Fig. 40.1
Nasal respiratory support via nasal prongs
40.1.1 Initiating Nasal Respiratory Support (NRS)
40.1.1.1 Nasal Continuous Positive Airway Pressure (NCPAP)
Nasal continuous positive airway pressure (NCPAP) goal in infants with respiratory distress syndrome (RDS) is to maintain lung recruitment or functional residual capacity (FRC). The level of pressure should achieve this goal without compromising circulatory or ventilatory function (Yu and Rolfe 1977). A pressure of 4–6 cm H2O usually will be adequate (Kugelman et al. 2007; Barrington et al. 2001; Khalaf et al. 2001; Friedlich et al. 1999). Meta-analyses of CPAP studies suggest that a pressure of at least 5 cm H2O is needed to provide benefit over ambient oxygen (Moretti et al. 1999). Higher pressures up to 8 cm H2O were used by some investigators, but at least in one study, the rate of pneumothorax using such pressure was relatively high (Morley et al. 2008).
Practical comments regarding different NCPAP devices will be discussed in Sect. 40.1.5.
40.1.1.2 Nasal Intermittent Mandatory Ventilation (NIMV)
The ventilator settings used for NIMV or nasal synchronized intermittent mandatory ventilation (NSIMV) were not similar in all studies. The effects of different initial settings on the success of NIMV and of manipulating the settings on clinical status have not been investigated.
No study has investigated the optimal level of positive end-expiratory pressure (PEEP) during NIMV. It is probably adequate to adopt the recommendations for NCPAP, as CPAP to maintain FRC in treating RDS is the basis for NRS and the intermittent mandatory ventilations used in NIMV are just added on top of the CPAP.
No studies have investigated optimal peak inspiratory pressure (PIP) during NIMV. Some NIMV studies used a PIP similar to that used during ventilation (Friedlich et al. 1999; Moretti et al. 1999), whereas others used pressures 2–4 cm H2O higher than pre-extubation PIP (Khalaf et al. 2001; Santin et al. 2004). One study used enough pressure “to see the chest rise” (Lin et al. 1998), and others chose specific target pressures (16–20 cm H2O) (Barrington et al. 2001; Ryan et al. 1989). Ryan et al. (1989) noted that despite a set pressure of 20 cm H2O, the pressure generated at the proximal end of the nasal prongs was highly variable (range 8–21 cm H2O; mean 10 cm H2O).
The optimal rate of inflation during NIMV has not been investigated. A range of rates have been used, mainly 10–25/min. There were reports on the use of assist control mode, in which every infant-initiated breath is supported by a ventilator inflation (Ali et al. 2007). No studies have investigated the flow to use in the circuit during NIMV. The circuit flow, and the leak from the device, will influence the PIP achieved during each inflation.
We suggest choosing the initial ventilator settings according to the indication for NIMV. For the initial treatment of RDS (Kugelman et al. 2007), NIMV was set at a rate of 12–30 breaths per minute (according to PaCO2), inspiratory time of 0.3 s, positive end-expiratory pressure of 6 cm H2O, and PIP of 14–22 cm H2O according to chest excursion and the infant’s weight. FiO2 was set to keep adequate oxygen saturation by pulse oximetry. Ventilator settings after extubation were those used for endotracheal ventilation prior to extubation in some studies (Khalaf et al. 2001; Friedlich et al. 1999; Moretti et al. 1999) or only “sigh” ventilation in other studies (Barrington et al. 2001).
40.1.1.3 Nasal Flow
Nasal cannulae have been used to deliver oxygen at flow rates of 0.5 l/min to as high as 6 l/min, usually with no intention of delivering CPAP. However, a significant amount of CPAP is generated (“inadvertent CPAP”) and is not measured continuously at the bedside (Locke et al. 1993). One must exercise caution when delivering flow rates greater than 2 l/min via nasal cannula without knowing the amount of pressure delivered. This is also true for the warmed and humidified new delivery systems. Nasal cannula at flows of 1–2.5 l/min can deliver positive distending pressure in premature neonates (Sreenan et al. 2001).
Kubicka et al. (2008) concluded that HFNC therapy using cannulae of 0.2-cm outer diameter can generate some level of CPAP when the mouth is closed. The amount of pressure generated was related to the flow rate, the size of the leak around the nasal cannula, and the degree of mouth opening. They estimated the levels of CPAP created by HFNC by measuring oral cavity pressures. They also raised the important safety and monitoring issues for the use of these devices. They speculated that if the nasal leak is eliminated with the use of cannulae that obstruct the nares completely, then dangerously high distending pressures may be generated when the mouth is closed. Thus, they suggested that HFNC therapy should not be used as a routine replacement for CPAP therapy.
Standard or low-flow nasal cannula is limited by inadequate humidification and unheated air flow. Delivery of such a gas may lead to airway dysfunction and negative respiratory outcomes. Cooling and loss of water from the airways may impair mucociliary transport, increase fluid osmolality, promote bronchospasm, and increase the viscosity of airway secretions. Moreover, considerable energy is required to heat and to humidify gas delivered into the nose, potentially interfering with optimal nutrition and growth (Waugh and Granger 2004; Schulze 2002). On the basis of studies with very low birth weight (VLBW) infants undergoing ventilation, the delivery of nonhumidified gas may lead to increases in air leaks, more severe chronic lung disease, impaired surfactant activity, and changes in pulmonary mechanics (Tarnow-Mordi et al. 1989; Shelly et al. 1988). Delivery of cold, relatively dry gases, particularly at high flow rates, to the nose may also lead to nasal mucosal injury and bleeding, resulting in pain and creating a portal of entry for infectious agents. The warmed and humidified new delivery HFNC devices overcome the disadvantages of commonly used nasal cannula therapy, in which the delivered gas is well below body temperature and only partially humidified (Kubicka et al. 2008).
40.1.2 Ventilator Settings Adjustments During NRS Therapy
40.1.2.1 Nasal Continuous Positive Airway Pressure (NCPAP)
NCPAP pressure may be weaned in the recovery stages of RDS as lung compliance improves to avoid overdistension. Pressures may be increased for lung recruitment in severe RDS (usually up to ~8 cm H2O) while monitoring the hemodynamic effects in the individual patient and chest radiograph to avoid hyperinflation. When treating the acute phase of RDS in the very premature infant, one should consider intubation and surfactant administration instead of increasing the CPAP level. We wean infants to spontaneous unsupported breathing from 4 to 6 cm H2O. We usually do not use lower pressures.
40.1.2.2 Nasal Intermittent Mandatory Ventilation (NIMV)
The setting of NIMV during the time the infant requires NRS is adjusted to maintain the goals of adequate oxygenation and ventilation (permissive hypercapnia). Oxygenation is maintained by FiO2 and mean airway pressure (inspiratory time [IT], peak inspiratory pressure [PIP], and CPAP level) and ventilation by the respiratory rate, expiratory time, PIP, and maintaining adequate CPAP, according to the rules of conventional endotracheal ventilation. Yet, this routine was not tested in controlled trials. For example, no study has shown that higher rates on the ventilator change the PaCO2, while the baby is breathing spontaneously and complements the NRS. Yet, increasing the rates may decrease the work of breathing. Meta-analysis of two trials comparing NIMV and NCPAP in infants with apnea demonstrated no difference with regard to carbon dioxide levels after 4–6 h of support (Lemyre et al. 2002). Nasal ventilation respiratory settings need to be further studied to allow evidence-based recommendation.
40.1.2.3 Nasal Flow
Oxygen should be monitored to keep the required oxygen saturation. An oxygen/room air mixer may be required to assure limitation on the higher level. The CPAP created at different flows of HFNC needs to be studied to guide a controlled use, if one’s intention is to create CPAP with nasal flow. There are fluctuations that are not continuously measured, and this may create obstacles in using formulas created according to flow, gestational age, and weight (Kubicka et al. 2008). Certainly, a high flow limitation should be kept to avoid the complications of air leak.
40.1.3 NRS Failure Criteria
Criteria for failure of nasal support differ between studies (Kugelman et al. 2007; Barrington et al. 2001; Khalaf et al. 2001; Friedlich et al. 1999). The criteria used in the study of Kugelman et al. (2007) for the initial treatment of RDS were clinical deterioration [increased respiratory distress] accompanied by at least one of the following or worsening of the following: pH <7.20 and PCO2 >60 mmHg, PaO2 <50 mmHg or arterial oxygen saturation by pulse oximetry (SpO2) <88 % on FiO2 >50 %, and recurrent significant apnea requiring repeated stimulation or bag-and-mask ventilation despite the use of methylxanthines or adequate nasal support (proper ventilatory settings and no technical problems). It is possible to switch from NCPAP to NIMV in order to try to minimize endotracheal intubation.
40.1.4 NRS Side Effects
40.1.4.1 Short-Term Side Effects
NRS seems to be safe. Of some concern is the study of Morley et al. (2008) that reported higher incidence of pneumothorax (9 %) in the CPAP group (8 cm H2O), as compared with 3 % in the intubation group (p < 0.001). However, other studies did not report such high rates of pneumothorax on NCPAP or on NIMV (Kugelman et al. 2007; Barrington et al. 2001; Khalaf et al. 2001; Friedlich et al. 1999; Moretti et al. 1999; Santin et al. 2004; SUPPORT Study Group of the Eunice Kennedy Shriver NICHD Neonatal Research Network et al. 2010). There was a concern that NIMV might cause more gastrointestinal complications than NCPAP because of gastric distension leading to cessation of feeds or perforation (Sreenan et al. 2001; Garland et al. 1985). However, no gastrointestinal complications were reported in other studies, and time to full feeds was similar in the two methods (Kugelman et al. 2007; Barrington et al. 2001; Khalaf et al. 2001; De Paoli et al. 2003; Davis et al. 2001).
The discomfort related to NRS may be of importance and deserves our attention for two main reasons. First, our aim is to be gentle and to keep a policy of “minimal handling” in the care of premature infants. Second, an irritable infant who “fights” the NRS may be exposed to a higher risk of pneumothorax (Morley et al. 2008). Thus, we have to be aware of the immediate discomfort and possible short- and long-term effects, developmental or hearing problems arising from our treatment.
NRS may irritate the infants because the nasal flow causes high noise levels (Surenthiran et al. 2003; Karam et al. 2008). Surenthiran et al. reported mean noise intensity in the ear at 1 kHz of 55 dB, higher on NCPAP compared with spontaneous breathing or conventional ventilation, and up to 102 dB at some frequencies (Surenthiran et al. 2003). In another study (Karam et al. 2008), mean noise level was 88.6 (SD 18.8) dB and was correlated with flow (p < 0.01) but not with pressure. A noise level above 90 dB was detected in 67 % of the measurements. NRS may cause pain and irritation in relation to nasal flow, high pressure in upper airways and local pressure, and trauma (Shanmugananda and Rawal 2007; Yong et al. 2005). Adequate techniques for fixation of the NRS devices that minimize the friction and direct pressure on the nose may decrease these undesired local effects. Devices that deliver humid and warm nasal flow were shown to reduce the rate of nasal mucosa injury as compared to standard HFNC (Woodhead et al. 2006).
NRS may affect physiological parameters during RDS and while the babies are stabilized, at the phase of recovering from RDS. Yu and Rolfe (1977) concluded that with correct use of NCPAP, an improvement in oxygenation generally occurs without obvious adverse cardiorespiratory effects. However, when appropriate pressures are exceeded, it is possible that both circulatory and ventilatory function might be severely compromised, possibly by overdistension and a decrease in the venous return. Kugelman et al. (2008) concluded that NRS in “stable” premature infants is associated with increased blood pressure and increased discomfort, despite a decreased respiratory rate. The clinical importance of these effects was modest. While these findings are reassuring when NRS is needed, they should be considered when the medical team balances its need and advantages with its adverse effects according to the clinical condition of the infant. To minimize the discomfort related to NRS, we should shorten its use when possible.
40.1.4.2 Long-Term Side Effects
The main goal of NRS is to administer gentle respiratory assistance to premature infants with RDS with avoidance of endotracheal ventilation. NIMV as the initial mode of ventilation was shown to reduce the rate of BPD (Kugelman et al. 2007) (Fig. 40.2). This study was limited by the small sample size of infants <1,500 or <1,000 g. Thus, the results of the study regarding BPD in VLBW infants should be taken with caution. A plausible explanation for the decreased rate of BPD in the NIMV group in this study could result at least partially from the reduced rate of endotracheal ventilation in this group. Previous studies have shown that the lowest incidence of BPD occurred in NICUs practicing early NCPAP instead of initial mechanical ventilation (Avery et al. 1987; Van Marter et al. 2000). Morley et al. (2008) studied infants who were born at 25–28 weeks gestation. While at 28 days there was a lower risk of death or need for oxygen therapy in the CPAP group than in the intubation group, at 36 weeks gestational age, 33.9 % of the infants who were assigned to receive CPAP had died or had bronchopulmonary dysplasia as compared with 38.9 % of infants who were assigned to receive intubation (p = 0.19). In the SUPPORT study (SUPPORT Study Group of the Eunice Kennedy Shriver NICHD Neonatal Research Network et al. 2010), 1,316 infants born between 24 0/7 weeks and 27 6/7 weeks of gestation were randomly assigned to intubation and surfactant treatment (within 1 h after birth) or to NCPAP treatment initiated in the delivery room, with subsequent use of a protocol-driven limited ventilation strategy. They found that “Death or BPD” in the NCPAP vs. surfactant groups did not differ (47.8 % and 51.0 %, respectively). To note, in these studies infants were treated initially with NCPAP and not with NIMV. On the other hand, a trend towards lower rate of BPD in NIMV compared to NCPAP, not reaching statistical significance, was reported in studies comparing the two modes at the post-extubation period (Barrington et al. 2001; Khalaf et al. 2001; Davis et al. 2001). Another retrospective study showed that the number of infants with BPD was significantly less when they were extubated to NSIMV compared to NCPAP (73 % versus 40 %; p < 0.01) (Kulkarni et al. 2006).
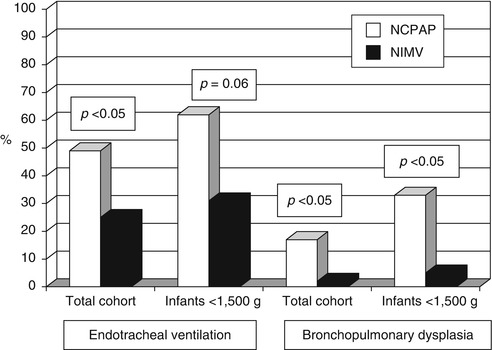
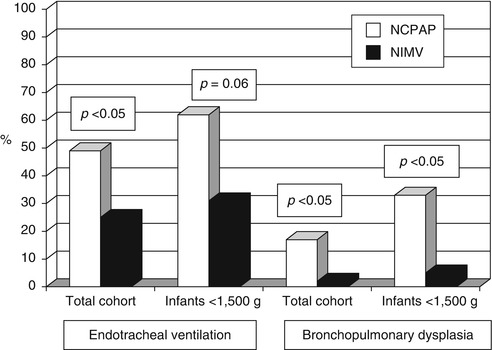
Fig. 40.2
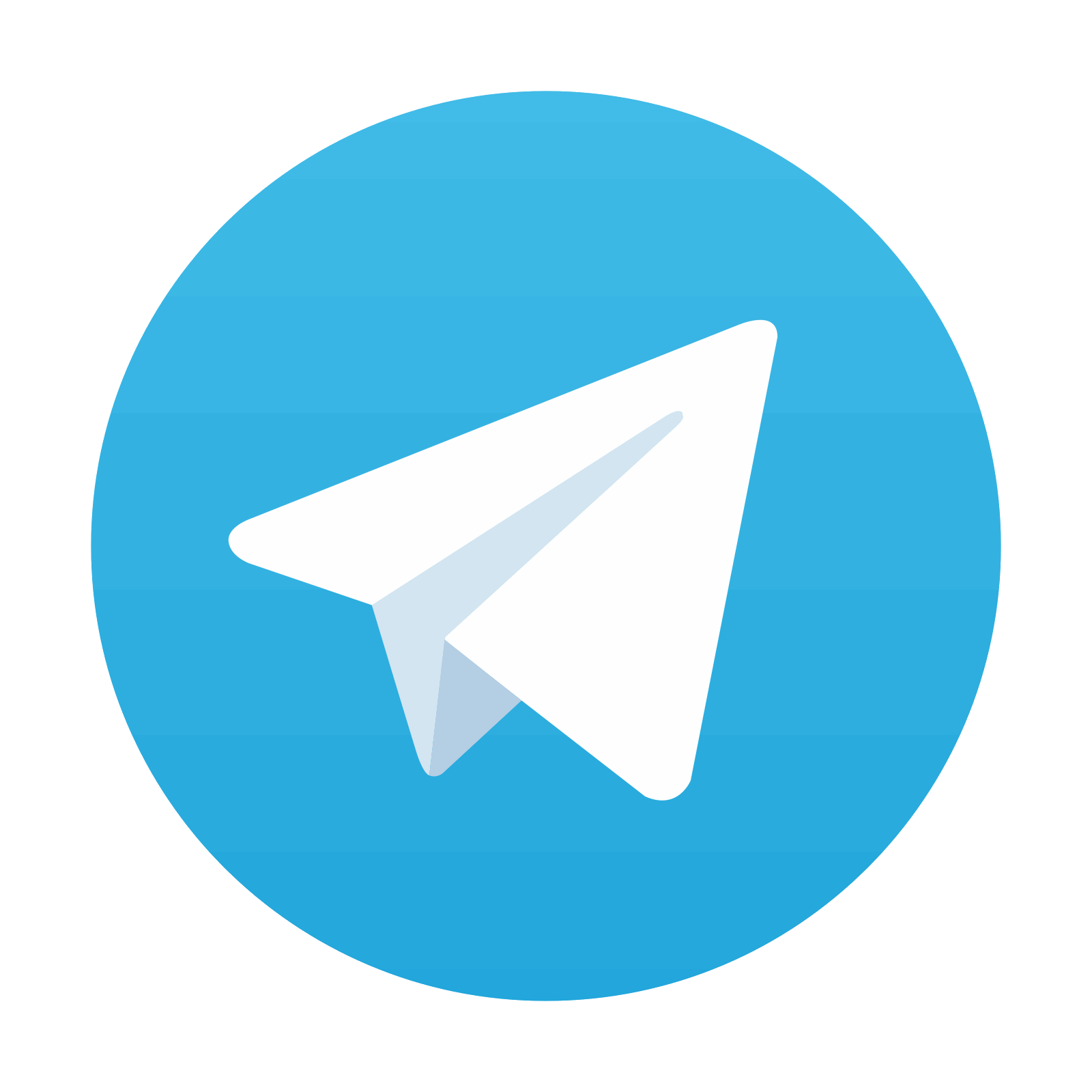
Endotracheal mechanical ventilation and bronchopulmonary dysplasia (BPD) in infants treated with NIMV and NCPAP (Reproduced with permission from Kugelman et al. (2007))
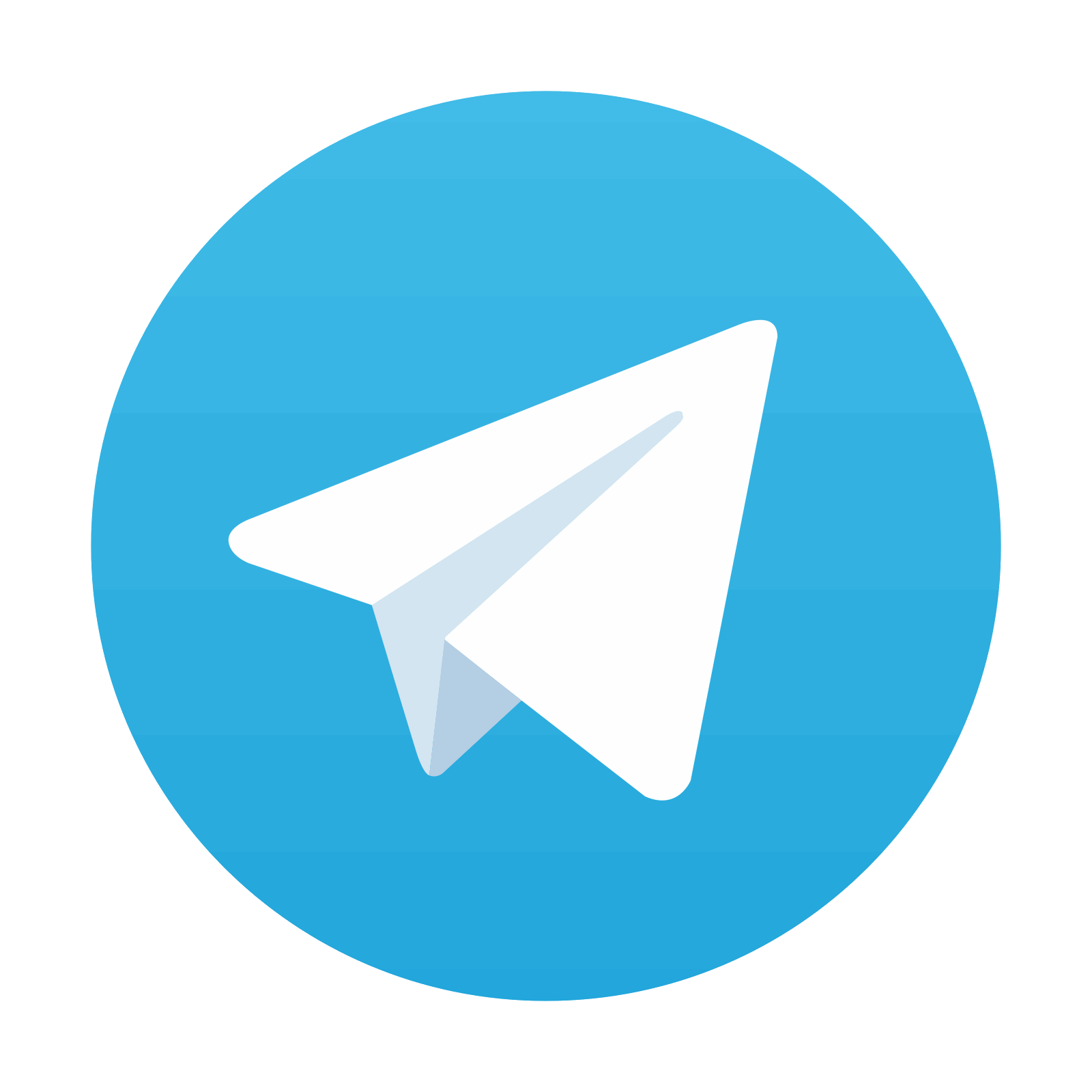
Stay updated, free articles. Join our Telegram channel

Full access? Get Clinical Tree
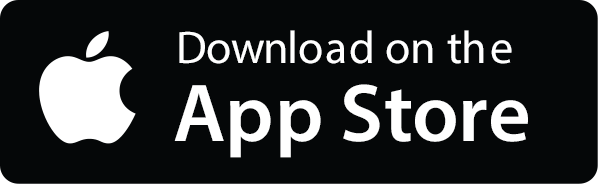
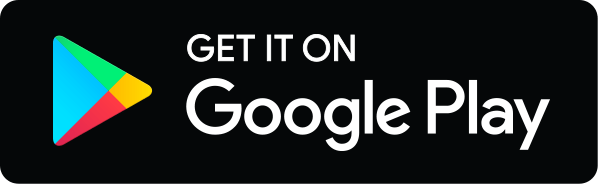
