposterior, and odontoid views failed to detect 61 % of fractures and 36 % of subluxations in a series of adult patients [119]. Therefore, further evaluation with high-resolution CT and MRI in many cases is necessary (Fig. 4).
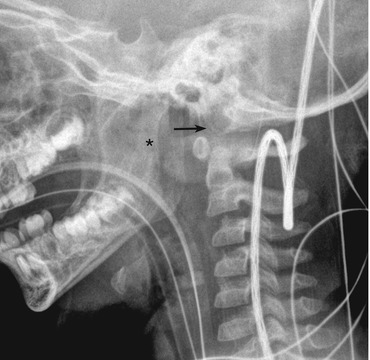
Fig. 3
Atlanto-occipital dislocation. Note the increased basion-dens interval and marked nasopharyngeal soft tissue prominence
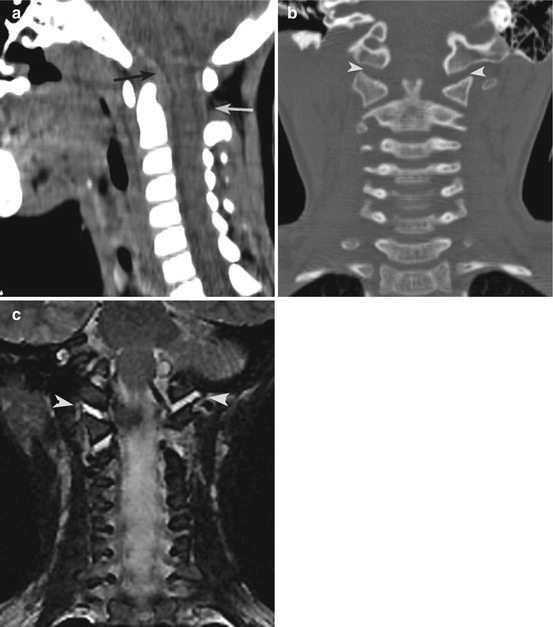
Fig. 4
Atlanto-occipital dislocation. The basion-dens interval in this child was 9 mm (normal <12 mm). However, the midline sagittal CT (a) shows a retrodental epidural hematoma (black arrow) and an increased posterior interspace between C1 and C2 (grey arrow). Coronal CT (b) shows bilaterally widened atlanto-occipital joints (grey arrowheads) to 4.2 mm (normal <2 mm). Coronal MRI (c) confirms injury to the atlanto-occipital joints evidenced by high T2 fluid signal (grey arrowheads). Note also the abnormal T2 signal in the visualized right atlantoaxial joint
The drawbacks of CT include poorer evaluation of ligamentous structures. And while the clinical relevance of subtle MRI findings of slightly increased high signal in the transverse or alar ligaments with a normal CT scan is not yet well understood, a study in a pediatric imaging center showed that the use of MR imaging in obtunded patients resulted in decreased time to C-spine clearance with resultant decreased ICU and hospital stays, without a significant increase in diagnosis of injuries [32]. Therefore both CT and MRI are useful adjuncts in the setting of pediatric cervical trauma.
Atlanto-occipital subluxation results from disruption of the ligaments connecting the occiput to the atlas and axis. It is often a result of high-speed motor vehicle accidents or pedestrian accidents. Children, particularly those younger than ten, are predisposed to this injury due to a greater head weight to body weight ratio and smaller, more horizontally oriented atlanto-occipital joints [56] (Figs. 3 and 4).
In a review of 16 patients aged 3–17 years of age who satisfied one or more known radiographic criteria or presented with well-known clinical and MRI features of atlanto-occipital dissociation, Pang and colleagues compared measurements of the occipital condyle-C1 interval (taking an abnormal value to be greater than 4 mm) with five radiographic criteria including a dens-basion interval of more than 12 mm, a Powers’ ratio of greater than 1, Harris’ basion-posterior-axial interval of greater than 12 mm or less than 0 mm, and an abnormal Sun’s interspinous ratio where the ratio of the interspinous distances between C1–C2 and C2–C3 is greater than 2.5 using high-resolution CT. They found that only the occipital condyle-C1 interval joint distance had a sensitivity and specificity of 100 % for detecting atlanto-occipital dislocations with the sensitivities of the other tests ranging from 25 % for the Sun’s interspinous ratio (C1–C2/C2–C3 distance, should be less than 2.5) to 50 % for an abnormal dens-basion interval and false-positive rates ranging from 10 % for the Powers’ ratio to 60 % for Sun’s interspinous ratio [90, 91] (Fig. 4).
Additional CT and MRI findings of atlanto-occipital dislocation include extra-axial and perimedullary blood, tectorial membrane rupture, alar ligament rupture, blood and edema in between the dens and clivus, blood in the predental soft tissues, edema in the C1–C2 prevertebral fascia, and edema in the posterior soft tissue between the occiput and C2 [90, 91]. Severe cases may result in transection of the brainstem and cervicomedullary junction or acute and delayed hydrocephalus with syringomyelia [74, 95].
Translational atlantoaxial subluxation without fracture occurs due to loss of the transverse ligament resulting in compression of the spinal cord between the odontoid and the posterior arch of the atlas. When traumatic in nature, this usually results from a flexion injury incurred during violent trauma [62]. (Nontraumatic causes due to ligamentous laxity of the transverse ligament or dens hypoplasia include Down syndrome, Klippel-Feil syndrome, and skeletal dysplasias which will be discussed further in the chapter [56].)
Dickman and colleagues differentiated injuries of the transverse ligament into two types with differing clinical consequences. Type I injuries disrupt the ligament substance in its midportion (IA) or at its periosteal insertion (IB); these injuries were found to necessitate internal fixation. Type II injuries disconnect an intact transverse ligament from the C1 lateral mass involving comminution of the C1 lateral mass (IIA) or avulsion of the tubercle from an intact lateral mass (IIB) and had a 74 % recovery rate with nonoperative treatment [24].
An atlantodental distance of greater than 4 mm in children is considered abnormal on plain lateral radiographs [24, 56]. CT allows for detailed evaluation of fractures of the atlas and avulsions of the C1 tubercles. MRI findings of transverse ligament disruption include loss of continuity of the ligament, high-signal intensity in the ligament, or blood separating the ligament from its insertion [24]. Additional imaging features on CT and MRI include widening and edema in the retropharyngeal soft tissues.
Atlantoaxial rotatory subluxation refers to a range of abnormalities involving the atlas and axis from mild abnormalities of rotation to complete fixation. Trauma is responsible for about 30 % of cases and is usually minor [55, 56]. Other causes include head and neck infections, surgery, and neoplasms. Pang and Li have described the normal rotational motion of C1 with respect to C2 [86, 87]. They suggest that atlantoaxial rotatory subluxation is a “stickiness,” or resistance to turning of C1 with respect to C2, and therefore cannot be evaluated with a static image alone. They describe the normal physiologic movements of C1 and C2 as follows: crossover of C1 with respect to C2 occurs at O° or with the nose pointing forward, for the first 23° of rotation C1 moves in isolation with respect to C2 mainly due to the horizontal orientation of the C1–C2 facets, and at about 23° of rotation tightening of the alar ligaments results in pull and rotation of the dens, which for the next 42° rotates nonlinearly with respect to C1 approaching its speed. At about 65° away from the neutral position, the two vertebrae rotate in unison [86, 87]. The movements on the right and left side of the head are mirror images of each other. Having described the normal physiology of the atlantoaxial joints, the group went on to develop a four-point system which requires CT imaging in three planes: the plane of rotation most comfortable to the patient, the neutral position in which the head is perpendicular to the CT table, and head rotation as far as possible to the contralateral side. Grade I injuries are those which show a locked C1–C2 articulation. Grade 2 injuries have reduction of the C1–C2 angle with forced correction, but no crossing of C1 with respect to C2. Grade 3 injuries have C1–C2 crossover only when the head is turned far to the opposite side, and not in the expected neutral position. A fourth type of injury shows dynamics in between the range of normal and grade 3 injuries and has been termed the “diagnostic grey zone” [86–88].
A fracture at the craniocervical junction specific to children involves the dens synchondrosis (Fig. 5). This synchondrosis normally fuses by 5–7 years of age but may remain open into adolescence [2]. True fractures will result in an anteriorly displaced and angulated odontoid process with a preserved atlantodental interval [16, 29]. However, nondisplaced fractures of the synchondrosis (odontoid epiphysiolysis) may be difficult to detect. According to one series, nondisplaced fractures occur less than 10 % of the time. The same series showed that 53 % of displaced or nondisplaced odontoid synchondrosis fractures have been shown to be associated with spinal cord injury, usually at the cervicothoracic junction. It is therefore important to reevaluate the synchondrosis for subtle widening on CT in cases of seemingly isolated spinal cord injury [29]. Contributory MRI findings include increased signal in the synchondrosis and prevertebral soft tissue changes at the C1–C2 level (Fig. 5d).
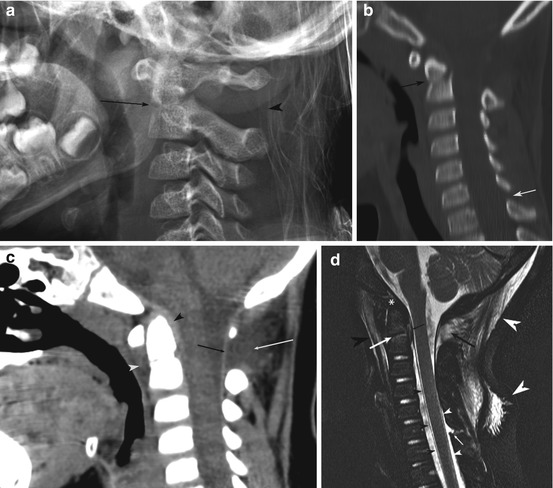
Fig. 5
A 6-year-old male with odontoid synchondrosis fracture. (a) Lateral radiograph demonstrates mild widening of the odontoid synchondrosis and of the C1–C2 spinous interspace. (b) Sagittal CT image with bone windows just off midline demonstrates angulation at the occipital synchondrosis (black arrow); subtle widening of the C5–C6 spinous processes (grey arrow). (c) Midsagittal CT image with soft tissue windows demonstrates small retrodental epidural hematoma (black arrowhead), widening of the spinous processes interspace (black arrow), retropharyngeal tissue edema (grey arrowhead), posterior soft tissue edema/hematoma (grey arrow). (d) MRI in the same patient shows the extent of injuries including a synchondrosis fracture and edema (grey arrow), C1–C2 interspinous ligament tear (black arrow), anterior and posterior soft tissue edema (large black and grey arrowheads respectively), small retrodental epidural hematoma (small black arrow), C5–C6 interspinous ligament tear and suspected dural tear (small grey arrow), lifting off of the anterior (small black arrowheads) and posterior dura (small grey arrowheads), and extensive CSF isointense epidural collection
True odontoid adult type fractures may occur in older children [56].
The neurocentral synchondrosis, which closes between 3 and 6 years of age, is another radiologic pitfall, and its presence on oblique radiographs may be misinterpreted as a pars interarticularis or hangman’s fracture [65, 78, 107]. Note should be made that true fractures through this synchondrosis have been reported and are analogous to odontoid epiphysiolysis. In one of the cases reported, initial radiographs, CT, and bone scan were attributed to congenital defects with subsequent subluxation at the pedicular level uncovering the underlying fracture [38].
True fractures of the pars interarticularis are generally classified according to the Effendi classification based on the degree of displacement of the anterior and posterior C2 fragments [26]. Type I fractures are either hairline fractures or have minimal anterior displacement or angulation of the axis. Fractures with greater than 3 mm of anterior translation of C2 with respect to C3 are considered type II. Type III fractures are the rarest and involve a displaced anterior fracture fragment with dislocated or subluxed unilateral or bilateral facet joints [26].
Craniovertebral Junction Bone Anomalies
The subsequently described anomalies of the craniovertebral junction may occur in isolation or as part of congenital syndromes; the latter will be described in greater detail further in the chapter.
Some authors have separated anomalies of the craniovertebral junction into those present at birth and those which, although resulting from an underlying faulty embryology, become symptomatic in childhood or early adulthood. The former group includes entities such as proatlas segmentation failures, congenital basilar impression, basilar invagination, atlas assimilation, condylar hypoplasia, absent components of the atlas, spondylolysis, and segmentation failures resulting in hemivertebrae. The second group includes entities such as acquired basilar impression, rotatory dislocation in the nontraumatic setting, os odontoideum, syndromic abnormalities, and osteogenesis imperfecta [71]. Note should be made of the fact that implied meaning of the terms “basilar invagination” and “basilar impression” has varied in literature, with certain authors reserving the term “basilar impression’ for congenital entities and basilar invagination for acquired entities [105] and others differentiating primary or congenital basilar impression from secondary or acquired basilar impression [66, 89].
For the purposes of the following discussion, we will use the term “congenital basilar impression” to define occipital dysplasia which results in a shallow occipital “box” resulting in a drop of posterior fossa contents and an abnormally high position of the vertebral column with respect to the foramen magnum [89]. “Acquired basilar impression” involves similar morphologic abnormalities but is due to softening conditions of the skull base such as osteogenesis imperfecta, hyperparathyroidism, rickets, and Paget’s disease [105]. The term “basilar invagination” on the other hand is reserved for the invagination of the opisthion into the cranial aperture, which may occur as a result of the posterior form of congenital basilar impression in which the exoccipital bones are elevated into the foramen magnum, without lordosis or retroflexion of the dens [89].
Congenital abnormalities may be further subdivided into those that involve the central pivot of the craniocervical junction and those that involve the two-ringed structures that surround this pivot, reflecting the previously discussed embryologic fates of the axial and lateral components of the proatlas and first two cervical sclerotome segments. The structures which form the central pillar are the dens, C2 vertebral body, and the clivus which is embryologically and functionally in vertical linearity with the central pillar. As already discussed, these structures arise from the centra of the proatlas and first two cervical sclerotomes. Structures arising from the neural arches of the same sclerotomes, and hypocentra of the proatlas and first cervical sclerotome, develop into the ringed structures surrounding the pivot and include the lateral portion of the clivus, the exoccipital bones and the opisthion, the anterior arches, posterior arches, and lateral masses of the atlantal ring [89]. Malformations of the central pillar include basiocciput hypoplasia, congenital basilar impression, basilar invagination, platybasia, basilar kyphosis, os avis, aplasia or hypoplasia of the dens, retroflexed dens, disturbance of the odontoid synchondroses, and bifid dens. Malformations of the surrounding rings include third occipital condyle, preoccipital arch, hyperplasia or hypoplasia of the occipital condyles, atlas assimilation, and aplasia and hypoplasia of the anterior or posterior C1 arches [89]. Appreciating these two ontogenetic categories is helpful in understanding the frequent associations seen between clival and odontoid craniocervical junction abnormalities such as platybasia, basilar kyphosis, retroflexed dens, and cerebellar ectopia, which affect both the clival and odontoid primordia. Note is made of the fact, however, that, as in basioccipital dysplasia which is often associated with occipital condyle hypoplasia, defects of the axial and ring components of the craniocervical junction are not mutually exclusive and not infrequently occur together [89, 105].
The following is a discussion of possible congenital anomalies of the craniocervical junction with reference to this ontogenetic classification.
Basioccipital Dysgenesis
Congenital basilar impression results from hypoplasia of the basiocciput and exocciput with obligatory constriction of the supraoccipital bone, the enchondral growth of which depends on the chondrocranial molds of the other two parts [89]. On imaging studies basilar impression is defined as elevation of the tip of the odontoid 5 mm above Chamberlain’s line and 7 mm above McGregor’s line (Fig. 6). Because this occipital hypoplasia results in a small posterior fossa, it is often associated with a Chiari I deformity of the cerebellum.
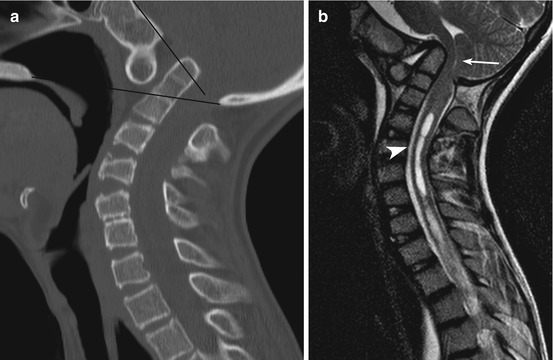
Fig. 6
Midline sagittal CT (a) and MRI (b) in a 14-year-old male with VACTERL syndrome and congenital basilar impression. The dens projects 18 mm above Chamberlain’s line and abnormally intersects Wackenheim’s clivus baseline. This results in severe compression of the cervicomedullary junction (grey arrow) and cervical syringohydromyelia (white arrowhead). There is fusion of the occipital ring to C1. A rudimentary disc space is seen at C5–C6
Studies have shown that patients with basilar impression have significantly shorter clivus lengths than control groups [84]. Normally, the basioccipital clivus measures more than 3.2 cm from the spheno-occipital synchondrosis in adults and should terminate at the cervicomedullary junction [84, 89, 94]. Anterior congenital basilar impression results from changes in the axial occipital sclerotomes and can lead to platybasia, often accompanied by basiocciput hypoplasia. (As previously described platybasia is defined as flattening of the basal angle to greater than 150°.) This results in elevation of the basion and an upward tilting of the foramen magnum and condyle-atlas articulations. The lordotic deformity of the skull base often results in a compensatory lordotic curve of the dens, which may cause significant impression on the adjacent brainstem (Fig. 6).
Posterior basilar impression involves the elevation of the exoccipital bones with secondary elevation of the dens towards the cranial cavity without retroflexion. It is this type of basilar impression that often results in basilar invagination or the projection of the opisthion into the cranial aperture [89].
Abnormalities of the Dens
The dens forms from the centra of the proatlas and first spinal sclerotome which fuse to the centrum of the second spinal sclerotome [71]. Therefore there are two embryologic dental segments, the apical and basal, both of which may fail to form. The most common variety of dens agenesis is absence of only the apical segment resulting in a short dens, but with adequate support for the cruciate ligament, which prevents instability. On the other hand, agenesis of both dental segments or only the basal segment will result in instability [89]; furthermore, lesions resulting in instability of this region have been associated with irregularities of the vertebral arteries leading to posterior fossa infarcts [4, 76]. Because sporadic congenital defects in the primordia of two centra are unusual, complete agenesis of both dental components results rather from collagenopathy syndromes such as spondyloepiphyseal and spondylometaphyseal dysplasias or mucopolysaccharidoses where there is a failure of ossification rather than of chondrification [89].
The os odontoideum is defined as an independent bone in the place of the dens, cranial to the axis vertebra (Fig. 7). Controversy exists in the literature as to whether the etiology of this condition is traumatic, the result of a non-united odontoid fracture, or developmental [18, 43, 45, 47, 89]. In support of a post-traumatic etiology, in one series of children aged 1–4 years, several cases of os odontoideum were identified in patients who had previously had radiographically documented normal odontoid processes [70]. Proponents of the traumatic theory also suggest that often there is a small bony cupola attached to the base of the dens that is thought to represent the fractured base of the dens [70, 71, 105]. On the other hand, os odontoideum has been identified in families, identical twins, and children with epiphyseal dysplasias suggesting a developmental nature [53, 77, 80]. Some authors point out that the current literature likely includes a heterogenous group of disorders, arising from both post-traumatic and developmental etiologies, discussed under the umbrella term of os odontoideum [89].
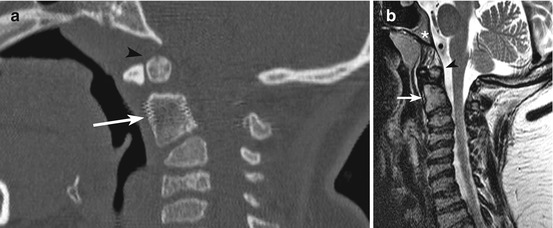
Fig. 7
Os odontoideum in a child with Kniest dysplasia. Midline sagittal CT (a) and T2-weighted MRI (b) show hypoplasia of the dens (grey arrows) and an os odontoideum (black arrowheads). There is hypoplasia of the clivus (white asterisk). Note also the mild cervical kyphosis centered at C3–C4, platyspondyly, and undulating vertebral body endplates
Irrespective of etiology, however, the clinical implications of os odontoidea are similar. Because of the gap between the os and the body of C2, there may be incompetence of the cruciate ligament resulting in atlantoaxial instability. While patients may be asymptomatic, symptoms can occur and range from neck pain, paretic episodes, and brainstem strokes resulting from vertebral artery stretching to compression of the cervicomedullary junction [4, 76, 105, 109].
An abnormality separate from the os odontoideum is the ossiculum terminale, which is felt to represent nonunion of the apical-dental segment derived from the centrum of the proatlas [21, 22]. The abnormality may be misinterpreted as a fracture, although its well corticated border should point to its congenital nature. As there is enough room on the base of the dens for the insertion of the cruciate ligament, instability has only been seen in cases where there is hypoplasia of the basal dental segment [89, 105].
Disturbance of the Craniovertebral Ring Structures
In morphometric studies on 404 occipital condyles, Naderi and colleagues have determined that normal condylar length is 23.4 mm, the normal condylar width is 10.6 mm, and the normal condylar height is 9.2 mm [81].
Hyperplasia of the proatlas hypocentrum is a rare anatomic abnormality which can result in a bony spur that projects obliquely backwards from the occipital bone. This bony spur has the potential of forming synovial joints with the anterior arch of C1 and has therefore been termed the third occipital condyle. There are reports of this abnormality causing significant symptoms resulting from compression and myelopathy at the craniocervical junction [58, 73]. Similarly, hyperplasia of the lateral sclerotome of the proatlas leads to hyperplastic and overgrown occipital condyles. Bilateral hyperplasia of the occipital condyles leads to early symptoms, generally presenting in the first two decades of life [73].
Hypoplasia of the occipital condyles often results in basilar impression and has been discussed in the section describing anomalies of the basiocciput.
Studies have shown that that there is about a 33–39 % association between abnormalities of proatlas segmentation and Chiari I deformity [71, 73] (Fig. 8).
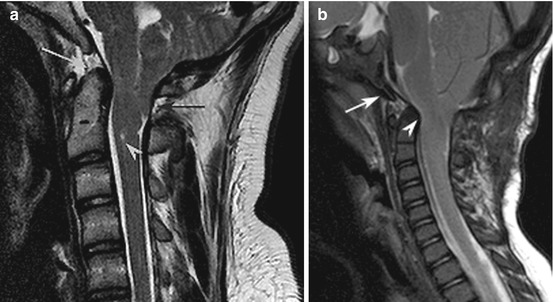
Fig. 8
Chiari I malformation in two different patients. (a) Midline sagittal T2-weighted MRI shows cerebellar tonsillar descent to below the level of the posterior C1 arch (black arrow) resulting in crowding of structures at the cervicomedullary junction. There is only very minimal retroversion of the dens (grey arrow). There is a tiny syrinx of the upper cervical cord (white arrowhead). (b) Midline sagittal T2-weighted MRI shows cerebellar tonsillar descent to the level of the posterior C1 arch. There is hypoplasia of the clivus (white arrow) which terminates at the mid-medullary level and mild retroversion of the odontoid which results in medullary compression and crowding at the foramen magnum
Atlas Anomalies
One of the most common anomalies of the craniocervical junction is assimilation of the atlas, which has been reported to have a prevalence rate of 0.08–2.8 % in the general population [12, 61] (Fig. 6). Assimilation can occur at the site of the anterior or posterior arches or lateral masses, or any combination of the above. While symptoms usually present in adulthood, a recent study of pediatric patients revealed that symptoms can occur in children and range from neck pain, torticollis, and motor and sensory symptoms to incontinence [34]. Assimilation of the atlas has been associated with increase in the atlantodental interval in as many as 57 % of patients [34], fusions of other cervical vertebrae, basilar invagination, os odontoideum, Chiari I deformity, and spinal canal encroachment [3, 28, 34, 68, 69].
Assimilation of the atlas was also associated with failures of the posterior arch of the atlas to form, arising from malformations from the lateral C1 sclerotome. Complete or partial agenesis of the posterior arch of the atlas can also occur in isolation and is ten times more common than abnormalities of the anterior arch, likely due to a developmental failure of chondrogenesis. In general, isolated agenesis or aplasia of the posterior arch does not affect the dental pivot and cruciate ligament and is more stable than its anterior counterpart [89]. However there are several reports of posterior arch anomalies causing a Lhermitte’s sign with neck extension, transient quadriparesis, and chronic neck pain [19, 54, 98, 100, 102].
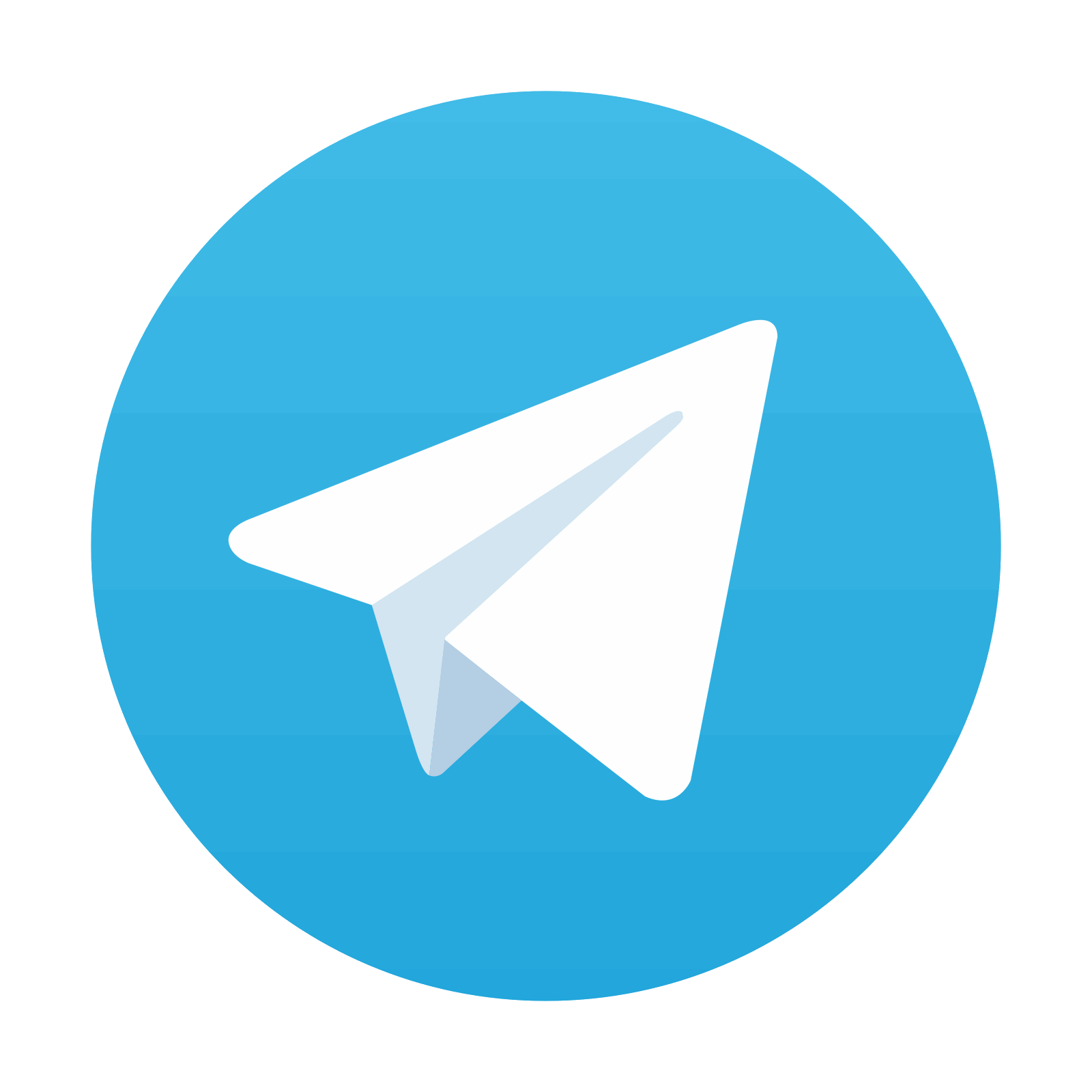
Stay updated, free articles. Join our Telegram channel

Full access? Get Clinical Tree
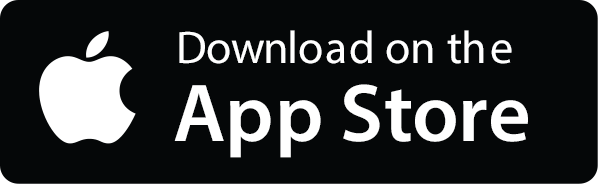
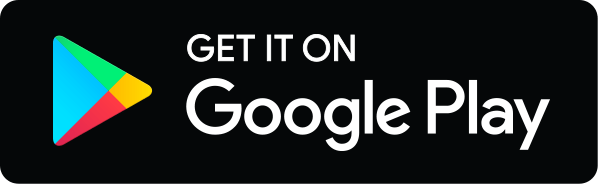