Chapter Contents
Congenital abnormalities 388
Macrosomia 388
Hypertrophic cardiomyopathy 389
Intrauterine growth restriction 389
Effects of antenatal and intrapartum hypoxia–ischaemia 390
Stillbirth, encephalopathy, hyperviscosity, polycythaemia, jaundice 390
Respiratory complications 390
Hypocalcaemia and hypomagnesaemia 390
Disordered postnatal metabolic adaptation 390
Iatrogenic complications 391
Outcome 391
Avoiding harm 391
Diabetes may predate pregnancy or a woman may develop transient or permanent diabetes during pregnancy. The fertility and well-being of women with diabetes dramatically improved with the availability of insulin, but a high perinatal mortality rate of 20–25% persisted until the 1960s. In the last three decades perinatal mortality rates have fallen, but these vary between countries and regions and progress against aspirations has been disappointing, with a neonatal death rate in the UK of 9.3 per 1000 births, 3.8 times the national rate ( Table 22.1 ) ( ; ; ; ; ; ).
CEMACH COHORT | UK | RATE RATIO | |
---|---|---|---|
Neonatal death | 9.3/1000 | 3.6/1000 | 2.6 |
Preterm delivery | 37% | 7.3% | 5 |
Congenital anomaly | 5.5% | 2.1% | 2.6 |
Birthweight >90th centile | 52% | 10% | 5.2 |
Shoulder dystocia | 7.9% | 3% | 2.6 |
Erb’s palsy | 4.5/1000 | 0.42/1000 | 11 |
Apgar <7 at 5 min | 2.6% | 0.76% | 3.4 |
Admission NNU | 56% | 10% | 5.6 |
Term admission SC | 33% | 10% | 3.3 |
When there is good preconceptional care and control of diabetes and in the absence of congenital abnormalities or other complications, babies should be managed as any other healthy term baby ( ). There is no indication for routine attendance of paediatricians at the delivery or routine admission to a neonatal unit ( ; ).
However, there may be complications for the fetus and in the newborn. Some neonatal complications are not specific to diabetes in pregnancy, but relate to being born preterm or by caesarean section ( ; ) and are covered elswhere in this book. These will be minimised by careful consideration of timing of delivery, maternal steroid administration prior to preterm delivery and reduced rate of unnecessary caesarean sections. Other complications are secondary to the abnormal metabolic environment of the fetus and ongoing disturbances postnatally and are more specific to diabetes in pregnancy ( Table 22.1 ). Of women who have prepregnancy diabetes, approximately one-third have type 2 diabetes, and the incidence and nature of complications do not vary according to type of diabetes ( ; ). Adequate antenatal screening and assessment should identify the pregnancies at greatest risk so that a management plan may be made, and the baby should be born in a centre where there are staff trained to manage unexpected complications.
Before discussing the aetiology and management of the fetal and neonatal complications of maternal diabetes, it is appropriate to be reminded of Farquhar’s vivid and classic description of babies born after diabetes in pregnancy ( ):
They emerge at least alive from within the fiery metabolic furnace of diabetes mellitus, but they resemble one another so closely that they might well be related. They are plump, sleek, liberally coated with vernix caseosa, full-faced and plethoric. During their first two or more extrauterine hours they lie on their backs, bloated and flushed, their legs flexed and abducted, their lightly closed hands on each side of the head, the abdomen prominent and their respiration sighing. They convey a distinct impression of having such a surfeit of both food and fluid pressed upon them by an insistent hostess that they desire only peace so that they may recover from their excesses.
Congenital abnormalities
Despite major improvements in the care of diabetes in pregnancy, there has been little overall change in the incidence of congenital anomalies ( ; ; ; ). Congenital anomalies in the offspring of women with diabetes occur with a frequency up to 10 times that observed in the general population. Congenital anomalies (along with intrapartum causes) now account for a large proportion of perinatal losses and have replaced respiratory distress syndrome (RDS) as the leading cause of perinatal loss ( ; ; ).
The cause of diabetic embryopathy is not fully understood. Genetic factors (diabetes-related genes) are unlikely to play a role, as the incidence of birth defects is not increased in babies of fathers with diabetes ( ). Also, insulin cannot be teratogenic, because the human placenta is impermeable to insulin at early gestation ( ) and fetal pancreatic β cells are not present before the 10th week ( ). It is likely that congenital anomalies are related to the diabetic intrauterine environment during the period of organogenesis, before the seventh week of gestation ( ). Therefore, most occur before the pregnancy is recognised and the intensified diabetes treatment is initiated. Possible contributory factors are hyperglycaemia ( ; ), hyperketonaemia ( ; ), increased levels of somatomedin-inhibiting factors ( ), decreased myoinositol concentration in the neuroectoderm ( ) and disturbances in the secretion of relaxin, an insulin homologue ( ). Finally, hypoglycaemia can also be embryotoxic in experimental animals ( ) but data from human studies are reassuring ( ).
Anomalies overrepresented in pregnancies complicated by diabetes are caudal regression syndrome ( ; ), neural tube defects, holoprosencephaly ( ), vertebral dysplasia, congenital heart disease, ventricular septal defect, transposition of the great vessels and small left colon syndrome ( ; ).
It is clear that efforts to prevent birth defects should start before conception, with contraceptive advice offered so that every pregnancy can be planned in advance with optimum periconceptual metabolic control. Some reports suggest that early first-trimester improvement in glycaemic control ( ; ; ) combined with prenatal diagnosis of anomalies using serum α-fetoprotein determinations and ultrasound scanning ( ) could reduce the impact of congenital anomalies.
Obstetricians and neonatologists must ensure that there has been adequate counselling of parents, involving the specialist team who will care for the baby postnatally, and that delivery takes place at an appropriate centre (dependent on the nature of the anomaly) to enable early access to specialist care. Routine postnatal echocardiography to screen for congenital heart anomalies is not indicated, unless an abnormality has been suspected on antenatal scanning or the baby presents with clinical signs of congenital heart disease ( ).
Macrosomia
‘Macrosomic’ and ‘large-for-gestational-age’ are not interchangeable terms. Macrosomia describes a baby who is heavier than his or her genetically determined birthweight and the clinical appearance of a baby who has had somatic growth in excess over head growth, and thus may be present in a baby of ‘normal’ birthweight.
Macrosomia and organomegaly attributed to fetal hyperinsulinaemia are well-known characteristics of pregnancy complicated by diabetes ( ; ). Glucose crosses the placenta by facilitated diffusion, therefore maternal hyperglycaemia imposes a carbohydrate surplus on the fetus. The fetus responds with increased secretion of insulin. Because insulin is an anabolic hormone the fetal hyperinsulinaemia stimulates protein, lipid and glycogen synthesis to cause macrosomia. Although this classic maternal hyperglycaemia–fetal hyperinsulinism theory of is widely accepted, the metabolic and endocrine disturbances are much more complex. For example, free amino acids also have a stimulatory effect on the development of the β cell, and the anabolic actions of insulin, in utero at least, could in part be mediated through the insulin-induced release of insulin-like growth factors ( ). In addition, birthweight is positively correlated with maternal concentrations of triglycerides and free fatty acids ( ).
Rates of macrosomia vary between centres; for example, from only 8% with birthweights above the 90th centile in an Italian study of strict maternal diabetic control to 52% with birthweights above the 90th centile in the recent UK cohort ( ; ; ). There is no direct relationship between overall maternal diabetes control and macrosomia ( ) – some babies are born with macrosomia after a pregnancy in which maternal blood glucose levels were well controlled. The reason for this may include the fact that glucose level is not the only parameter describing optimal diabetic control in pregnancy. However associations of birthweight with maternal glucose levels (even when not excessively raised) and cord blood C peptide levels have been reported. Enhanced control of even mild gestational diabetes is associated with reduced fetal overgrowth and risk of shoulder dystocia ( ; ; ; ).
The striking physical appearance ( Fig. 22.1 ) of macrosomic infants has already been described. Much of the increased mass is fat ( ). The organomegaly is selective, the liver and heart are often enlarged and skeletal length is increased in proportion to weight, but the brain size is not increased relative to gestational age and so the head may appear disproportionately small ( ).

The clinical significance of macrosomia is the risk of the complications of delivery of a large infant, such as shoulder dystocia, obstructed labour, perinatal asphyxia and birth injury (e.g. brachial plexus injury or fractured clavicle or humerus) ( ; ; ).
Finally, parents and health professionals must be prepared for ‘catch-down’ in postnatal growth of macrosomic babies, especially when breastfed. This is a normal and healthy adaptation and, provided the baby appears to be feeding well and is healthy, there should be no concern if there is an initial period of slow weight gain such that weight crosses down centile lines ( ).
Hypertrophic cardiomyopathy
Cardiac enlargement and hypertrophy in babies of diabetic mothers were reported as early as 1944 ( ). The condition has now been studied more extensively ( ). Improved echocardiographic techniques have shown a generalized myocardial hypertrophy with a disproportionate thickening of the septum ( ; ; ). Symptomatic infants generally have severe hypertrophy with hypertrophied septal muscles bulging into the left ventricle, thereby narrowing the left ventricular outflow tract ( Fig. 22.2 ). Although the condition is transient it may cause fetal or neonatal death ( ; ). The cardiomyopathy has been related to maternal diabetes control ( ; ) and to fetal and neonatal hyperinsulinaemia ( ). Resolution of the signs can be expected in 2–4 weeks, and the septal hypertrophy regresses within 2–12 months ( ; ) (see also Ch. 28 ).

If clinical signs occur, the presentation is usually within the first weeks of postnatal life with cardiorespiratory distress and congestive heart failure. Systolic ejection murmurs can be heard in most affected infants, and chest radiography reveals cardiomegaly ( ). The majority of the infants need supportive care only. If congestive heart failure develops, propranolol is recommended. ( ). Digitalis and other positive inotropic agents are generally contraindicated because they increase systolic contraction and may exacerbate the outflow tract obstruction ( ).
Intrauterine growth restriction
Maternal vascular disease may complicate diabetes and lead to placental insufficiency and impaired fetal growth, so some infants will be born small-for-gestational-age ( ). Overzealous diabetic control and maternal hypoglycaemia may have the same result ( ). The small-for-gestational-age infant of the diabetic mother appears to be at even greater risk of adverse outcome, especially neurodevelopmental sequelae ( ), because the perinatal problems associated with diabetes in pregnancy are compounded by those of intrauterine malnutrition (see Ch. 10 ).
Effects of antenatal and intrapartum hypoxia–ischaemia
Stillbirth, encephalopathy, hyperviscosity, polycythaemia, jaundice
The mechanism for increased risk of significant fetal hypoxia–ischaemia during diabetes in pregnancy is not fully understood. In the UK cohort of diabetes in pregnancy, 10% of perinatal deaths were related to intrapartum causes ( ). In addition, there will be many babies affected by intrapartum hypoxia–ischaemia born alive and requiring expert resuscitation. This is one of the reasons why delivery after diabetes in pregnancy must occur in units where expert advanced neonatal life support is available. If a neonate has unexpected and severe complications of hypoxia–ischaemia the baby will require transfer to a neonatal unit which provides intensive care, if this is not available in the hospital of birth. As total body cooling becomes an established treatment for hypoxic–ischaemic encephalopathy, transfer for this treatment at a specialist centre should be considered ( ).
Infants born after diabetes in pregnancy have an increased risk of being polycythaemic and developing neonatal hyperviscosity syndrome ( ). Normoblastaemia and extensive extramedullary erythropoiesis were observed as early as 1944 ( ) and the aetiology of the increased erythropoiesis has since been described ( ). Elevated umbilical plasma erythropoietin concentrations have been correlated directly with plasma insulin levels in both infants of diabetic mothers (IDMs) and controls ( ). The raised erythropoietin levels are thought to be secondary to relative cellular hypoxia, which is the result of insulin-induced high glucose uptake and high metabolic rate. A more direct effect of insulin was shown in another study in which insulin stimulated growth in culture of late erythroid progenitors in cord blood from premature infants, term infants and IDM ( ).
Lysis of this red cell load contributes to the prolonged unconjugated hyperbilirubinaemia often found in IDMs. There may also be functional immaturity of hepatic enzymes ( ).
Renal vein thrombosis and thrombosis in other vessels, which has been reported to occur with increased frequency in IDMs, is probably related to polycythaemia and hyperviscosity ( ).
Polycythaemia and hyperbilirubinaemia should be managed as described elsewhere in this book.
Respiratory complications
Although the incidence of RDS was previously reported to be 5–6 times higher after diabetes in pregnancy than in the normal population, this incidence is falling in line with improved control of diabetes and prevention of preterm delivery ( ). Rarely, babies born after diabetes in pregnancy have typical RDS despite a normal lecithin–sphingomyelin ratio in the amniotic fluid. This may be because RDS is secondary to the retarded maturation of the pulmonary surfactant system, including surfactant proteins ( ; ; ), rather than simply to the reduced production of phospholipids. Animal studies have demonstrated that insulin inhibits cortisol-induced lecithin synthesis by pneumocytes, probably by inhibiting the production of fibroblast–pneumocyte factor, which promotes phosphatidylcholine synthesis ( ). It has also been demonstrated that butyrate inhibits the transcription of mRNA for surfactant proteins ( ).
There is also an increased risk for transient tachypnoea of the newborn ( ) or respiratory distress associated with cardiac abnormalities. Finally, many babies are delivered before term and/or by caesarean section, and the contribution of these factors to increased respiratory morbidity from RDS or transient tachypnoea of the newborn must be considered ( ). The management of these respiratory complications, should they occur, should be as recommended elsewhere in this book.
Hypocalcaemia and hypomagnesaemia
Neonatal hypocalcaemia has been reported in up to 50% of babies born after diabetes in pregnancy ( ; ) and both its incidence and severity appear to be related to diabetes control ( ; ). Hypocalcaemia is usually associated with hyperphosphataemia and occasionally with hypomagnesaemia. The aetiology is not entirely clear, but neonatal hypoparathyroidism has been demonstrated and may in part be secondary to maternal magnesium loss ( ; ; ). Perinatal hypoxia–ischaemia may also exacerbate hypocalcaemia.
No published study has commented upon the clinical significance of hypocalcaemia or hypomagnasenia. Usually no clinical signs are seen and no treatment is necessary.
Disordered postnatal metabolic adaptation (see Ch. 34.1 )
Almost all babies, regardless of circumstances of pregnancy, manifest a period (of some hours postnatally) of asymptomatic hypoglycaemia before a spontaneous increase in blood glucose level occurs. This is more prolonged or becomes clinically significant for some babies born after diabetes in pregnancy ( ). However, all regain normal blood glucose control within the first few days after birth. For the full definition and symptoms of hypoglycaemia, see Ch. 34.1 . It is likely that plasma insulin levels fall to normal within 12–24 hours of birth in all infants except those whose mothers’ diabetes was poorly controlled ( ). Where maternal blood glucose levels are raised but below the diagnostic level for diabetes, there is only a marginal and barely significant relationship between maternal fasting blood glucose levels and incidence of neonatal hypoglycaemia ( ).
Hyperinsulinism at birth is the result of increased placental transfer of glucose and other nutrients stimulating increased fetal insulin secretion (see above) ( ). The pancreas shows hyperplasia and hypertrophy of the islets of Langerhans ( ; ) without evidence of pancreatic pathology. An increase of the glucagon and pancreatic polypeptide cell fractions in the pancreas has also been shown by immunocytochemical methods ( ) and a counterregulatory hormone response has been demonstrated which is likely to curtail the period of hypoglycaemia ( ; ; ). However, it has been reported that some infants fail to develop the normal increase in plasma glucagon at 2–4 hours of age ( ).
The effect of hyperinsulinism on the liver has been confirmed using stable isotope methods ( ) which demonstrated decreased glucose production. If raised insulin levels persist beyond the first postnatal day, other metabolic fuels may be affected. For example, in theory plasma free fatty acid and blood ketone body concentrations may be lower than in normal term infants, although clinical studies have not confirmed this ( ). Plasma amino acid concentrations are less affected than in organic hyperinsulinism secondary to congenital pancreatic disorders. The characteristic low blood levels of branched-chain amino acids are a not invariable finding ( ; ), possibly because of other transient disturbances in postnatal metabolic adaptation, including the effects of concurrent asphyxia or hypoxia.
Management must be with the aim of balancing the risks of prolonged and severe hypoglycaemia against the effects of overintrusive practices, such as unnnecessary separation of mother and baby or formula supplementation ( ; ; ).
Those caring for the baby must regularly monitor the baby for abnormal feeding behaviour and neurological signs, and must document their findings. If at any stage there are abnormal clinical signs, the blood glucose level must be measured and an urgent paediatric review arranged. It is UK national guidance that IDMs should have regular blood glucose monitoring ( ). Blood glucose screening may be discontinued when at least two consecutive prefeed blood glucose levels are satisfactory and the baby remains well (see Ch. 34.1 ).
In the healthy baby, blood glucose monitoring should not commence before 3–4 hours of age in order to avoid ‘false positives’ associated with the physiological fall in blood glucose. Measurements should be prefeed. It should be noted that test strip reagents are inaccurate, especially at high packed cell volume ( ). Measurement using an accurate method only occurred in 25% of cases in the UK cohort ( ).
Hypoglycaemia is usually prevented by supporting early (within 1–2 hours after birth) and frequent breastfeeding, as it has been shown that a feed of 10 ml/kg human milk can cause an increase of blood glucose of the order of 1 mmol/l at this time ( ). Additional breast milk or formula supplementation should only occur when indicated by clinical signs and low blood glucose levels. There is evidence in other groups of babies that excessive formula supplementation may impair counterregulatory responses so volumes of formula should be kept to the minimum required ( ).
Sick infants unable to tolerate enteral feeding, or those who remain hypoglycaemic despite full enteral feeds, should receive an intravenous infusion of glucose at an initial rate of 5 mg/kg/min (3 ml/kg/h of 10% dextrose) but increasing rapidly if indicated by repeat blood glucose monitoring. Glucose should not be administered at a rate higher than that required to maintain normoglycaemia, in order to avoid continued stimulation of insulin secretion. Rarely, babies with severe and persistent hyperinsulinism require glucose infusions in excess of 10 mg/kg/min. If enteral feeds have been tolerated these should not be reduced when intravenous glucose is commenced. Neurological signs of hypoglycaemia in any infant warrant immediate correction by a bolus injection of 200–400 mg/kg glucose followed by glucose infusion or increased rate of glucose infusion, the rate being adjusted as necessary to maintain normoglycaemia. In other circumstances, boluses should be avoided as increased insulin secretion and rebound hypoglycaemia will result.
A single injection of glucagon (0.03–0.1 mg/kg), which has a temporary hyperglycaemic effect by releasing glucose from glycogen stores, is a useful measure in the event of delay in siting intravenous lines.
Intravenous glucose should be weaned gradually as indicated by blood glucose monitoring. Rebound hypoglycaemia may occur if the glucose infusion is decreased too quickly.
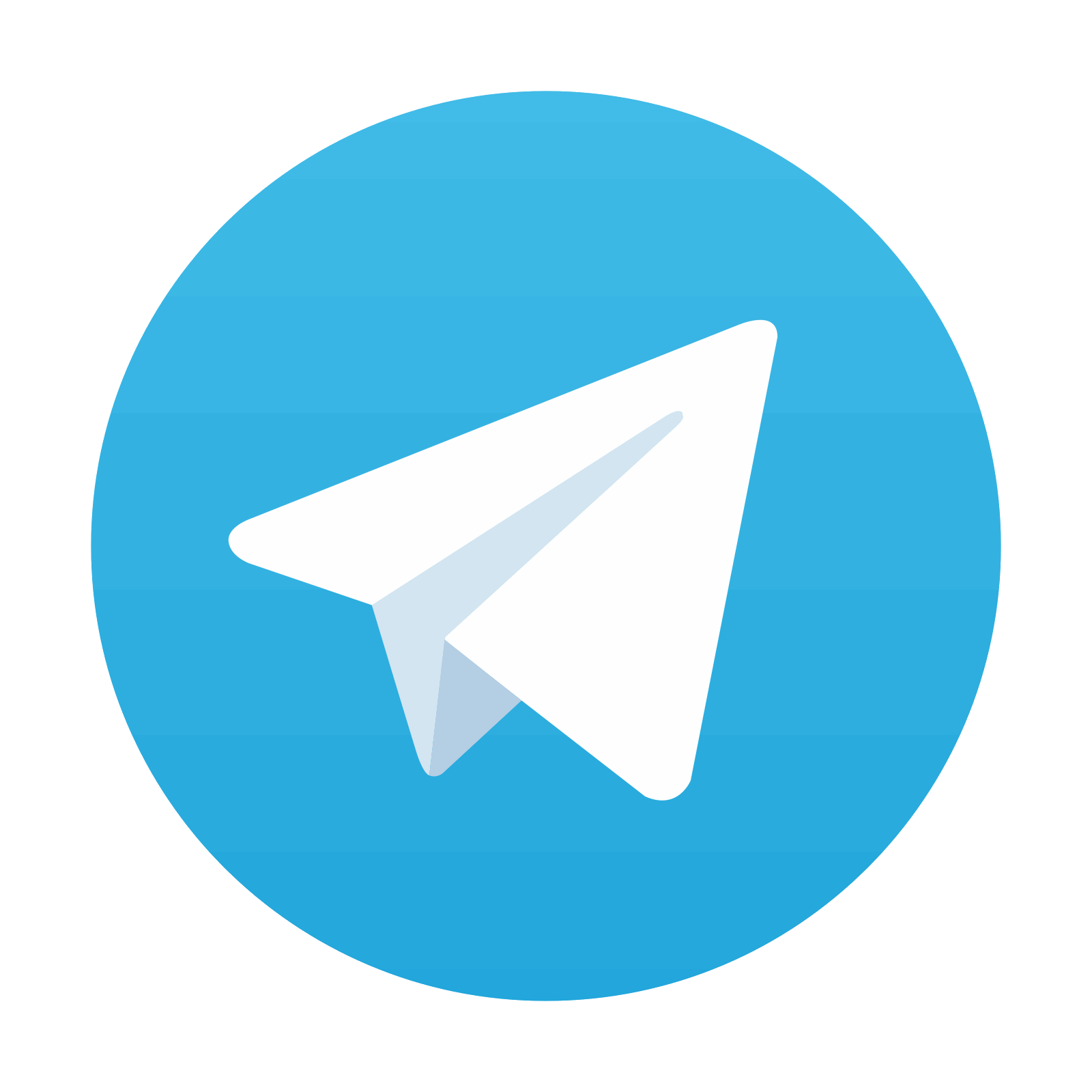
Stay updated, free articles. Join our Telegram channel

Full access? Get Clinical Tree
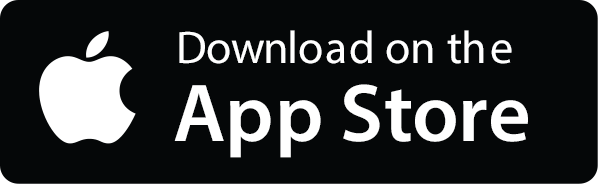
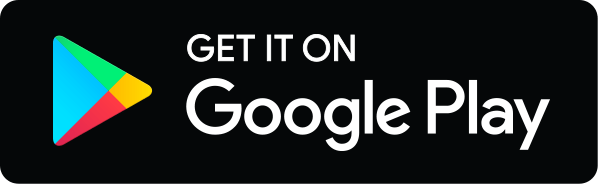