Key Points
- •
Noninvasive prenatal diagnosis (NIPD) based on analysis of cell-free DNA in maternal plasma for fetal sex determination is now an established clinical service in many countries.
- •
NIPD for a small number of single-gene disorders, including achondroplasia, thanatophoric dysplasia, Apert and Crouzon syndromes, congenital adrenal hyperplasia and cystic fibrosis, is now offered in accredited clinical practice in the United Kingdom.
- •
NIPD for monogenic disorders offers definitive diagnosis and, unlike noninvasive prenatal testing or screening (NIPT or NIPS) for aneuploidy, does not require an invasive test for confirmation of diagnosis.
- •
The potential to test for a wide range of other conditions has been demonstrated, and new technologies and approaches are allowing the development of NIPD for conditions with a more complex genetic basis.
- •
Potential service users and health professionals value the opportunity to have a test with no risk for miscarriage that is available early in pregnancy and easy to access.
- •
Uptake of NIPD is likely to be high, and many women unlikely to request invasive testing would have NIPD if available to inform decisions about termination or to prepare for the birth of an affected child.
- •
NIPD care pathways using straightforward approaches for the exclusion of paternal or de novo mutant alleles are less expensive than invasive testing. However, more complex approaches and likely increased uptake will increase costs above those for invasive testing.
Introduction
The identification of cell-free fetal DNA (cffDNA) circulating in maternal plasma, which is present from early in pregnancy, has paved the way for the development of noninvasive prenatal diagnosis (NIPD), avoiding the small miscarriage risk associated with invasive prenatal diagnosis. The cffDNA is rapidly cleared from the maternal circulation after delivery, and because the whole fetal genome is represented, it offers scope for comprehensive prenatal diagnosis. NIPD for monogenic disorders is diagnostic, and results do not require confirmation on chorionic villi or amniocytes obtained by invasive testing as diagnosis is undertaken in pregnancies known to be at increased risk because of family history or sonographic findings and is targeted at a specific gene or panel of genes. This contrasts with noninvasive prenatal testing or screening (NIPT or NIPS) for aneuploidy, which is considered a very sensitive screening test requiring an invasive test to confirm a positive result ( Table 22.1 ). This is because cffDNA emanates from the placenta, and testing maternal plasma can detect confined placental mosaicism, and because the majority of cell-free DNA (cfDNA) in maternal plasma comes from the mother herself, NIPT can reveal unexpected maternal chromosomal rearrangements. Although maternal mosaicism can complicate NIPD for monogenic disorders, this can be taken into account by testing maternal genomic DNA (gDNA) in parallel with the cfDNA, but as far as we are aware, placental mosaicism for cell lines containing mutations found in single-gene disorders has not been reported.
NIPD for Single-Gene Disorders | NIPT for Aneuploidy | |
---|---|---|
Type of test | Diagnostic | Screening |
Confirmation by invasive testing | Not required | Required |
Approach | Analysis targets possible changes to one or more specific genes | Analysis across all chromosomes or selected chromosomes |
Placental mosaicism | Placental mosaicism for cell lines containing mutations for single gene disorders not reported; does not detect chromosomal mosaicism | False positives can occur because of detection of chromosomal cell lines confined to the placenta (CPM) |
Maternal mosaicism | Controlled for by concurrently testing maternal genomic DNA | False positives can occur because of maternal mosaicism for chromosomal cell lines |
Maternal chromosomal rearrangements | Not detected | Discordant results may result from detection of maternal chromosomal rearrangements, particularly if using the whole-genome sequencing approach |
Maternal tumour cell lines | Not detectable | May be detected if using the whole-genome sequencing approach |
Differences between NIPD and NIPT also apply to the timing of testing, largely because any cffDNA-based test is affected by the fetal fraction. The fetal fraction increases as pregnancy progresses, usually reaching a level that can be used for testing in straightforward applications such as the detection or exclusion of an allele not present in the maternal genome, for example, fetal sex determination, by 7 weeks’ gestation. However, for more complex applications that require accurate quantification (e.g., aneuploidy screening), accurate testing usually requires higher levels of cffDNA, which are attained around 10 to 12 weeks in most pregnancies. It is therefore critical that the gestational age is determined using ultrasonography before embarking on cfDNA testing. Furthermore, ultrasonography should be used to look for evidence of multiple pregnancies, which may complicate results. If an empty sac or ‘vanishing twin’ is identified, this may lead to false-positive results because the placenta continues to shed cffDNA in the absence of a fetal pole. NIPD is possible in multiple pregnancies, but in the absence of any abnormal ultrasound findings, it is not possible to tell which fetuses are affected, and invasive testing will be needed for definitive diagnosis. However, in dizygotic twins, it may be possible to analyse single nucleotide polymorphisms (SNPs) to measure variations in the fetal fraction between genomic regions to determine zygosity and analyse the fetal fragments for each fetus.
It is not possible to separate maternal and fetal cfDNA and, as described earlier, any test based on analysing cfDNA in maternal plasma will analyse both the maternal and fetal fractions, the maternal fraction being the most abundant. Consequently, the early clinical applications of NIPD focussed on the detection of paternal alleles or those arising de novo that were therefore not present in the mother (e.g., fetal sex determination, fetal RHD typing in RhD-negative mothers, paternally inherited single-gene disorders , or single-gene disorders arising de novo , such as achondroplasia ). NIPD can also be used in pregnancies at risk for autosomal recessive conditions if the parents are heterozygous for different mutations. In these cases, termed ‘paternal exclusion testing’, if the paternal allele is identified in maternal plasma, an invasive test is still required for definitive prenatal diagnosis to determine inheritance of the maternal allele and see whether the fetus is affected or not.
Many different laboratory approaches have been reported for NIPD, largely in research settings ( Table 22.2 ). New technologies, such as massively parallel sequencing (MPS), that allow accurate quantification of specific sequences has meant that tests are now being developed that take into account the presence of the maternal cfDNA, thus allowing diagnosis of autosomal recessive X-linked conditions.
Condition | Method | Total Samples | Results | Reference |
---|---|---|---|---|
Autosomal Dominant Conditions | ||||
Achondroplasia | Restriction digest | 1 | 1 affected | Saito et al. (2000) |
Restriction digest | 1 | 1 affected | Li et al. (2004) | |
MALDI-TOF MS | 2 | 2 affected | Li et al. (2007) | |
PCR-RED | 6 | 4 affected 2 unaffected | Chitty et al. (2011) | |
QF-PCR | 2 | 1 affected 1 unaffected | Lim et al. (2011) | |
NGS | PCR-RED: 14/14 affected MPS: 8/9 affected | Chitty et al. (2015) | ||
Apert syndrome | Allele-specific real-time PCR | 1 | 1 affected | Au et al. (2011) |
PCR-RED | 2 | 1 affected 1 unaffected | Raymond et al. (2010) | |
Crouzon syndrome | PCR-RED | 1 | 1 unaffected; recurrence excluded | Raymond et al. (2010) |
Huntington disease | QF-PCR | 1 | 1 unaffected | Gonzalez-Gonzalez et al. (2003) |
QF-PCR | 4 | 2/3 affected 1/1 unaffected | Bustamante-Aragones et al. (2008) | |
QF-PCR | 1 | 1 unaffected | Gonzalez-Gonzalez et al. (2008) | |
Myotonic dystrophy | Nested PCR | 1 | 1 affected | Amicucci et al. (2000) |
Thanatophoric dysplasia types 1 and 2 | PCR-RED | 4 | 3 affected 1 recurrence excluded at 12 wk | Chitty et al. (2013) |
MPS | PCR-RED: 9/11 affected MPS: 9/9 affected | Chitty et al. (2015) | ||
Torsion dystonia | RT-PCR | 2 | 2 affected | Meaney and Norbury (2009) |
Autosomal recessive: parents carrying different mutations | ||||
Congenital adrenal hyperplasia | Fluorescent SNPs | 1 | 1 unaffected | Chiu et al. (2002) |
Craniosynostosis | COLD-PCR | 1 | 1 affected | Galbiati et al. (2014) |
Cystic fibrosis | PCR-RFLP | 1 | 1 affected | Gonzalez-Gonzalez et al. (2002) |
SnaPshot | 3 | 2 affected 1 unaffected | Bustamante-Aragones et al. (2008) | |
NGS panel with 10 common mutations | 4 | 4 correctly classified: 2 inherited paternal mutations | Hill et al. (2015) | |
Haemoglobin E | Nested PCR and restriction digestion | 5 | 3 affected 2 unaffected | Fucharoen et al. (2003) |
Seminested and nested real-time PCR for three different mutations | 39 beta(E) 12 beta(17) 9 beta(41/42) | All correctly classified 26 affected/carrier beta(E) 6 affected/carrier beta(17) 5 affected/carrier beta(41/42) | Tungwiwat et al. (2007) | |
HB Lepore | Allele-specific PCR | 1 | 1 unaffected | Lazaros et al. (2006) |
Leber congenital amaurosis | Denaturing HPLC | 1 | 1 affected | Bustamante-Aragones et al. (2008) |
Propionic acidaemia | SnaPshot; melt curve analysis. | 1 | 1 affected (positive using both methods) | Bustamante-Aragones et al. (2008) |
α-Thalassaemia | Real-time nested PCR | 13 | 8 carriers, 1 HbH, 2 HbBarts, 2 no mutation | Tungwiwat et al. (2006) |
QF-PCR | 30 | 10/30 paternal allele correctly excluded | Ho et al. (2010) | |
β-Thalassaemia | COLD-PCR | 35 | 10/21 affected Cd39 11/21 unaffected 12/14 affected IVSI-110 2/14 unaffected | Galbiati et al. (2011) |
AS-PCR for SNPs | 2 | 1 affected 1 unaffected | Papasavva et al. (2006) | |
APEX | 7 | 3 inherited paternal mutations 3 unaffected 1 incorrect | Papasavva et al. (2008) | |
Genome-wide MPS and SPRT analysis | 1 | 1 carrier | Lo et al. (2010) | |
Fetal DNA enrichment and real-time PCR | 10 | 10 paternal mutations correctly identified | Ramezanzadeh et al. (2016) | |
Autosomal recessive: parents carrying the same mutations | ||||
Congenital adrenal hyperplasia | Targeted MPS and haplotype analysis | 14 | 14 affected | New et al. (2014) |
Targeted MPS and haplotype analysis – hidden Markov model | 1 | 1 unaffected | Ma et al. (2014) | |
Cystic fibrosis | Single-cell short tandem repeat genotyping | 32 | 32 correctly classified (7 affected) | Mouawia et al. (2012) |
Maple syrup urine disease | Targeted MPS and haplotype analysis | 1 | 1 correctly classified | You et al. (2014) |
Methylmalonic academia | Relative mutation dosage with digital droplet PCR and parental SNP analysis | 1 | 1 correctly classified | Gu et al. ( 2014 ) |
Sickle cell disorder | Relative mutation dosage using digital RT-PCR | 65 | 52 correctly classified 7 incorrectly classified 5 unclassified | Barrett et al. (2012) |
PAP | 1 | 1 negative for linked paternal SNP allele | Phylipsen et al. (2012) | |
Spinal muscular atrophy | Single-cell short tandem repeat genotyping | 31 | 31 correctly classified (7 affected) | Mouawia et al. (2012) |
Targeted MPS and haplotype analysis | 5 | 5 correctly classified | Chen et al. (2016) | |
α-Thalassaemia | Real-time quantitative PCR | 158 | 61/62 affected Sensitivity: 98.4% False-positive rate: 20.8% | Sirichotiyakul et al. (2012) |
Allele-specific real-time PCR | 67 | 33/67 correctly classified unaffected | Yan et al. (2011) | |
β-Thalassaemia | Relative mutation dosage using digital PCR | 10 | 5 correctly classified 1 incorrect 4 unclassified | Lun et al. (2008) |
Targeted MPS and relative haplotype dosage | 2 | 2 correctly classified as carriers | Lam et al. (2012) | |
(PAP) | 13 | Paternal SNP allele detected in maternal plasma in 13 cases | Phylipsen et al. (2012) | |
Targeted MPS and haplotype analysis | 10 | NIPD possible in 8/10 cases | Papasavva et al. (2013) | |
High-resolution melting analysis | 50 | 25/27 correctly classified as affected 19/23 correctly classified as unaffected | Zafari et al. (2016) | |
X-linked | ||||
Haemophilia A and B | Relative mutation dosage using digital PCR | 7 | 3/3 affected haemophilia A 4/4 affected haemophilia B | Tsui et al. (2011) |
Retinitis pigmentosa (X-linked) | Sequencing | 1 | 1 mutation on paternal allele detected | Bustamante-Aragones et al. (2006) |
DMD and Becker muscular dystrophy | Targeted MPS and haplotype analysis | 9 | 2/2 affected DMD 7/7 unaffected | Parks et al. (2016) |
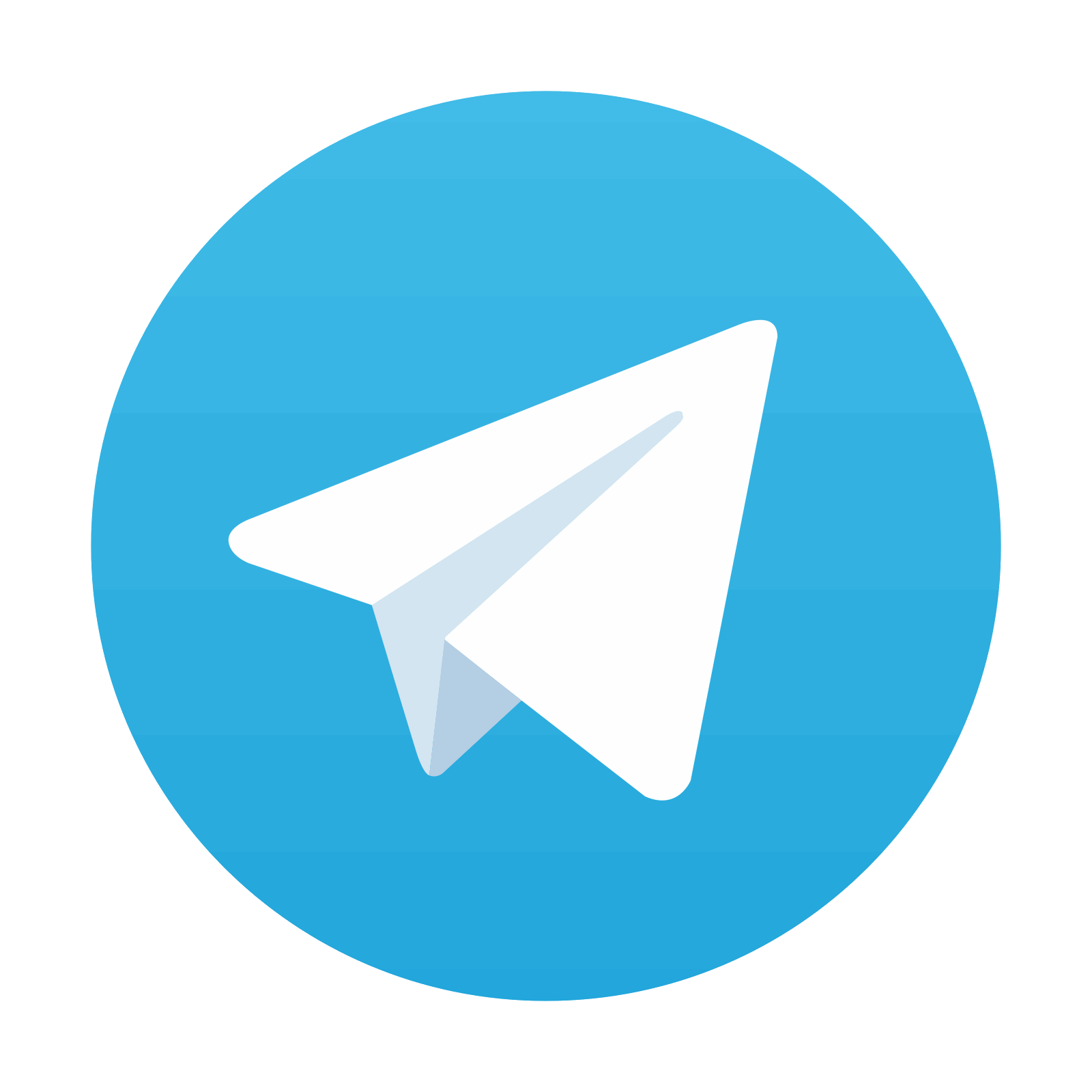
Stay updated, free articles. Join our Telegram channel

Full access? Get Clinical Tree
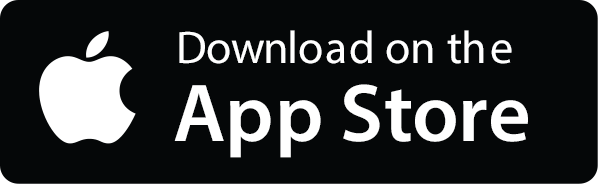
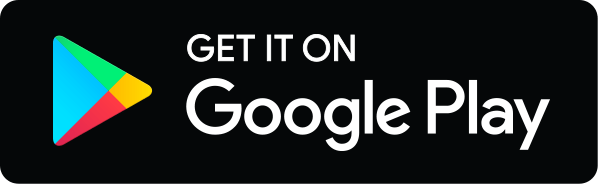
