Chapter Contents
Introduction 405
History of drug toxicity in neonates 406
Percutaneous toxicity 406
Protein binding and sulphonamides 406
Impaired metabolism and chloramphenicol 406
Thalidomide and teratogenicity 406
Formulation problems 407
Ceftriaxone and calcium drug interaction 407
Licensing 407
Drug handling 408
Absorption 408
Drug distribution 408
Clinical implications 409
Protein binding 409
Biotransformation 409
Excretion 411
Drug prescribing and administration 411
Oral or nasogastric route of administration 412
Topical administration 412
Intravenous administration 412
Intramuscular administration 412
Rectal administration 413
Drug interactions 413
Pharmacodynamic interactions 413
Pharmacokinetic interactions 413
Drug absorption interactions 413
Protein-binding alterations 413
Enzyme induction 413
Enzyme inhibition 414
Renal excretion 414
Drug therapy for the fetus 414
Corticosteroids in preterm labour 414
Antiarrhythmic agents in fetal tachycardia 414
Antiretroviral therapy to prevent vertical transmission of HIV 414
Endocrine disorders 414
Drugs in breast milk 414
Risk of drug toxicity in breastfed infants 414
Therapeutic drug monitoring 415
Therapeutic ranges 415
Introduction
Medicines have made a significant contribution to reducing both mortality and morbidity in neonates. The benefits of antibiotics, analgesics, surfactant and anticonvulsants are discussed in detail in other sections of this textbook.
The practising neonatologist does not need to be an expert in clinical pharmacology. There are, however, advantages in understanding basic facts regarding how neonates handle medicines and the practical problems associated with the administration of medicines to neonates of different weights and gestation.
History of drug toxicity in neonates
Some of the most important examples of drug toxicity in humans have occurred in newborn infants or the developing fetus. These cases illustrate the difference between neonates and adults in relation to drug absorption, distribution, metabolism and toxicity, as well as formulation issues ( Table 24.1 ).
YEAR | DRUG/COMPOUND | TOXICITY | MECHANISM |
---|---|---|---|
1886 | Aniline dye | Methaemoglobinaemia | Percutaneous absorption |
1956 | Sulphisoxazole | Kernicterus | Bilirubin displacement from plasma proteins |
1959 | Chloramphenicol | Grey-baby syndrome | Impaired metabolism |
1961 | Thalidomide | Phocomelia | Fetal production of teratogenic metabolite |
1982 | Sodium chloride/water | Alcohol poisoning | Formulation |
1984 | Vitamin E and renal failure | Coagulopathy, hepatic | Formulation? |
1998 | Cisapride | Arrhythmia | Drug interaction |
2006 | Ceftriaxone | Calcium precipitation in heart and lungs | Drug interaction |
Percutaneous toxicity
One of the earliest recognised drug toxicities in humans was methaemoglobinaemia, due to the effects of aniline dye, which was reported in 1886. The dye had been used to stamp the name of the institution on nappies for newborn babies. Ten newborn infants developed cyanosis after percutaneous absorption of the dye. Several months later another outbreak was observed and the connection to the dye was noted ( ). Subsequently there have been other cases of newborn infants developing cyanosis following percutaneous absorption of aniline dye.
The absorption of compounds is enhanced by prolonged contact of a wet nappy with the perineum. The greater surface area to weight ratio of newborn infants in comparison with children and adults results in greater relative exposure of topical medicines/chemicals. Also the relative increase in the water content of the dermis and the thinner stratum corneum facilitate transcutaneous diffusion of small molecules. There have been other examples of percutaneous toxicity occurring in the newborn infant, including neurotoxicity in association with hexachlorophane and hypothyroidism following the use of topical iodine ( ).
Protein binding and sulphonamides
In 1956, Silverman and colleagues reported a difference in mortality rate and kernicterus among premature infants who received two different antibiotic regimens ( ). Neonates who received a combination of penicillin and sulphisoxazole had a significantly higher mortality than those who received oxytetracycline. It was almost 10 years before laboratory studies showed that the sulphonamide had displaced bilirubin from albumin by nature of its higher binding affinity. This marked increase in the free bilirubin concentration resulted in kernicterus. This unfortunate adverse drug reaction shows the importance of considering protein binding of drugs in the neonate. This is especially so in sick preterm infants where there are high plasma concentrations of bilirubin together with an impaired capacity to metabolise and excrete bilirubin due to the reduced activity of glucuronosyltransferase.
Impaired metabolism and chloramphenicol
In 1959, the grey-baby syndrome was reported with the antibiotic chloramphenicol. Newborn infants developed abdominal distension, vomiting, cyanosis, cardiovascular collapse, irregular respiration and eventually death ( ). The following year, pharmacokinetic studies showed that neonates were unable to metabolise chloramphenicol to the same extent as children and adults. This was due to impaired glucuronidation of chloramphenicol, and a halving of the dose of chloramphenicol prevented the development of the grey-baby syndrome. Recognition of the limited capacity for drug metabolism in the neonate has led to more appropriate dosage regimens for other medicines.
Thalidomide and teratogenicity
Only 2 years after the death of several newborn infants in association with the use of chloramphenicol, a short letter to The Lancet reported the possible association between the administration of thalidomide, given as an antiemetic or sedative during pregnancy, and multiple congenital abnormalities in the newborn infant, in particular phocomelia ( ). Thalidomide was an exceptionally safe antiemetic when studied in healthy adult volunteers. Animal studies involving thalidomide in pregnant rats initially showed no evidence of teratogenicity. Careful retrospective analysis showed that the effect of thalidomide on the human fetus was time-specific ( Table 24.2 ). Subsequent studies in rats showed that the species was sensitive but only at 12 days’ gestation. It is important to remember the difference between the human fetus and animal models and to be aware of the potential teratogenic effect of medicines that are otherwise exceptionally safe.
DAYS’ GESTATION ON EXPOSURE | EFFECT |
---|---|
21–22 | Absence of external ears and paralysis of central nerves |
24–27 | Phocomelia of arms |
26–29 | Phocomelia of legs |
34–36 | Hypoplastic thumbs and anorectal stenosis |
Thalidomide itself is not teratogenic. It is the metabolites of thalidomide, in particular the monocarboxylic and dicarboxylic metabolites, that are teratogenic. These metabolites, however, do not cross over from maternal plasma into the fetus. Thalidomide itself crosses over into the fetus and is then metabolised to produce the toxic metabolites. It is unfortunate that the fetus with limited capacity to metabolise medicines can, in this case, metabolise the drug with the subsequent formation of a potent teratogen.
Formulation problems
In the early 1980s there were two major tragedies involving premature infants that were thought to be related to the constituents of the medicinal products rather than the drug itself. Ten ventilated preterm infants developed central nervous system depression with associated hypotonia, apnoea and seizures ( ). This was followed by a severe metabolic acidosis and multiorgan failure and was described as the gasping syndrome. These infants all had umbilical arterial catheters and were receiving multiple injections of heparinised bacteriostatic sodium chloride, used for flushing the catheters, and also medications reconstituted with bacteriostatic water. Both the sodium chloride and the water contained 0.9% benzylalcohol. The infants who died had significant levels of benzylalcohol in their serum.
The unit that described this inadvertent poisoning stopped using bacteriostatic saline and water and subsequently no further cases were noted. The healthy adult can tolerate up to 30 ml of 0.9% benzylalcohol as a single dose (approximately 0.5 ml/kg). The newborn infants who developed the gasping syndrome were receiving between 20 and 50 times this amount on a daily basis.
In 1983, an intravenous (IV) form of vitamin E was marketed as a prevention for the retinopathy of prematurity. Seven months later, E-ferol was withdrawn from the market following the deaths of 38 infants who had received the drug ( ). The affected infants showed signs of hepatic, renal and haematopoietic toxicity. It has been postulated that the emulsifiers used to solubilise vitamin E were responsible.
Ceftriaxone and calcium drug interaction
Ceftriaxone is a widely used cephalosporin. It is a highly protein-bound antibiotic and may displace bilirubin. Despite these concerns it has, however, been extensively used in neonates. In 2002 a fatality was reported following co-administration of ceftriaxone and calcium-containing solutions to a neonate in France. This report resulted in a national pharmacovigilance investigation and in 2006 the French Health Products Safety Agency issued a warning letter regarding the risk of precipitation of ceftriaxone–calcium salt in neonates. There have been seven reported deaths worldwide ( ). Ceftriaxone is therefore no longer recommended in the neonatal period ( ).
Licensing
The Medicines Act of 1968 requires that all medicines manufactured or marketed in the UK have been authorised and examined for efficacy, safety and quality. The Medicines Act was introduced in response to significant cases of drug toxicity that occurred in the late 1950s and early 1960s. Two of these involved the developing fetus (thalidomide) and the newborn infant (chloramphenicol). It is ironic that legislation that was introduced to protect all humans has failed to protect those who are at the greatest risk – newborn infants. This is despite the fact that tragedies in the newborn infant precipitated the legislation.
Unlicensed and off-label use
Unlicensed medicines involve modifications to a licensed medicine (e.g. tablets that are crushed and prepared into suspensions), using chemicals as medicines, the manufacture of formulations under a ‘specials’ licence and using medicines licensed in other countries. A particular problem in neonates and children is the off-label use of medicines. This includes the use of licensed medicines: at doses outside that stated in the product licence; for different indications; outside the licensed age range; and by an alternative route, e.g. the use of an IV formulation orally.
Over 50% of drug prescriptions on a British neonatal intensive care unit were off-label and 90% of babies received a drug that was either unlicensed or used in an off-label way ( ). Such results do not imply that current prescribing is inappropriate but reflect the lack of scientific evidence and appropriate formulations for drug therapy in the neonate. In children there is a greater risk associated with the use of unlicensed and off-label medicines ( ). This is not to say that clinicians should restrict themselves to the use of licensed products. However, neonates deserve the same high standards in drug treatment as adults. This can only be achieved by the scientific study of clinically needed medicines in newborn infants in a controlled setting. Such clinical trials would not only provide evidence of efficacy but also reduce the number of deaths associated with the use of new medicines in a non-controlled manner. If there are deaths in a clinical trial, the investigators and regulatory authorities have a responsibility to review the reasons for any deaths at an early stage and to consider whether the experimental drug may be responsible. A review of published paediatric clinical trials over a 7 year period identified 99 randomised controlled clinical trials in neonates ( ). Three of the trials involving neonates were then terminated prematurely by independent safety monitoring committees because of drug toxicity. In nine other neonatal clinical trials, patients experienced severe drug toxicity.
Furthermore, licensing should provide appropriate formulations. Many ampoules of off-label drugs contain amounts such that 10-fold errors can occur with the use of a single vial, e.g. morphine.
Legislative changes
In Europe and the USA, new legislation has been introduced that provides a financial incentive for the pharmaceutical industry to study appropriate medicines in newborn infants. It is essential that any clinical trials in neonates involve medicines that are likely to be of significant clinical benefit to neonates and are not simply studied to increase the considerable profits that can be obtained by such a patent extension.
Drug handling
Large variations in gestation, size and disease states, combined with rapid maturational changes, make optimising drug therapy in the neonatal period more challenging than at any other time. This section focuses on what the body might do to drugs, using specific examples relevant to clinical practice to illustrate key principles. An overview of the major components involved in drug handling is given in Figure 24.1 .

Absorption
Many drugs in neonatal practice are administered IV and bypass the need for absorption. All the drug delivered can be assumed to be available to the body. However, for drugs administered extravascularly, the body compartment to which the drug is delivered is usually separated from the intended site of action and absorption is an important first step in making the drug available. The fraction of drugs absorbed by a given route compared with an IV dose is called the bioavailability. Absorption characteristics are relevant to drugs given orally, intramuscularly, rectally or transcutaneously. In practice, oral administration is the only one commonly used, as the variability from the other sites is usually too great for predictable drug therapy.
Clinical implications
Absorption from the gastrointestinal tract will depend on the physicochemical properties of the drug and individual patient factors and may be significantly different in newborns ( Box 24.1 ).
Drug properties
Molecular weight
Lipid solubility
Degree of ionisation
Drug release characteristics
Drug-induced alterations in gut motility
Patient characteristics
Surface area available for absorption
pH of stomach and duodenum
Gastric emptying rate
Gastrointestinal microflora
Enzyme activity in gut wall
Gastrointestinal blood flow
In the immediate newborn period, gastric pH may be neutral. With increasing maturity and postnatal age, there is a decrease in intragastric pH ( ). There is a rapid fall within hours to between 1.5 and 3.5 in preterm and term infants. However, other factors such as feeds make it difficult to maintain an acid pH consistently in the neonatal period. Acid-labile drugs, such as oral penicillin, may have enhanced absorption. Acidic drugs, such as phenobarbital, may have reduced absorption, owing to their increased degree of ionisation in the more neutral environment.
The gastric emptying time will affect the absorption time profile. Gut motility is influenced by gestational age, postnatal age and the type of feeding used ( ). Another variable that alters absorption is the colonisation of the gastrointestinal tract with microflora. This is influenced by maternal flora, type of feed and antibiotic use. The metabolic capacity of microflora may influence absorption of drugs, but the clinical importance of this is far from clear.
Nevertheless, some drugs are given orally with good effect. Caffeine to treat apnoea of prematurity is often administered by an oral route, and effective, therapeutic concentrations of caffeine are achieved rapidly ( ).
Key message
Absorption of drugs given by the extravascular route will depend on the physicochemical properties of the drug and patient characteristics. Extreme variability in patient characteristics means that extrapolating bioavailability data from adult studies is flawed. Furthermore, other issues such as administration difficulties, losses due to vomiting and spillage may be far more important determinants of drug effect than absorption characteristics. Oral administration cannot guarantee predictable drug delivery in the critical situation.
Drug distribution
In an optimum drug dosage regimen, a desired target concentration is achieved. This requires consideration of the distribution volume of the drug, usually referred to as the apparent volume of distribution ( V d ). This is not a real physiological volume but relates the amount of drug in the body to the blood concentration. Distribution of the drug depends on:
- •
the size of the body water and fat compartments
- •
protein-binding capacity
- •
haemodynamic factors such as cardiac output and regional blood flow.
Clinical implications
The age-related changes in body compartments and protein binding are particularly relevant in the neonatal period. In adults, total body water is 50–55% of bodyweight. In an extremely premature infant, total body water constitutes approximately 92% of bodyweight and body fat less than 1%. At term, total body water is around 75% of bodyweight and body fat has increased to 15%. The intracellular volume increases from 25% bodyweight in the preterm infant to around 33% at term. Selecting a drug dose is made more difficult by rapid postnatal changes. There is a rapid contraction in the extracellular volume shortly after birth, with interstitial fluid loss ( ).
For water-soluble drugs, the differences in body composition tend to result in a V d that is greater in newborns, especially preterms. Therefore, at equal doses per bodyweight, peak concentrations in blood will be lower (although mean concentration at steady state is unaffected by V d ). For drugs distributed mainly in the extracellular fluid space, the dose required on a milligram per kilogram basis would be significantly greater, because of the larger extracellular fluid compartment. However, for highly lipophilic drugs, very premature infants may have a reduced V d .
Aminoglycosides, such as gentamicin, bind minimally to proteins and are mainly distributed in body water. Therefore, in preterm infants, the V d is greater (0.35–0.75 l/kg) ( ; ) and a higher loading dose is required.
By contrast, benzodiazepines are lipophilic and highly protein-bound. The median V d of midazolam in preterm neonates is 1.1 l/kg, which is similar to that seen in children or adults ( ). Recommended loading doses per bodyweight are similar in neonates and children.
Protein binding
The pharmacological effect of a drug is related to the unbound fraction in the blood. The degree of binding to plasma proteins is a major factor in determining drug distribution. The age-related quantitative and qualitative changes in plasma proteins are crucial to the likely clinical effects. Acidic and neutral drugs are largely bound to albumin, whereas basic drugs bind to albumin, α 1 -acid glycoprotein and lipoprotein.
Clinical implications
With increasing prematurity, the total plasma protein and plasma albumin are lower ( ) and the binding affinity less. The consequence is increased concentrations of unbound drug. Protein binding is also affected by endogenous substances, most notably bilirubin. Because of competitive binding, increased bilirubin levels may displace and therefore increase the free drug levels. Conversely, certain drugs may displace bilirubin from albumin-binding sites and so increase the risk of bilirubin toxicity. In practice, it is only when plasma protein binding is high (90% or more) that clinically important displacement of bilirubin will occur. Table 24.3 shows examples of where this may happen.
DRUG | % BOUND TO PROTEIN | POTENTIAL PROBLEM |
---|---|---|
Aspirin | 95 | Yes |
Caffeine | 25 | No |
Ceftriaxone | 83–96 | Yes |
Diazepam | 75–90 | Possible |
Digoxin | 16–30 | No |
Furosemide | 95 | Yes |
Indometacin | 95 | Yes |
Penicillin | 65 | No |
Phenobarbital | 20–35 | No |
Phenytoin | 90 | Yes |
Theophylline | 35–55 | No |
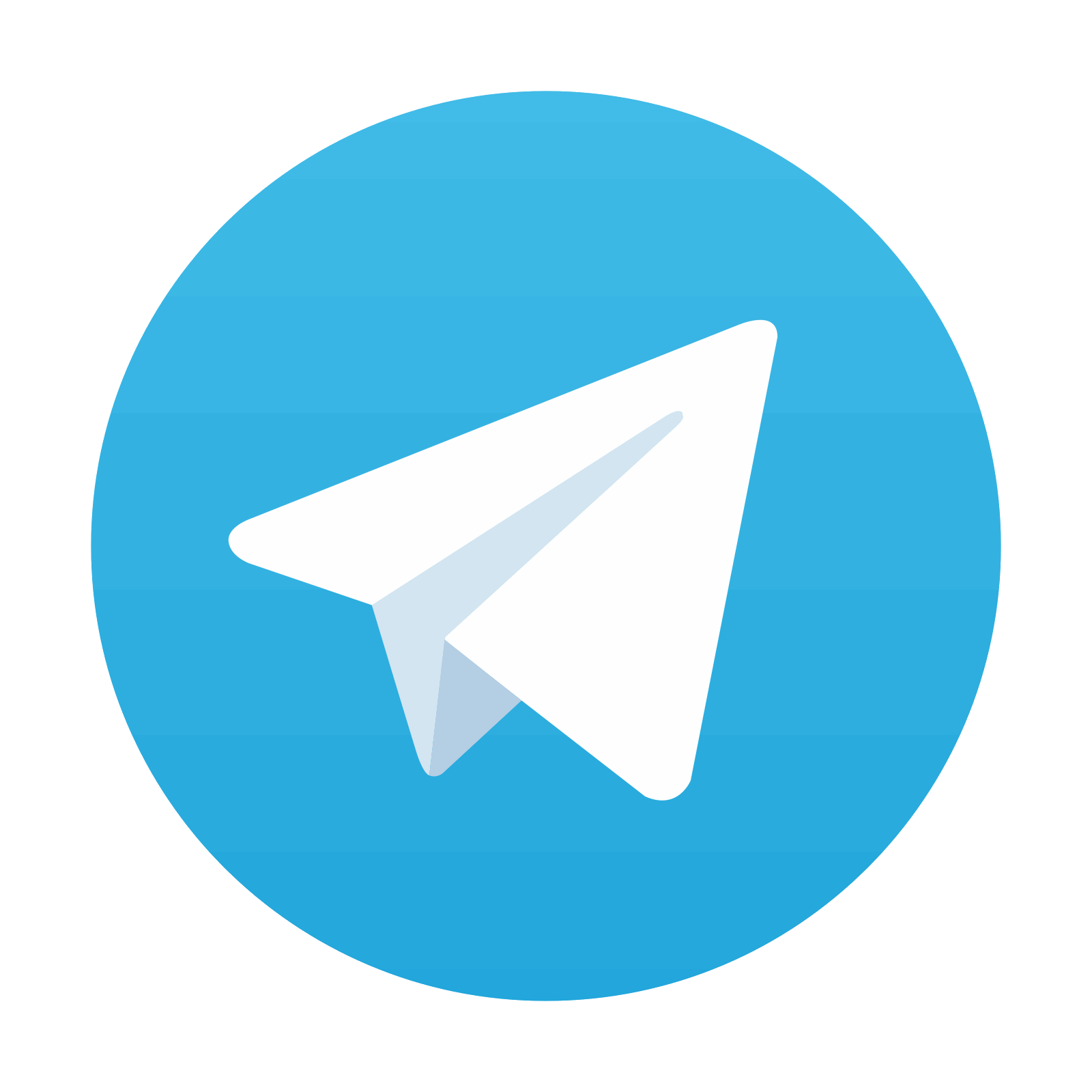
Stay updated, free articles. Join our Telegram channel

Full access? Get Clinical Tree
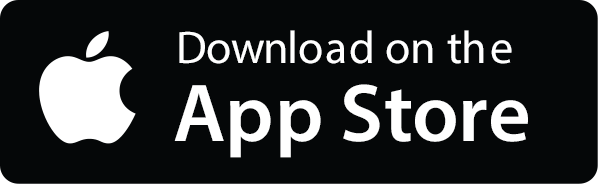
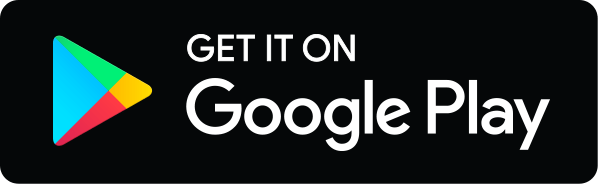
