Obstructive Sleep Apnea Syndrome
Pathophysiology and Clinical Characteristics
Definitions
Obstructive sleep apnea syndrome (OSA) is defined by the American Thoracic Society (ATS)1 as ‘a disorder of breathing during sleep characterized by prolonged partial upper airway obstruction and/or intermittent complete obstruction (obstructive apnea) that disrupts normal ventilation during sleep and normal sleep patterns.’
Sleep apnea in children was well described as early as 1976 by Guilleminault et al.2,3 Sleep-disordered breathing occurs in children of all ages, from neonates to adolescents, with a peak prevalence between 2 and 8 years of age. Habitual snoring occurs in 5% to 12% of children; the prevalence of SDB is estimated at 4% to 11%,4 and the prevalence of OSA is estimated at 1% to 4%.5–7 SDB is more common among boys and among children who are heavier.4
Pathophysiology
Transition to the sleep state normally results in elevation of upper airway resistance, mainly due to reduction in airway diameter, resulting from the reduced tone of the pharyngeal dilator and constrictor muscles. Normal sleep is characterized by relative hypoxemia and hypercapnia, particularly during REM sleep, when compared to wakefulness. This normal phenomenon is magnified in patients with underlying pulmonary (such as nocturnal asthma) or upper airway (such as OSA) disease. This is the result of the following physiologic factors:8
• Functional residual capacity is reduced during sleep mainly during rapid eye movement (REM) sleep, leading to more rapid hypoxemia with apnea. This is due to decreased intercostal and upper airway muscle tone, particularly during REM sleep.
• Central ventilatory drive regulating upper airway tone and ventilatory response to hypoxia and hypercapnia decrease during normal sleep.
• Upper airway resistance increases during sleep, as a result of the decrease in upper airway tone. Small increases in upper airway resistance can have a significant impact on breathing.
Children with OSA tend to have a narrow upper airway. The patency of the upper airway is determined by both anatomic and physiologic factors. The site of obstruction in children tends to be more distal than in adults, typically involving the oropharynx and hypopharynx. Magnetic resonance imaging of upper airway structures confirmed that children with OSA have significantly larger adenoids and tonsils than those of controls.9 Arens et al. described the region in which these two lymphoid tissues overlap to constitute the site with the smallest airway diameter as the ‘overlap region.’ The upper airway is rich in neural receptors, which play a part in controlling baseline muscle tone. Any loss in this tone, as occurs at sleep onset, contributes to increased pharyngeal resistance. During inspiration, many dilator upper airway muscles exhibit phasic respiratory activity. This phasic activation of the muscles of the nose, pharynx, and larynx occurs before diaphragm and intercostal muscle activity, suggesting pre-activation of the upper airway muscles in preparation for the development of negative pressure.
The Starling Resistor Theory
Under conditions of negative inter-luminal pressure, collapse of the upper airway occurs variably during inspiration. The pattern of flow on the driving pressure occurs in ‘Starling resistors,’ which are a specific model of ‘collapsible tube’ behavior (Figure 27-1).8 The model predicts that, under conditions of flow limitation, maximal inspiratory airflow is determined by the pressure changes upstream (nasal) from the collapsible site of the upper airway and is independent of the downstream (hypopharyngeal and tracheal) pressure generated by the diaphragm. The upper airway can be represented as a tube with a collapsible segment, the resistance of which is zero. The segments upstream and downstream from the collapsible segment each have a fixed diameter, resistance, and pressure. The upstream pressure can be approximated by the nasal pressure and the downstream pressure can be approximated by the hypopharyngeal pressure. In this model of the upper airway, inflow pressure at the airway opening (the nares) is atmospheric, and downstream pressure is equal to tracheal pressure. Collapse occurs when the pressure surrounding the collapsible segment of the upper airway (critical tissue pressure = Pcrit) becomes greater than the pressure within the collapsible segment of the airway. In normal subjects with low upstream resistance or sub-atmospheric critical tissue pressure, hypopharyngeal pressure never drops to critical pressure; thus, airflow is not limited and is largely determined by negative tracheal pressure. However, if hypopharyngeal pressure falls below critical pressure, maximal inspiratory flow reaches its maximum limitation, and becomes independent of downstream pressure swings. Under these circumstances, nasal resistance and critical pressure determine maximal inspiratory flow as described by the following equation:
Figure 27-1 Starling resistor model of the upper airway.
The upper airway is represented as a tube with a collapsible segment. The upstream (nasal) and downstream (trachea) segments have fixed diameters and resistance (RUS, RDS) and pressures (PUS, PDS). Collapse occurs when the pressure surrounding the airway (Pcrit) is greater than that within the airway. Redrawn with permission from Marcus CL. Pathophysiology of OSAS in children. In: Loughlin GM, Carrol JL, Marcus CL editors. Sleep and breathing in children, A developmental approach. Marcel Dekker Inc. NY; 2000. p. 601–24.
where VImax is maximal inspiratory flow; PN is nasal pressure; Pcrit is critical tissue pressure; RN is nasal resistance. Airflow will become zero (i.e., the airway will occlude) when nasal pressure falls below critical pressure.
Normal infants and children have a narrower upper airway than adults. Nevertheless, they snore less and have fewer obstructive apneas. This could be due to either structural differences or differences in neuromotor regulation of the upper airway. It is difficult to determine critical pressure in normal children, as their upper airway is very resistant to collapse. Marcus et al. have shown that Pcrit in children correlated with the severity of OSA.10
The upper airway in children with OSA is more collapsible compared with control subjects during wakefulness and sleep, and under general anesthesia. Several mechanisms might lead to more airway collapse in these subjects, including: decreased motor tone, increased airway compliance, and excessive inspiratory driving pressures caused by proximal airway narrowing. This increased propensity of the airway to collapse should be reflected by increased motion of airway boundary during respiration as negative upper airway intraluminal pressure is increased. Using respiratory-gated magnetic resonance imaging to quantify changes in shape and airway cross-section area during tidal breathing in children with OSA, compared with control subjects, it has been shown that fluctuations in airway area during tidal breathing are significantly greater in subjects with OSA compared with control subjects.11
Resistive pressure loading is a probable explanation, although increased airway compliance may be a contributing factor. Studies using denervated upper airways have shown that when upper airway muscle function is decreased or absent, the airway is more prone to collapse.12,13 Children have active upper airway dynamic responses to both negative pressure pulses and hypercapnia during sleep.14 Normal children compensate for their smaller upper airway by increasing the ventilatory drive to their upper airway muscles. This compensatory mechanism may be absent or diminished in children with OSA.
The Etiology of OSA
The etiology of OSA in children is multifactorial, and is probably a combination of abnormal airway structure, decreased neuromuscular control, and other factors such as genetic, hormonal, and metabolic (Figure 27-2).
Figure 27-2 Obstructive sleep apnea syndrome (OSA) in children may result from a combination of factors. With permission from Marcus CL. Pathophysiology of OSAS in children. In: Loughlin GM, Carrol JL, Marcus CL editors. Sleep and breathing in children, A developmental approach. Marcel Dekker Inc. NY; 2000. p. 601–24.
Pierre Robin sequence, consisting of micrognathia and posterior displacement of the tongue and soft palate, is frequently associated with sleep-disordered breathing, presenting in early infancy, in the first few weeks of life.
Midfacial hypoplasia syndromes include Crouzon syndrome, Apert syndrome, and Pfeiffer syndrome.
Children with Down syndrome frequently present OSA because of their craniofacial structure: maxillary hypoplasia and small nose with low nasal bridge. In addition, recurrent upper airway infections result in adenotonsillar hypertrophy.15 Children with Arnold–Chiari malformation may be at risk for developing both central and obstructive sleep apnea due to brainstem compression affecting respiratory drive, and/or activation of the pharyngeal and laryngeal muscles that are enervated by the ninth and tenth cranial nerves.
The high prevalence of habitual snoring in first-degree relatives of children with OSA, and documented familial aggregation of OSA, suggest a familial predisposition to this condition.16,17 African-Americans are at greater risk than Caucasians when controlling for age, sex, and BMI, indicating ethnicity as another risk factor for OSA.18
Prematurity was also identified as a risk factor for OSA.5,19 Former preterm infants are at increased risk to develop OSA in early infancy, possibly due to facial asymmetry, greater incidence of muscle hypotonia, or nasal obstruction following prolonged intubation.20
Adenotonsillar hypertrophy is the most common etiology of OSA in children, making adenotonsillectomy the first-line treatment at this age. The fact that most children significantly improve following surgery21 proves their role in the etiology of OSA in children. However, the fact that cure is not achieved in all children22 indicates that other factors are involved. The tonsils and adenoids increase in size from birth to about 12 years of age, and are largest in relation to the underlying upper airway size between 3 and 6 years of age.23 The correlation between adenotonsillar size and OSA is not strong. While the severity of obstructive events was related to the size of the adenoids in one study,24 other studies have failed to show a correlation between adenotonsillar size and OSA severity.25
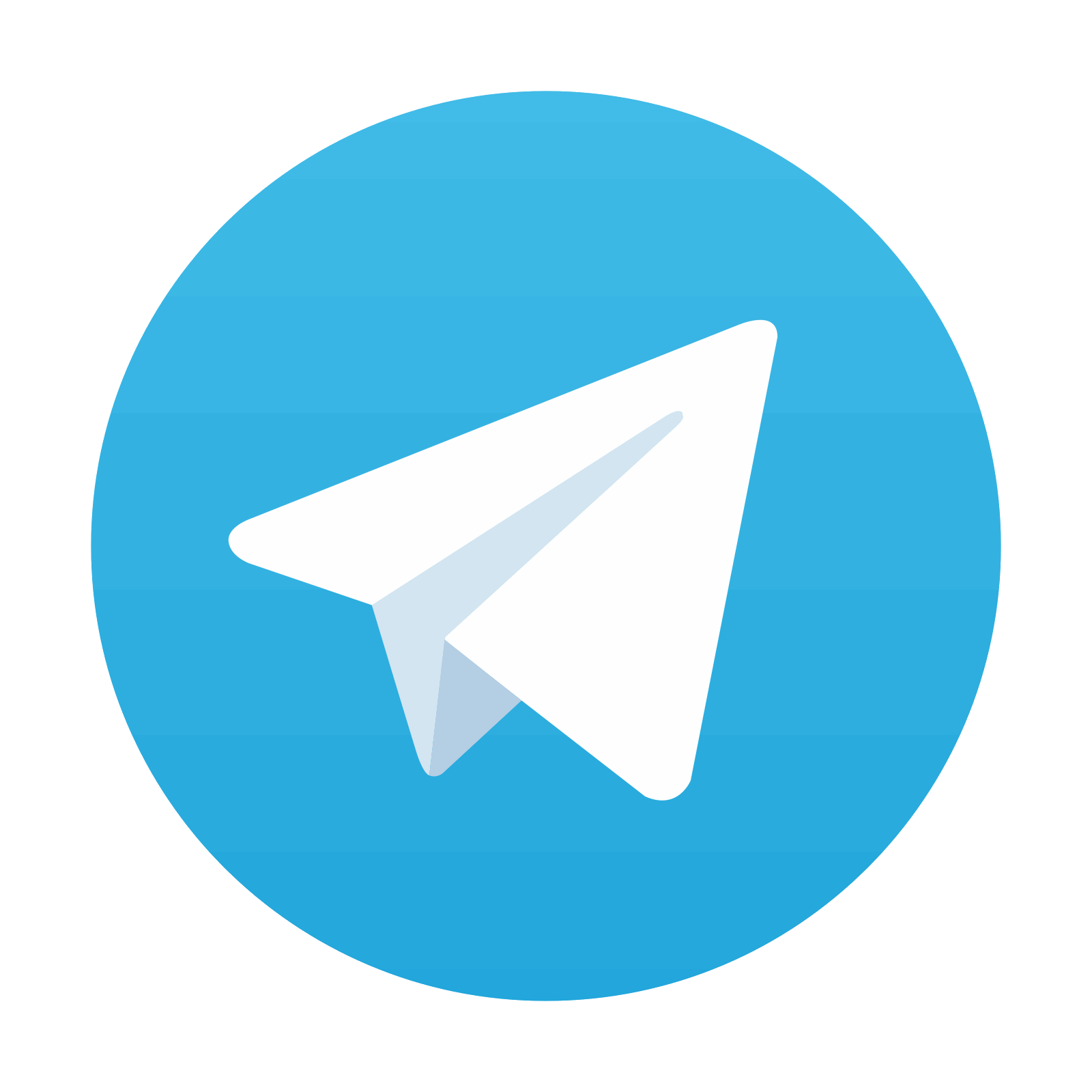