KEY POINTS
- 1.
Seizures are very common in the neonatal period and constitute a neurologic emergency.
- 2.
Specific aspects of the physiology of the developing brain make it highly susceptible to hyperexcitability and seizure occurrence.
- 3.
Brain injury often causes or contributes to seizures. Among premature infants, seizures are most often triggered by intraventricular hemorrhage; in term babies, hypoxic-ischemic encephalopathy and stroke are the leading causes of seizures.
- 4.
Electrolyte derangements are a frequent and readily treatable cause of neonatal seizures; work-up of the newborn with seizures should assess serum glucose, calcium, magnesium, and sodium.
- 5.
Genetic mutations and inborn errors of metabolism may cause seizures in newborns and should be considered, especially in patients not responsive to antiseizure drug treatment.
- 6.
“Everything that shakes is not a seizure”—A wide differential diagnosis should be considered, including jitteriness, benign neonatal sleep myoclonus, and drug exposures, especially when the electroencephalogram (EEG) does not confirm the presence of seizures.
- 7.
Continuous video (v)-EEG is the current gold standard for seizure monitoring of newborns. Amplitude-integrated EEG (aEEG) is a useful bedside screening technique.
- 8.
Phenobarbital remains the first-line antiseizure drug for newborns. When unsuccessful, additional drugs should be considered promptly, including fosphenytoin and levetiracetam. Infants in status epilepticus should be treated aggressively with those established antiseizure drugs. Status epilepticus refractory to those medications should be treated with a midazolam infusion and a pyridoxine trial.
- 9.
Given the potential for adverse effects, antiseizure drugs should be discontinued as soon as clinically appropriate, ideally prior to hospital discharge.
- 10.
Patients with neonatal seizures have an increased risk of epilepsy, intellectual disability, and neuropsychiatric disorders later in life.
Introduction
Seizures are more common during the neonatal period than at any other age, occurring at a rate of 1.5 to 3 per 1000 live births. They should be considered a neurologic emergency and require prompt recognition and treatment.
Seizures can be recognized on an electroencephalogram (EEG) as rhythmic activity lasting at least 10 seconds and consisting of an obvious onset, evolving frequency and amplitude over time, and a definitive offset. In term newborns, seizure activity is characterized by the presence of rhythmic sharp waves and spikes. In preterm infants, seizures are often characterized by low frequency (often approximately 1 Hz) focal discharges, often constrained to a limited area of cortex. , Due to immature myelination, seizures in neonates propagate slowly to other cortical areas. Status epilepticus is diagnosed if electrographic seizure activity exceeds 50% of a 1-hour (or longer) recording epoch.
In full-term infants, seizures can be either “electroclinical” (characterized as having an associated clinical manifestation, such as apnea or rhythmic limb movements, along with EEG evidence for seizure) or “electrographic-only” (also referred to as “subclinical,” i.e., without an observable clinical change). In extremely premature infants, seizures are almost always electrographic only. EEG monitoring is an essential part of neonatal intensive care unit (NICU) care, as many seizures in this age range are clinically silent.
Pathophysiology
The increased susceptibility of the neonatal brain to seizures is due to a combination of extrinsic and intrinsic factors. Extrinsic factors include all of the pathologies to which a newborn may be subjected, such as hypoxia-ischemia, intracranial hemorrhage, infection, inborn and acquired metabolic errors, and congenital brain malformations. Intrinsic factors are those related to aspects of brain maturation, including ion channels, synapses, and circuits ( Fig. 49.1 ; Table 49.1 ).

Ion channels | Earlier development of Na + , Ca 2+ channels |
Delayed development of K + channels | |
Neurotransmitters and synapses | Electrical synapses (gap junctions) common in early development |
Excitatory synapses appear before inhibitory synapses | |
Overexpression of excitatory synapses during critical period | |
NMDA and AMPA receptor subunit configurations favor greater excitability early in life | |
GABA is excitatory in the prenatal and early postnatal period | |
Circuits and networks | Underdeveloped myelination favors focal seizure onset and limited seizure spread |
At any age, seizure occurrence can be conceptualized as an imbalance between excitation (E) and inhibition (I) in neurons, synapses, and brain circuits. When E increases and/or I decreases, either temporarily or on a chronic basis, the balance of E/I tips toward excessive excitability. However, this E/I imbalance concept is oversimplified and seizure generation is likely mediated by additional signaling mechanisms. For a seizure to happen, hyperexcitability must be coupled with hypersynchronous neuronal firing to cause the abnormal excitation (i.e., seizure) to propagate and lead to a clinical event. In neonates, a seizure may involve clinical changes such as rhythmic motor activity or altered vital signs, but often, a seizure on EEG may not have any associated clinical correlate.
During brain development, the regulation or expression of physiologic factors governing neuronal excitation evolve, and a seizure can be generated by different mechanisms and manifestations at different postconceptual ages. A couple of the major concepts are summarized here; for details, the reader is referred to comprehensive reviews. , , ,
Ion Channels
Neuronal action potentials are generated by influx of sodium ions (Na + ) and terminated by efflux of potassium ions (K + ). Mutation of genes controlling either Na + or K + channels can predispose to hyperexcitability and seizure generation. For example, mutations in the gene that codes for one type of voltage-dependent potassium channel, KCNQ2 (responsible for action potential frequency adaptation), underlies both a relatively benign epilepsy (benign familial neonatal epilepsy) and a severe epileptic encephalopathy. Likewise, mutation in the gene SCN1A , which codes for voltage-dependent sodium channels, underlies Dravet syndrome, in which seizures typically begin in the toddler age range but could occur in neonates. Of note, development of ion channels mediating depolarization (Na + , calcium [Ca 2 + ]) develop earlier than those mediating repolarization (K + ).
Ion Gradients
During the premature period and possibly extending shortly beyond term, the basal intracellular chloride ion (Cl − ) concentration exceeds the extracellular Cl − concentration. Therefore, when Cl − channels open as a result of GABAergic (gamma-aminobutyric acid) synaptic transmission, Cl − exits from the cell, depolarizing it and causing neuronal excitation (and potentially, seizure activity). As development proceeds, the Cl − gradient reverses, and the extracellular Cl − concentration becomes much higher than the intracellular Cl − concentration; in that case, activation of GABA receptors promotes an influx of Cl − into the neuron, which hyperpolarizes it, causing inhibition ( Fig. 49.2 ). The reason for the different internal Cl − concentrations at different gestational ages relates to membrane chloride-cation transporters whose differential expression over time leads to the variable Cl − concentrations. During prenatal life, the sodium-potassium-chloride cotransporter 1 (NKCC1) predominates and intracellular Cl − accumulates. By term or shortly thereafter, the expression of NKCC1 declines and there is increased expression of another transporter, potassium-chloride cotransporter 2 (KCC2), which leads to a net efflux of Cl − , leaving the neuron with a lower basal concentration of Cl − . In summary, in the fetal or preterm brain, GABA binding to its receptor results in Cl − efflux, depolarizing the neuron. Postterm, GABA binding results in Cl − entry into the cell, hyperpolarizing it. Hence the physiologic “paradox” of early GABA-induced depolarization (and excitation) is a consequence of the age-related expression of Cl − cotransporters and variable internal Cl − concentrations (see Fig. 49.2 ). Depolarizing GABA plays key trophic roles in the developing brain and is essential for normal synaptogenesis and circuit formation, but this situation comes at the price of increased excitability and seizure predisposition during early brain development.

Synaptic Receptors
GABAergic transmission is excitatory rather than inhibitory in the preterm and early postterm infant, leading to the “paradoxical” GABA excitation just described. This physiologic situation has clinical implications, possibly accounting for the failure of GABAergic drugs (phenobarbital, benzodiazepines) to fully stop neonatal seizures. Studies are ongoing to establish whether specific blockers of NKCC1 (e.g., bumetanide) can ameliorate neonatal seizures by preventing excessive intracellular Cl − accumulation (see Fig. 49.2 ). Hopefully, other rational therapies can be developed based on the specific physiologic idiosyncrasies of early brain development.
There is also a developmental sequence for excitatory neurotransmitters and receptors; excitatory transmission develops earlier than inhibitory transmission. Brain excitation is largely mediated by glutamate, which binds to two classes of glutamate receptor: alpha-amino-3-hydroxy-5-methyl-4-isoxazolepropionic (AMPA) and N-methyl-D-aspartic acid (NMDA) (see Fig. 49.1 ). AMPA receptors mediate fast excitatory transmission and NMDA mediates relatively prolonged excitatory responses. Subunit expression of both AMPA and NMDA receptors favors excessive excitation in neonates. ,
Other Factors Favoring Hyperexcitability in the Developing Brain
Additional physiologic factors in the developing brain promote susceptibility to seizures. Intercellular communication via gap junctions (electrical synapses) plays a pivotal role in neuronal development; fast-acting electrical transmission via gap junctions can facilitate rapid synchrony of neuronal networks and facilitate seizures. Gap junctions are most active prior to chemical synapse formation. Other factors that play a role in early brain excitability include homeostatic regulation of glial K + and the sodium-potassium pump.
Differential Diagnosis
Seizures during the neonatal period are often provoked by acute brain injury, usually in the setting of stroke, intracranial hemorrhage, or hypoxic-ischemic encephalopathy ( Fig. 49.3 ). In addition, a myriad of genetic mutations, inborn errors of metabolism, and other metabolic derangements can lead to neonatal seizures (see Fig. 49.3 and Tables 49.2 – 49.6 ). Not all rhythmic movements in newborns are due to seizures. Nonepileptic causes of paroxysmal events should be considered, especially when episodes of concern are not verified as seizures on EEG ( Table 49.7 ).

Jitteriness |
Benign neonatal sleep myoclonus |
Neonatal tremor |
Hyperekplexia |
Drug exposure Selective serotonin reuptake inhibitors (SSRIs) Caffeine Drugs of abuse (e.g., heroin/opiates, cocaine) Neonatal alcohol withdrawal syndrome |
Motor automatisms |
Dystonic posturing secondary to reflux/Sandifer syndrome |
Apnea, cardiovascular dysfunction |
Evaluation
Seizure occurrence should be followed closely with EEG monitoring ( Table 49.8 ). Continuous video (v)-EEG is the gold standard for seizure identification and monitoring, yet the interpretation of neonatal recordings is challenging, even for experienced readers. There is also considerable interobserver variability and a continuing tendency to treat patients lacking electrographic evidence of seizures. Prompt laboratory and imaging work-up to search for possible etiologies should be pursued ( Table 49.9 ).
Technique | Advantages | Disadvantages |
---|---|---|
Amplitude-integrated EEG (aEEG) | Economical Readily available Easy lead placement Ongoing bedside evaluation | Limited number of electrodes Lower sensitivity, especially when seizures are brief and distant from recording leads Very vulnerable to artifacts, which are especially prevalent in preterm infants |
Continuous video (v)-EEG | Current gold standard for seizure detection | Time intensive Not available at all institutions Interpretation requires specially trained personnel |
Initial labs | Prioritize: Arterial blood gas, basic metabolic panel, complete blood count, transaminases, calcium, magnesium, glucose |
Infectious work-up | Prioritize: Blood, urine, and CSF cultures Consider TORCH screen (the acronym TORCH complex or TORCHes infections refers to the congenital infections of toxoplasmosis, others [syphilis, hepatitis B, varicella-zoster, and Zika virus], rubella, cytomegalovirus [CMV], and herpes simplex) Septicemia without meningitis may also cause seizures |
EEG | Prioritize: Continuous EEG if available (otherwise consider aEEG) |
Imaging | Prioritize: Head ultrasound If available and when patient is stable: Magnetic resonance imaging Consider: Magnetic resonance angiography and venography if clinically indicated |
Metabolic work-up | Prioritize: Ammonia, lactate, pyruvate, acylcarnitine profile, plasma amino acids, urine organic acids, urine sulfites If indicated: CSF amino acids (pair with plasma) CSF glucose (pair with plasma), pyruvate, lactate CSF neurotransmitters Consider: Genetic testing for ALDH7A1 mutations, often as part of a panel Alternatively: Consider urine alpha-aminoadipic semialdehyde (deficiency contributes to pyridoxine-dependent epilepsy) |
Additional considerations | Important: Placental pathology (in cases of suspected hypoxic-ischemic encephalopathy or arterial stroke) If possible: Expedite newborn screen results Consider: Expedited genetic work-up |
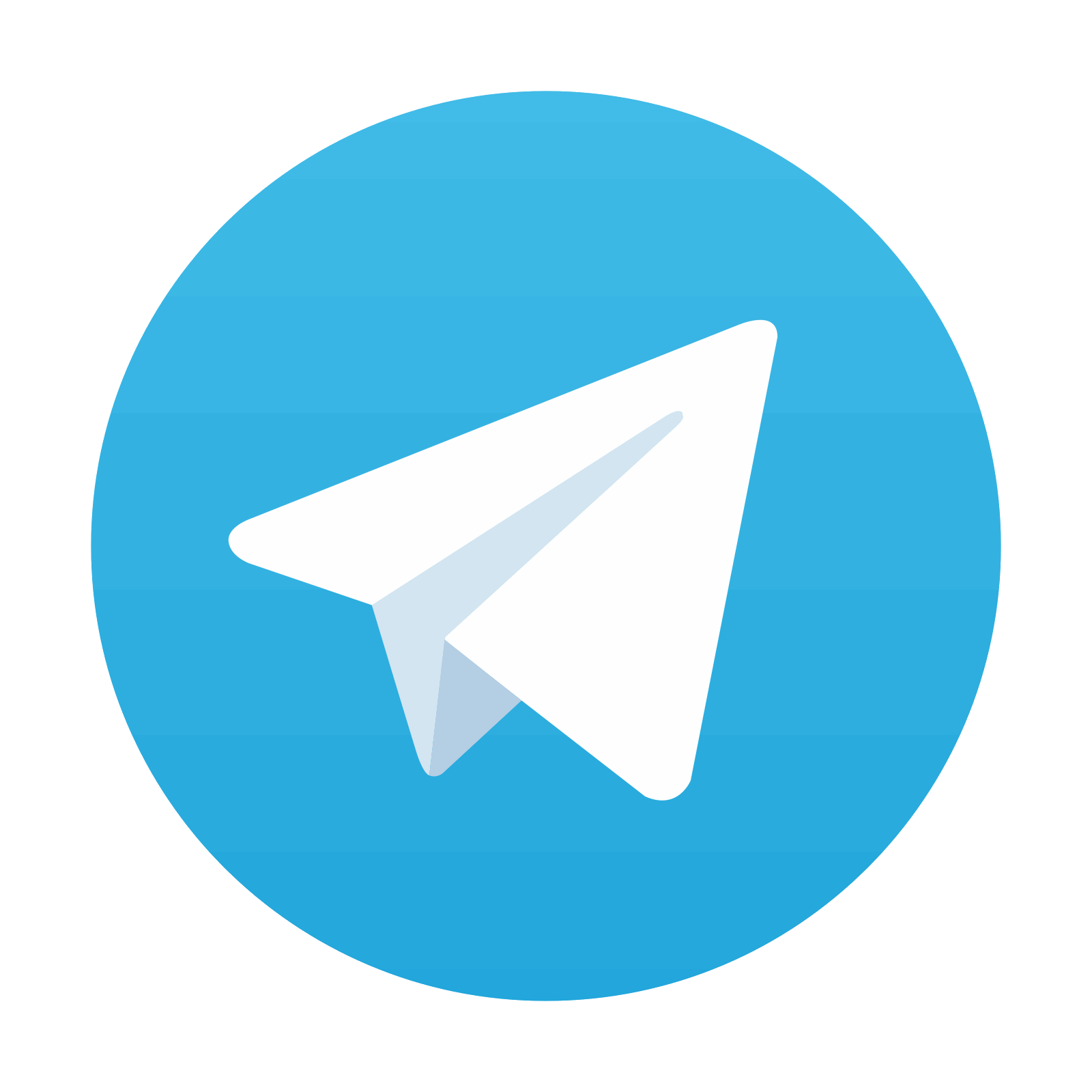
Stay updated, free articles. Join our Telegram channel

Full access? Get Clinical Tree
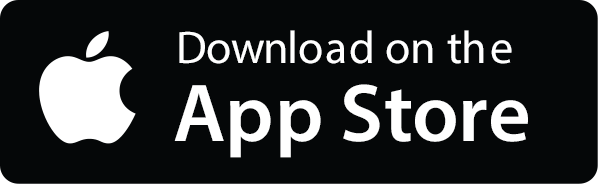
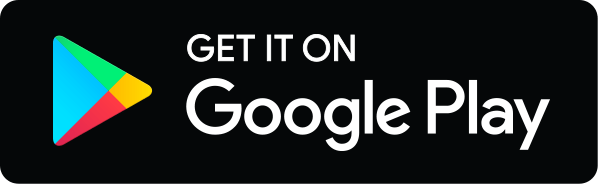
