Keywords
preeclampsia, pregnancy, obesity, insulin resistance, inflammation, endothelium
Editors’ comment: In the early part of the previous century the association between hypertension, increased blood sugar levels and obesity had already been commented on, while during the mid 1900s French medical texts mentioned “goutte diasthesique,” the association of “hypertension, hyperglycemie, hyperuricemie, and hypercholesterolemie.” Years later this became syndrome X, metamorphosing quickly into “metabolic syndrome” (with the latter’s multi-defined criteria). Chesley was aware of such associations too, and in his landmark study of the remote prognosis of eclamptics sought and demonstrated increased hyperglycemia in this population. Since then preeclampsia has been associated with increased insulin resistance, shown to have higher incidence in diabetic and obese women, and subject to scores of observations regarding dyslipidemia. The obesity epidemic, with half of women of reproductive age in the United States obese or overweight, has resulted in this disorder being the leading attributable risk for preeclampsia. The metabolic changes associated with obesity are likely to be related to this increased risk and are reviewed in this chapter.
Introduction
In normal pregnancy, cytotrophoblast cells from the placenta invade the maternal spiral arteries, causing them to lose their smooth muscle and enabling the expansion of vascular capacity necessary to support fetal growth. In some, but not all, cases of preeclampsia there is insufficient remodeling of the spiral arteries, resulting in reduced uteroplacental perfusion. Compromised uteroplacental perfusion is thought to lead to the release of signals (many unidentified), from the placenta into the maternal circulation, that target the maternal vascular endothelium. In women who develop preeclampsia, the effects include widespread evidence of inflammation and endothelial cell dysfunction. These changes are thought to reflect a disturbance of the delicate balance/conflict between mechanisms intended to preserve fetal growth and those that protect the health of the mother.
A salient feature of preeclampsia, however, is its heterogeneity. Indeed, there are inconsistencies with the notion that the poorly perfused placenta is the sole origin of factors altering endothelial function and causing preeclampsia. Failure of physiologic transformation of the spiral arteries may also be observed in patients with (1) fetal growth restriction without preeclampsia, (2) preterm labor and intact membranes, (3) preterm premature rupture of membranes, and occasionally (4) clinically uncomplicated pregnancies. Conversely, the maternal preeclampsia syndrome may occur with minimal evidence of failed spiral artery transformation. Maternal constitutional susceptibility is probably a determining factor. Gestational diabetes and prepregnancy obesity are often associated with larger babies yet predispose to preeclampsia. Preeclampsia superimposed on chronic hypertension or diabetes mellitus confers a higher risk of poor perinatal outcome compared to preeclampsia alone. Furthermore, not only is there an excess of cardiovascular disease in women with a history of preeclampsia, but preeclampsia and cardiovascular disease share obesity, insulin resistance, diabetes, and inflammation as risk factors. These data are consistent with maternal factors as important determinants of preeclampsia.
The metabolic syndrome (MetS), also known as syndrome X, or the insulin resistance syndrome, or Reaven syndrome, comprises a constellation of metabolic factors and physical conditions associated with increased risk of development of type 2 diabetes and cardiovascular disease. The chapter first overviews some of the ways in which the MetS is thought to promote cardiovascular disease. It then uses this as a platform to focus on evidence that the components of the MetS, particularly chronic insulin resistance, inflammation and dyslipidemia, contribute to maternal endothelial cell dysfunction and the preeclampsia syndrome. The chapter then summarizes evidence suggesting that the same underlying MetS-related disturbances that contribute to preeclampsia also increase a woman’s risk of developing cardiovascular disease in later life. Perhaps diverging from conventional thinking, the review then extends this hypothesis to suggest that the MetS actually contributes to poor placental development, with maternal and placental processes then cooperating in a vicious circle of inflammation and endothelial dysfunction. The intended “take-home” message is that obesity and other factors related to the metabolic syndrome construct should be a focus for clinical and research strategies for prevention of preeclampsia and related pregnancy complications, and their cardiovascular sequelae.
Metabolic Syndrome
The clustering of metabolic and physiological abnormalities was first described in 1923 by Eskil Kylin as a syndrome consisting of hypertension, hyperglycemia, obesity, and hyperuricemia. Gerald Reaven, in his 1988 Banting Lecture, described “syndrome X” as the clustering, more often than predicted by chance, of resistance to insulin-stimulated glucose uptake, hyperinsulinemia, hyperglycemia, elevated very low-density lipoprotein (VLDL) triglycerides, reduced high-density lipoprotein (HDL) cholesterol, and hypertension. Criteria for the MetS have evolved over the past decade ( Table 7.1 ) based on the prevailing views of its pathogenesis.
Components | WHO 1998 | NCEP 2001 | NCEP 2004 | IDF 2005 |
---|---|---|---|---|
Insulin resistance | Glucose uptake below lowest quartile under hyperinsulinemic and euglycemic condition | |||
Hyperglycemia | ||||
Fasting plasma glucose (mg/dL) | ≥110 | ≥110 | ≥100 | ≥100 |
Impaired glucose tolerance | Included | Recommended | ||
Previously diagnosed diabetes | Included | Included | Included | Included |
Central obesity | ||||
Waist-to-hip ratio and/or | >0.85 (>0.9) | |||
Body mass index (kg/m 2 ) | >30 | |||
Waist circumference (cm) | >88 (>102) | >88 (>102) | ≥80 (≥94) for Europids, ethnic specific | |
Elevated blood pressure | ||||
Blood pressure (mm Hg) | ≥140/90 | ≥130/85 | ≥130/85 | ≥130/85 |
Treatment for hypertension | Not mentioned | Included | Included | Included |
Dyslipidemia | ||||
Triglycerides (mg/dL) | ≥150 | ≥150 | ≥150 | ≥150 or on triglyceride Rx |
HDL-C (mg/dL) | <39 (<34) | <50 (<40) | <50 (<40) | <50 (<40) or on HDL-C Rx |
Treatment for dyslipidemia | Not mentioned | Not mentioned | Not mentioned | Included |
Urinary albumin excretion or | ≥20 μg/min | |||
Albumin/creatinine ratio | ≥30 mg/g |
In 1998, the World Health Organization Task Force on Diabetes identified insulin resistance as the dominant cause of the MetS. By this criterion, clinical indicators of insulin resistance were required for diagnosis ( Table 7.1 ). With growing evidence of a paramount role for obesity, however, the latter has assumed a more important position among diagnostic criteria. Indeed, obesity and physical inactivity are currently considered to be the driving forces behind the MetS, modulated by susceptibility factors including adipose tissue disorders, genetic factors, race, aging, and endocrine disorders. Insulin resistance is often secondary to obesity but can have genetic components as well. These concepts led to the National Cholesterol Education Program (NCEP) criteria for the MetS in which the need for demonstration of insulin resistance was supplanted by increased waist circumference (abdominal obesity).
The majority of epidemiological studies have used NCEP criteria, defining MetS as the presence of three or more of the following five risk factors: abdominal obesity, high triglycerides, low HDL cholesterol, hypertension, and elevated fasting glucose ( Table 7.1 ). The more recent International Diabetes Federation definition requires central obesity plus any two of the other four 2004 NCEP factors ( Table 7.1 ).
Metabolic Syndrome and Cardiovascular Disease
This section of the chapter is intended to illustrate several of the key pathways by which the MetS is thought to promote cardiovascular disease in the general population. Comprehensive reviews are available on the subject of MetS and cardiovascular disease. Contemporary reviews are also available that specifically deal with the question of how prenatal and early postnatal environment/exposures increase later-life susceptibility to obesity, MetS, cardiovascular disease, and type 2 diabetes.
The MetS is becoming increasingly prevalent worldwide. Using the 2004 NCEP glucose threshold of 100 mg/dL ( Table 7.1 ), each of the major components – obesity, elevated triglycerides, low HDL-cholesterol, and elevated fasting plasma glucose – occurs in approximately one-third of the United States population. The MetS often coexists with other medical conditions, including fatty liver, obstructive sleep apnea, gout, depression, and polycystic ovarian syndrome. People with the MetS are at roughly twice the risk of atherosclerotic cardiovascular disease compared to those without. It also raises the risk of type 2 diabetes by about 5-fold. Large-scale clinical trials such as the Women’s Health Study and the Framingham Offspring Study have confirmed the contribution of the MetS to cardiovascular disease in women. Data suggest that the presence of the MetS is more predictive of future cardiovascular disease in women than in men.
Although individuals with the MetS are at increased risk of cardiovascular disease, and although the MetS risk factors are found in combination more often than by chance, it is unclear whether the MetS construct is clinically more useful as a marker of risk above and beyond its individual components (i.e., the whole is not greater than the sum of its parts). By extension, rather than focusing just on the MetS construct as a whole, it will be important to evaluate the connection of preeclampsia with individual MetS-related factors.
Obesity
Obesity is usually defined as a body mass index (BMI)≥30 kg/m 2 , and overweight as BMI of 25.0 to 29.9, with BMI calculated as weight in kilograms divided by the square of the height in meters. BMI as a measure of obesity has limitations because it does not distinguish between fat and lean tissue (nearly half of all National Basketball Association players would be inaccurately classified as overweight or obese on the basis of BMI) or fat distribution. Regardless of whether adiposity is gauged by BMI, waist circumference, bioimpedance analysis of fat mass, or dual energy X-ray absorptiometry scans, it is clear that obesity is a major driving force for the increased prevalence of cardiovascular disease world-wide. This includes the developing world, where rates of obesity have tripled in regions that have been adopting a Western lifestyle. Nations of the Middle East, Pacific Islands, Southeast Asia, and China are facing this threat. Some developing countries experience the paradox of families in which the adults are overweight but the children are underweight, the latter potentially attributable to intrauterine growth restriction which may predispose to later-life obesity via acquisition of the “thrifty phenotype.” In the United States, medical costs and lost productivity attributable to excess adiposity totaled about $117 billion in the year 2000. At least one-third of all pregnant women in the United States are obese; data released in 2013 by the Centers for Disease Control and Prevention show that 57% of African-American women, 44% of Hispanic women, and 33% of white women are obese.
Prospective cohort studies of non-pregnant populations suggest that obesity precedes the development of insulin resistance. The increased risk of adverse health outcomes among obese individuals is significantly influenced by MetS-related pathologies, including inflammation, oxidative stress, insulin resistance, dyslipidemia, and endothelial dysfunction, all of which are significantly more prevalent in women with preeclampsia compared to women with uncomplicated pregnancy. However, approximately 29% of obese men and 35% of obese women (obesity gauged by BMI) in the United States are “metabolically healthy” as defined by having either none or not more than one of the following MetS abnormalities: elevated blood pressure, fasting triglycerides, fasting glucose, C-reactive protein, homeostasis model assessment of insulin resistance (HOMA) value, or reduced HDL cholesterol level. Independent characteristics enriched in this “uncomplicated obese” sub-group include non-Hispanic black race, smaller waist circumference and higher level of physical activity. Therefore, an obese individuals’ cardiovascular disease risk depends jointly on adiposity and metabolic profile.
Adipose tissue is pleiotropic and functions not just as a lipid storage depot but as an active endocrine organ that secretes an array of bioactive molecules called adipokines. Several adipokines are listed in Table 7.2 according to their apparent role in the pathogenesis of endothelial dysfunction and atherosclerosis, and their association with preeclampsia. It is interesting that the majority of these mediators seem to be produced more actively in adipose tissue from obese individuals; that is, the production is not greater simply because of more tissue but also because of predominant location of the tissue (visceral versus subcutaneous) and/or intrinsic differences in the adipocyte with obesity. Visceral and subcutaneous fat are quite different metabolically. Materials produced by visceral fat are drained directly to the liver where they can upregulate the hepatic production of acute-phase reactants and inflammatory cytokines. This is reflected in the increased circulating concentrations of C-reactive protein, plasminogen activator inhibitor 1 (PAI-1) and inflammatory cytokines in individuals with visceral obesity. Also, visceral fat in vitro produces more C-reactive protein and inflammatory cytokines than subcutaneous fat. The importance of adipose tissue versus the placenta as the source of elevated circulating leptin, adiponectin, interleukin-6 (IL-6), tumor necrosis factor (TNF)-α, and plasminogen activator inhibitor (PAI-1) during preeclampsia is, however, unclear.
Endothelial Cell Dysfunction | Atherosclerosis | Preeclampsia | |
---|---|---|---|
Leptin | Increased NO-induced vasorelaxation | Stimulation of thrombus formation | Elevated in plasma from 20 weeks gestation in women who later develop preeclampsia; rises markedly from 32 weeks as preeclampsia develops |
Adiponectin | Decreased expression of adhesion molecules Stimulation of NO-induced relaxation | Inhibition of transformation of macrophages to foam cells Stabilization of atherosclerotic plaques (TIMP-1) | Paradoxically upregulated in preeclampsia; more recent reports variable; correlates negatively with fasting insulin values; possible feedback mechanism to counter insulin insensitivity |
IL-6 | Stimulation of CRP synthesis in the liver (weak evidence that CRP decreases NO production in endothelial cells) | Stimulation of monocyte/macrophages infiltration of atherosclerotic plaque Destabilization of atherosclerotic plaque (matrix metalloproteinases) | Many, although not all, studies show higher third-trimester plasma levels of IL-6 and TNF-α in women with preeclampsia; first-trimester IL-6 concentrations may be elevated in women who later develop preeclampsia |
TNF-α | Increased expression of adhesion molecules | Stimulation of oxidized LDL uptake by macrophages (scavenger receptors) | The source of excess circulating TNF-α in preeclampsia is apparently from macrophages of adipose tissue as placental mRNA is not increased |
Increased expression of MCP-1 and M-CSF | Stimulation of proinflammatory cytokines release by monocytes/macrophages | ||
Destabilization of atherosclerotic plaques (matrix metalloproteinases) | |||
Resistin | — | Promotes fat deposition and has proinflammatory properties | Higher first-trimester resistin in women who developed preeclampsia |
FABP4 | — | Correlated with carotid intimal thickening, insulin resistance and later-life cardiovascular diseae | Higher circulating FABP4 in first and second trimesters in women who developed preeclampsia |
PAI-1 | — | Inhibition of fibrin clot breakdown | PAI-1 is principally of placental vascular endothelial origin and is raised in the maternal circulation of women with preeclampsia |
Angiotensin II | Vasoconstriction | Stimulation of intimal infiltration by monocytes | Preeclamptic patients manifest exaggerated pressor responses to Ang II, although the circulating Ang II concentrations are lower compared to control pregnancies |
Increased expression of adhesion molecules, MCP-1 and M-CSF | Stimulation of migration and proliferation of smooth muscle cells | Evidence for the existence and importance of a local, utroplacental renin-angiotensin system | |
Stimulation of NADPH oxidase (reactive oxygen species production) | Agonistic autoantibodies to the angiotensin II type I receptor are implicated in preeclampsia |
It is clear that visceral fat is a much better predictor of cardiovascular disease than BMI or percent body fat. The central distribution of body fat (increased ratio of waist to hip circumference) is associated with a higher risk of morbidity and mortality than a more peripheral distribution of body fat. Although the information for preeclampsia is limited, there is an increased risk of preeclampsia determined by early pregnancy waist circumference, a surrogate for visceral fat.
Free Fatty Acids and TNF-α
Visceral adipose tissue of obese/insulin resistant individuals releases excess amounts of non-esterified (“free”) fatty acids (FFA) and the inflammatory cytokine TNF-α into the circulation. Insulin inhibits adipocyte hormone-sensitive lipase, decreasing adipocyte triglyceride hydrolysis and thus limiting concentrations of FFA and glycerol in the circulation. Insulin resistance thus increases hormone-sensitive lipase activity and adipocyte lipolysis ( Fig. 7.1 ). The resulting hepatic overload with FFAs increases hepatic triglyceride production and export into the circulation in the form of VLDL; it also leads to hepatic triglyceride deposition, predisposing to fatty liver. Overproduction of VLDL is a fundamental feature of the MetS that initiates a sequence of further lipoprotein changes, namely higher concentrations of atherogenic remnant lipoprotein particles and smaller-sized (more oxidizable) low-density lipoprotein (LDL) particles, and lower concentrations of HDL cholesterol ( Fig. 7.1 ). The MetS is also associated with higher plasma concentrations of oxidatively modified LDL, which, in turn, are associated with increased risk of myocardial infarction even after adjustment for LDL cholesterol and other established risk factors. These lipid abnormalities are also features of preeclampsia.

FFA and TNF-α can reduce endothelium-dependent vasodilatation, increase blood pressure, and further impair insulin sensitivity via mechanisms that are not well understood. Production of reactive oxygen species by FFA and TNF-α is probably heavily involved. Free fatty acids increase the production of superoxide anion radical (O 2 − ) and hydrogen peroxide in several cell types by stimulating the enzyme NADPH-oxidase. In states of adiposity, processing of excess intracellular free fatty acid by the mitochondria causes mitochondrial uncoupling and overproduction of reactive oxygen species. Reactive oxygen species can oxidatively destroy the endothelium-dependent vasodilator nitric oxide (NO), thus reducing vascular relaxation responsiveness. Raised concentrations of FFA can also blunt insulin-induced phosphatidylinositol (PI3) kinase activation in endothelial cells, thus reducing the bioavailability of nitric oxide by inhibiting endothelial nitric oxide synthase (eNOS) ( Fig. 7.2 ). FFA may also increase synthesis of the vasoconstrictor endothelin-1 (ET-1) ( Fig. 7.2 ).

FFAs circulate in the bloodstream bound to albumin. Serum albumin functions as an antioxidant by sequestering copper and by scavenging hydroxyl radicals, the latter by virtue of albumin’s reduced cysteine residue (Cys34). As illustrated in Fig. 7.3 , and as will be discussed in more detail in the context of preeclampsia, oversaturation of albumin by free fatty acids results in a conformational change in albumin such that the copper bound to albumin becomes redox active; albumin is thereby converted from an antioxidant to a prooxidant. The excess ROS produced by these various mechanisms may adversely affect cellular metabolism and subvert endothelium-dependent relaxation by multiple means, including destroying the vasodilator nitric oxide before it can reach its intended targets ( Fig. 7.2 ).

TNF-α inhibits insulin action in adipocytes, possibly through inhibition of insulin receptor substrate 1 (one primary substrate of the insulin receptor) by c-Jun N-terminal kinases (JNK) ( Fig. 7.4 ). In animal models of pre-diabetic metabolic syndrome, endothelial cell expression of TNF-α is increased in association with endothelial dysfunction; blockade of TNF-α restored endothelium-mediated vasodilatation. TNF-α, like free fatty acids, may mediate endothelial dysfunction partly by lowering bioavailable nitric oxide, secondary to both stimulation of ROS and inhibition of eNOS ( Fig. 7.2 ).

Renin-Angiotensin System
Human adipose tissue expresses all components of the renin-angiotensin system. The expression of angiotensinogen in omental adipose tissue is higher in obese compared to lean subjects, and plasma angiotensinogen levels are positively correlated with body mass index (BMI). Besides being potentially important in systemic vasoconstriction angiotensin II may induce oxidative stress by activation of NADPH-oxidase. Several additional angiotensin II signaling pathways are thought to contribute to the chronic sub-acute inflammatory state characteristic of obesity.
Both angiotensin II and circulating autoantibodies that activate the angiotensin type I (AT1) receptor have been implicated in the etiology and pathogenesis of preeclampsia. Information is lacking, however, about any relationship of these agonistic AT1-receptor autoantibodies to the MetS. Suggestive of a relationship, however, the minority of non-pregnant women with a history of preeclampsia who retain agonistic AT1-receptor autoantibodies in their circulation 18 months postpartum also have higher homeostasis model assessment of insulin resistance (HOMA) indices on average than women with prior preeclampsia who do not have evidence of these autoantibodies.
Adipocyte Hypertrophy and Endoplasmic Reticulum Stress
Calories consumed in excess of calories expended (a fundamental cause of obesity) leads to increased storage of the surplus energy in the form of adipocyte intracellular lipid droplets ( Fig. 7.4 ). Whether adipocyte hypertrophy or adipocyte hyperplasia, or both, occur in response to excess postprandial lipids and glucose depends upon the type of adipose tissue. Subcutaneous fat deposition occurs early in development of obesity, with visceral deposition of fat occurring only after subcutaneous capacity has been reached. Macrophages are observed more frequently, along with increased expression of the proinflammatory cytokine monocyte chemoattractant protein-1 (MCP-1), in human omental compared to subcutaneous fat, correlating with waist circumference. Such data support the notion that visceral fat has more adverse health consequences than subcutaneous fat.
Hypertrophy of adipocyte lipid droplets may underlie the endoplasmic reticulum stress and mitochondrial abnormalities that are central to the adverse effects of obesity. One manifestation of endoplasmic reticulum stress is the “unfolded protein response” in which abnormally folded proteins aggregate in the cytosol and interfere with normal cellular functions ( Fig. 7.4 ). The unfolded protein response can further increase cellular insulin resistance, with further increases in lipids and endoplasmic reticulum stress, generating a vicious circle of worsening insulin resistance and apoptosis (programmed cell death) ( Fig. 7.4 ). It has been suggested that endoplasmic reticulum stress and the unfolded protein response are involved in the placental, endothelial, and oxidative stress associated with preeclampsia and intrauterine fetal growth restriction (IUGR) and data support this. However, the relationship of endoplasmic reticulum stress in preeclampsia to maternal obesity and insulin resistance has not, to our knowledge, been explored.
Pregnancy-Induced Metabolic Changes
Carbohydrate and Lipid Metabolism
Profound changes in carbohydrate and lipid metabolism occur with normal pregnancy, and these changes are important for fetal development. This section summarizes some of these changes in order to provide a framework for information on disturbed insulin and lipid metabolism in preeclampsia.
Pregnancy results in remarkable maternal metabolic adjustments that optimize nutrient availability for the growing fetal-placental unit. Two metabolic stages of pregnancy can be distinguished, the first roughly corresponding to the first two-thirds of pregnancy when fetal growth is limited and differentiation and organogenesis are dominant, and the second occurring during the last third of pregnancy when fetal and placental growth accelerate ( Table 7.3 ). Maternal metabolism is predominantly anabolic during the first two trimesters, with storage of a greater proportion of nutrients, as evidenced by accumulation of maternal fat mass. At this stage, maternal glucose tolerance is normal or slightly increased compared to non-pregnant women, and insulin sensitivity in peripheral tissues and hepatic glucose production is normal. By late pregnancy, however, the mother’s metabolism switches to a more catabolic state to provide the fuels needed by the fetus. Because glucose is the preferred fuel of the fetus, a state of modest insulin resistance normally develops during the last half of pregnancy to augment maternal plasma glucose, lipid and amino acid concentrations for diffusion across the placenta.
Normal Pregnancy | Preeclampsia | |||
---|---|---|---|---|
Early | Late | Early | Late | |
Maternal Metabolism | Anabolic | Catabolic | Anabolic | Catabolic |
Glucose tolerance | ∼ | ↓ | ∼↓ | ↓↓ |
Insulin sensitivity | ∼ | ↓ | ∼↓ | ↓↓ |
Free fatty acids | ↑ | ↑↑ | ↑↑ | ↑↑↑ |
Triglycerides | ↑ | ↑↑ | ↑↑ | ↑↑↑ |
Cholesterol | ∼ | ↑ | ∼↑ | ↑ |
One result of the mobilization of maternal adipose lipid depots during the second half of pregnancy is a striking increase in circulating FFA concentrations. Human placental lactogen, which reaches very high levels during late gestation, appears to contribute to the increase in FFAs by its direct lipolytic action on adipose tissue. Suggesting a feed-forward process, the increasing levels of plasma FFAs may contribute to the gestational insulin resistance. TNF-α and leptin probably also foster gestational insulin resistance.
Much of the glycerol and FFA released from fat are taken up by the liver and re-esterified for synthesis of very low-density lipoprotein (VLDL) triglycerides ( Fig. 7.1 ). Higher estrogen concentrations and decreased hepatocyte beta-oxidation also lead to increased hepatocyte VLDL production. By term, plasma triglycerides increase by 50–300% over non-pregnancy levels, at which time higher triglycerides are found not only in VLDL but also in intermediate-density lipoprotein (IDL), low-density lipoprotein (LDL) and high-density lipoprotein (HDL). Lipoprotein lipase, tethered to the luminal side of capillaries and arteries of extrahepatic tissues, hydrolyzes lipoprotein triglycerides to produce FFA and monoacylglycerol ( Fig. 7.1 ). These lipolysis products are predominantly taken up by tissue locally to meet the needs of that tissue. This promotes triglyceride clearance from the circulation. However, adipose tissue lipoprotein lipase activity decreases substantially during the last third of pregnancy due to insulin resistance and other hormonal influences. The result is a decrease in the rate of removal of triglyceride-rich lipoproteins from the circulation. Therefore, both increased production and decreased removal contribute to the dramatic late gestational increase in postprandial and fasting triglycerides.
Both circulating FFAs and glycerol, but not triglycerides, cross the membranes of the placental syncytiotrophoblast to the fetal circulation. However, triglyceride-rich lipoprotein particles are an important source of essential fatty acids for the fetus. A variant of lipoprotein lipase in the trophoblast microvillous membrane appears to be relatively unresponsive to insulin; this enzyme makes FFAs available by hydrolyzing lipoprotein triglycerides. An unusually low activity of placental lipoprotein lipase was observed in preterm pregnancies complicated by intrauterine growth restriction (IUGR). This suggests that syncytiotrophoblast lipoprotein lipase activity contributes to FFA delivery to the fetus and, as a consequence, fetal growth. Membrane-bound and cytosolic fatty acid binding proteins are important in determining the direction and net flux of fatty acids toward the fetus. After uptake by trophoblast cells, fatty acids are re-esterified to provide a fat reservoir. After intracellular hydrolysis releases fatty acids to fetal plasma, they bind α-fetoprotein and are transported to the fetal liver.
A direct relationship between maternal triglycerides and birthweight has been found in humans. Obesity is strongly correlated with elevated triglyceride and VLDL in pregnancy, both in the mother and in the macrosomic newborn. Severe correction of maternal hypertriglyceridemia (as with hypolipidemic drugs), however, has negative effects on fetal growth and development.
Maternal cholesterol levels increase during pregnancy, with a roughly 50% rise over prepregnant levels by term. Maternal cholesterol is important for the fetus during early pregnancy but its importance lessens by late pregnancy owing to the ability of fetal tissues to synthesize cholesterol. HDL cholesterol increases by week 12 of pregnancy in response to estrogen and remains elevated for the rest of pregnancy. Reversal of the physiologic hyperlipidemia of pregnancy begins within hours of delivery and is essentially complete by 6 to 10 weeks postpartum.
Maternal Weight Gain in Pregnancy
Maternal weight gain during pregnancy is anticipated and attributable to the fetus, placenta, amniotic fluid, as well as the physiologic uterine hypertrophy, increase in blood and fluid volume, breast enlargement and fat stores. Excessive weight gain is primarily related to the increase in maternal adiposity. Prepregnancy obesity is linked to a host of perinatal problems related to adverse outcome (gestational diabetes, cesarean delivery, infant macrosomia, preterm birth, neural tube and other birth defects, antepartum stillbirth, preeclampsia, eclampsia). The risk of perinatal death more than doubles with maternal obesity. Recognizing that a greater percentage of women are entering pregnancy overweight or obese and that many are gaining too much weight during pregnancy, the Institute of Medicine convened a task force to re-evaluate the 1990 guidelines for weight gain in pregnancy. Numerous studies published between 1990 and the 2009 guidelines also demonstrated that prepregnancy BMI above normal values (overweight and obese) as well as excessive gestational weight gain during pregnancy are associated with insulin resistance, gestational diabetes, preeclampsia, cesarean section, large for gestational age infants and breastfeeding problems. An important consideration for the 2009 guidelines ( Table 7.4 ) was not only the welfare of the infant, but also the health of the mother given the substantial research that prepregnancy weight and gestational weight gain impact pregnancy outcomes as well as the short- and long-term health of the mother. Major differences in the 2009 guidelines compared to those published in 1990 are that the weight gain recommendations are based on World Health Organization BMI categories and that a relatively narrow range of weight gain is recommended for obese women during pregnancy. In addition, optimizing BMI prior to conception is emphasized ( Table 7.4 ).
Prepregnancy BMI | BMI (kilogram/meters 2 ) | Total Weight Gain Range (pounds) | Rates of Weight Gain in the Second or Third Trimester (mean range in pounds/week) |
---|---|---|---|
Underweight | <18.5 | 28–40 | 1 (1–1.3) |
Normal weight | 18.5–24.9 | 25–35 | 1 (0.8–1) |
Overweight | 25.0–29.9 | 15–25 | 0.6 (0.5–0.7) |
Obese (includes all classes) | ≥30.0 | 11–20 | 0.5 (0.4–0.6) |
Additional evidence published since the release of the new guidelines has confirmed the association of excessive gestational weight gain with adverse clinical outcomes including hypertensive disorders and gestational diabetes. In an assessment of pregnancy outcomes in nulliparous women with weight gain above or below the 2009 IOM guidelines, excess weight gain occurred in 73% of women and was associated with an increased risk of hypertensive disorders, cesarean delivery, and large for gestational age neonates. There were no consistent associations with insufficient weight gain and adverse outcomes in that study. Gestational weight gains below, within, or above the IOM guidelines were all associated with significant postpartum weight retention, but this retention was substantially higher 15 years after delivery in women with gestational weight gain above the recommendations. Of importance is that weight gain during pregnancy is associated with a higher accrual of abdominal or central adiposity over time. McClure and colleagues studied women 4 to 12 years after delivery and also found that excessive gestational weight gain was associated with abdominal adiposity. Fraser and colleagues recently reported associations between excessive gestational weight gain and waist circumference and risk of central adiposity in mothers 16 years after pregnancy. Visceral adiposity is more metabolically active and has been linked to a more adverse cardiometabolic profile.
Preeclampsia and Metabolic Syndrome
Obesity and Insulin Resistance
As previously noted, a strong relationship exists between prepregnancy obesity and preeclampsia. Obesity and overweight contribute to the risk of both preterm preeclampsia and severe preeclampsia, a finding with potentially profound public health implications. Analysis of the National Hospital Discharge Survey public-use data set shows an increase in the United States of almost 25% in the rate of preeclampsia during the 18-year period ending in 2004. Much of this rise might reflect the growing “epidemic” of obesity. Stone et al. compared 70 women with severe preeclampsia to 18,000+normotensive controls, all without history of prepregnancy hypertension, and observed that severe obesity and a history of preeclampsia were the only outstanding risk factors for development of severe preeclampsia. Eskanazi et al., using similar standard criteria for severe cases and controls, noted that, regardless of parity, women with severe preeclampsia were more likely to have had a high prepregnancy BMI (adjusted OR 2.7; 95% CI 1.2 to 6.2).
Analysis of the Danish birth cohort (41,000 nulliparas and 29,000 multiparas) revealed that, whether primiparous or multiparous, women with obesity are overrepresented in cases of both mild preeclampsia and severe or early-onset preeclampsia ( Fig. 7.5 ). Data from the USA Collaborative Perinatal Project (a prospective, cohort study of 19,053 black and 19,135 white women delivering from 1958 to 1964) similarly support a clear relationship between prepregnancy BMI and the risk of severe and mild preeclampsia in both white and black races. The results of both studies show a dose-response relationship between prepregnancy BMI, even in the nonobese BMI range, and risk of both mild and severe (including preterm) preeclampsia.

Women with abnormally low BMI in the first trimester are significantly less likely to develop preeclampsia or gestational hypertension than women with normal BMI. However, excessive gestational weight gain during pregnancy is associated with an increased risk of gestational hypertension and preeclampsia regardless of prepregnancy BMI, with the risk being higher with higher BMI. There must be some reservation about the relationship between gestational weight gain and preeclampsia because of the increase in fluid retention characteristic of preeclampsia that would increase weight independent of adipose accumulation. The results of a large, prospective population-based study showed that weight gain during the inter-pregnancy interval is strongly associated with risk of major maternal and perinatal complications, including preeclampsia, independent of whether women are overweight or lean. These striking findings suggest that adipose accrual, even below the obese range, progressively increases the risk of developing preeclampsia. One would assume that central adiposity is the culprit.
Most women with preeclampsia are of normal weight but as a group these same women gain significantly more weight over subsequent decades postpartum than women who did not experience preeclampsia, suggesting that preeclampsia unmasks a latent predisposition to obesity (obesogenic phenotype). If this is the case, the population attributable risk (PAR) of preeclampsia as a result of adiposity, estimated in the Pittsburgh population as 20 to 30% for primiparous women (unpublished data), might be the “tip of the iceberg.”
Gestational insulin resistance is accentuated in women with preeclampsia and this difference can be demonstrated in pregnant women weeks before clinically evident preeclampsia, and in postpartum women years after a preeclamptic pregnancy. This suggests that insulin resistance is an important underlying risk factor for preeclampsia, as it is for cardiovascular disease.
By analogy to the relationship of cardiovascular disease or Type 2 diabetes to obesity and insulin resistance, it is unlikely that insulin resistance is the only metabolic change in obesity responsible for the increased risk of preeclampsia. Both lean and obese individuals who are insulin-resistant are at increased risk of cardiovascular disease and Type 2 diabetes. In obese and lean individuals with equivalent insulin resistance, however, Type 2 diabetes still develops more frequently in the obese, indicating that the effect of obesity to increase morbidity is due to more than insulin resistance. Using animal models investigators have shown that adipose accrual reduces vasorelaxation to endothelium-dependent vasodilators before the development of (and thus independently from) insulin resistance, but in association with increased tissue oxidative stress and oxidative destruction of the vasodilator nitric oxide, the latter evidenced by tyrosine nitration in vascular tissue. Therefore, there may be effects of adiposity on the endothelium that are independent of insulin sensitivity. There are data that have led to the hypothesis that factors more prevalent in obese but also relevant to nonobese populations – inflammation, thrombosis, abnormalities of the renin-angiotensin system, abnormalities of angiogenesis, poor nutrition – predispose the gravida to develop preeclampsia.
Obesity promotes endothelial dysfunction in the setting of pregnancy. Obese gravid women have poorer endothelial function on average compared to lean pregnant women; endothelial-dependent vasodilatation was lower at each trimester (51%, 41% and 39%, respectively) in obese women (without previous history of cardiovascular or metabolic disease) than lean women.
Inflammation
An important advance in obesity research came with the understanding that obesity is characterized by chronic, low-grade inflammation. The term “metaflammation” was coined in 2006 to describe this metabolically triggered low-grade inflammation orchestrated by metabolic cells in response to excess nutrients and energy. It is increasingly evident that inflammation contributes to the development of insulin resistance, dislipidemia, oxidative stress, and cardiovascular problems associated with obesity. Obesity is associated with both local adipose inflammation and systemic inflammation. Obese individuals on average manifest increased plasma concentrations of several inflammatory markers and signaling cytokines, including IL-6, TNF-α, and C-reactive protein. Peripheral blood mononuclear cells from obese patients are in a proinflammatory state.
Intriguingly, macrophages and adipocytes share a distant evolutionary origin in the fat body present in insects. Obesity with enlarged fat cells is associated with increased numbers of macrophages in the adipose tissue surrounding individual adipocytes. Adipocyte progenitor cells (preadipocytes), especially proliferating preadipocytes, have phagocytic capacity under appropriate stimulation and thus appear to be macrophage-like cells. Like adipocytes, macrophages are extraordinarily adept at taking up and storing lipids, and share genes and fat metabolism proteins including fatty acid transporters (i.e., fatty acid binding protein 4 (FABP4, also known as A-FABP or aP2)) and peroxisome proliferator-activated receptor-gamma (PPAR-γ), the latter a key nuclear receptor protein that upregulates genes that mediate fatty acid uptake and trapping by adipocytes.
Chronic inflammation is implicated in the mechanisms underlying the adiposity-preeclampsia relationship. Obese or diabetic women are more likely than nonobese women to enter pregnancy in a subclinical inflammatory state. Prepregnancy obesity in the human results in placental over-accumulation of macrophages and increased expression of the proinflammatory cytokines IL-1, TNF-α, and IL-6 in the placenta. The strong linear relation between prepregnancy BMI and preeclampsia risk is partly accounted for by inflammation (using C-reactive protein as a marker) and dyslipidemia (triglycerides). Analyses of correlative data, however, reveal that inflammation in preeclampsia is not always linked to the MetS or insulin resistance.
There is evidence of enhanced neutrophil activation during preeclampsia including: (1) greater basal and fMLP-induced superoxide production, (2) increased CD11b expression basally in neutrophils from women with preeclampsia compared to normal pregnancies, and (3) increased neutrophil elastase. Information is lacking, however, on the impact of obesity on these abnormalities.
The transit of neutrophils and monocytes through the placental intervillous space does not normally result in their activation, but trans-placental neutrophil and monocyte activation does appear to occur during preeclampsia. Trophoblast debris shed into the maternal circulation, and maternal neutrophils activated during transit through the placenta, may induce a widespread systemic vascular inflammatory response. Furthermore, the systemic vasculature of women with preeclampsia evidences increased infiltration of neutrophils compared to normal non-pregnant women or women with uncomplicated pregnancy (matched for BMI).
Inflammatory Cytokines
C-reactive protein (CRP), TNF (tumor necrosis factor)-α and IL (interleukin)-6 are all increased in the circulation of women with preeclampsia.
CRP , an acute-phase reactant and inflammatory mediator produced by the liver as well as adipose tissue, is elevated in obese individuals and associated with cardiovascular morbidity. CRP is also elevated in early pregnancy in women who later develop preeclampsia. Studies from our group indicated that CRP has a stronger association with preeclampsia among obese women than triglycerides and together inflammation and triglycerides could account for approximately one-third of the effect of BMI on preeclampsia risk.
TNF-α is produced in adipose tissue both as a locally acting agent by adipocytes as well as a circulating cytokine by infiltrating macrophages of adipose tissue. TNF-α is associated with insulin resistance, oxidative stress, and endothelial dysfunction. Concentrations are higher in obese individuals. TNF-α is increased in preeclampsia; the likely source is adipose tissue as placental mRNA is not increased. Although appealing as a mechanism by which obesity might increase the risk of preeclampsia, plasma TNF-α concentrations were not observed to be higher in obese pregnant women than in nonobese pregnant women.
IL-6 , another potent inflammatory mediator, is associated with obesity, insulin resistance, vascular damage, and later-life cardiovascular disease. IL-6 produced by adipose tissue is estimated to account for 30% of circulating IL-6. Circulating concentrations are higher in obese individuals as well as in women with preeclampsia, indicating a potential relationship.
Adipokines
Leptin and adiponectin, two well-recognized cytokines produced by adipose tissue, affect metabolism and have been linked to cardiovascular disease. There are a growing number of adipokines that may have implications for metabolic syndrome; a few that may have relevance to preeclampsia are briefly discussed below.
Leptin is secreted by adipocytes in positive relation to adipocyte size and obesity. It is important for appetite regulation and energy expenditure. The increased concentrations of leptin in obesity are associated with leptin resistance. Leptin may contribute to obesity-associated hypertension through stimulation of central sympathetic pathways. Leptin has structural similarity to proinflammatory cytokines IL-6 and IL-12 and has cytokine-like functions to activate endothelial cells and monocytes. Leptin is produced by the placenta with message concentration approaching that present in adipose tissue. Maternal plasma leptin concentrations rise gradually to 32 weeks and thereafter decline slightly in women with uncomplicated pregnancy followed by a drastic decline postpartum. Leptin’s functional significance during pregnancy may be related to nutrient availability and delivery to the fetus, including amino acids, by upregulating placental System A amino acid transport activity. Leptin concentrations are also positively correlated with fetal weight and insulin resistance. Leptin concentrations in women destined to develop preeclampsia are reportedly higher at 20 weeks gestation and rise markedly from 32 weeks as the manifestations of preeclampsia develop. The increased leptin in the maternal circulation is thought to be of placental origin. Nonetheless, even in late pregnancy when leptin is highest, its concentration still correlates with maternal BMI.
Adiponectin is also produced in adipose tissue and has insulin-sensitizing effects. It increases free fatty acid oxidation and is associated with reduced serum free fatty acids, triglyceride, and glucose concentration. It also has anti-inflammatory effects by inhibiting TNF-α-induced monocyte adhesion and adhesion molecule expression. Circulating adiponectin concentrations are lower in obese individuals and inversely correlated with cardiovascular risk. During pregnancy, adiponectin levels reach their lowest concentrations in the third trimester when maternal insulin resistance is greatest and these low concentrations are accentuated further with gestational diabetes. Concentrations are inversely related to BMI in the third trimester. Our current understanding is that adiponectin is not produced by the placenta. Despite the recognized association of insulin resistance and preeclampsia, the precise relationship between adiponectin and preeclampsia remains unclear. Early studies reported higher adiponectin concentrations in women with preeclampsia. It has since been noted that there are several forms of circulating adiponectin; the high molecular weight oligomers are considered the most active in inducing insulin sensitivity. As of this writing, there is no consensus on the precise relationship of adiponectin concentrations and preeclampsia, as some studies report lower concentrations and other higher concentrations with preeclampsia.
Resistin , also referred to as adipose tissue-specific secretory factor, is secreted primarily by adipocytes and mononuclear cells. Resistin impairs glucose intake by adipocytes, increases glucose concentrations, and promotes insulin resistance. It has also been shown to promote fat deposition and has proinflammatory properties. Although not consistent across all studies, serum concentrations are reported to be higher in obese compared with lean individuals and implicated in insulin resistance and cardiovascular disease. In pregnancy, circulating resistin is present during the first trimester and progressively increases with gestational age. Reports are conflicting with regard to maternal serum resistin with established preeclampsia. Recently, Nanda and colleagues evaluated maternal serum resistin concentrations in the first trimester in normal and pathologic pregnancies and reported higher median resistin concentrations in women who later developed preeclampsia compared to controls but no correlation with maternal weight. The authors suggest that resistin may have a pathogenic role in the development of preeclampsia; clearly, further investigation is needed to understand the significance of this relationship.
Fatty acid binding protein 4 (FABP4) , also known as adipocyte fatty acid binding protein or aP2, is a member of the intracellular fatty acid binding protein family expressed in adipose tissue, macrophages, trophoblasts and endothelium. Fatty acid binding proteins are linked to inflammatory and metabolic pathways; specifically, FABP4 is secreted from adipocytes into the circulation and is positively correlated with BMI, waist circumference, and insulin resistance. Higher baseline levels independently predicted the risk of developing metabolic syndrome over a 5 year period and were positively correlated with carotid intima media thickness in a Chinese population. Recent studies indicate a role in preeclampsia. Fasshauer and colleagues demonstrated higher maternal serum FABP4 concentrations in women with preeclampsia compared to normotensive controls. Scifres et al. compared maternal FABP4 concentrations in the first and second trimesters in women who developed preeclampsia to matched, normotensive pregnant controls. Maternal serum FABP4 levels were elevated before the clinical onset of preeclampsia and this increase occurred independently of maternal BMI. There was a modest univariate correlation between first-trimester BMI and maternal FABP4 concentrations (correlation coefficient 0.7, p <0.01). FABP4 is highly upregulated in the placental basal plate with advancing uncomplicated gestation, and further upregulated in preeclampsia as compared to preterm labor with no evidence of inflammation. Placental FABP4 is localized to invasive cytotrophoblasts. Expression of FABP4 is upregulated by hypoxia in primary human trophoblasts, suggesting that upregulation of this protein supports fat accumulation in the hypoxic placenta. Further investigation is needed to elucidate the precise role of FABP4 in preeclampsia.
A number of other novel adipokines such as visfatin, retinol binding protein 4 and vaspin have been identified; however, further study is needed to understand their physiologic role in normal and pathologic pregnancies.
Dyslipidemia
The dyslipidemia that occurs with preeclampsia closely resembles the dyslipidemia of the MetS and, in many ways, it represents an accentuation of normal pregnancy changes. Heightened gestational insulin resistance, abnormally increased concentrations of TNF-α (an inhibitor of lipoprotein lipase), and increased human placental lactogen are thought to contribute to this dyslipidemia.
The high prevalence of metabolic complications among obese pregnant women suggests that obesity adversely affects the usual metabolic adjustments in fuel metabolism that accompany pregnancy. Hyperlipidemia is exaggerated in obese pregnant women, in the form of higher FFA, lower HDL cholesterol, and higher triglycerides packaged into VLDL, LDL, and HDL. Meyers and colleagues reported that obese women commence pregnancy with higher plasma triglycerides, reach the same maximum, and then return to higher post-natal levels than normal weight women. Importantly, the atherogenic LDL subfraction measured in the third trimester was two-fold higher in obese women compared to normal weight controls. Recent work confirms prior findings of higher baseline cholesterol, LDL, and triglycerides, but did not demonstrate an effect of excessive gestational weight gain on the rate of change in lipid profiles in either normal or overweight/obese pregnant women. More work is needed, however, to delineate the impact of prepregnancy obesity and gestational weight gain on the metabolic milieu of pregnancy.
Mean plasma triglyceride and free fatty acid concentrations increase about two-fold on average in women with preeclampsia relative to women with uncomplicated pregnancy ( Fig. 7.6 ). About one-third of women with preeclampsia develop plasma triglyceride values above 400 mg/dL, greater than the 90th percentile measured in randomly selected women at 36 weeks gestation. This difference reflects a pronounced increase in triglyceride-rich lipoproteins (especially VLDL 1 ). The hypertriglyceridemia of preeclampsia is accompanied by a decrease in cardioprotective HDL cholesterol relative to normal pregancy. Not all women with preeclampsia become dyslipidemic, and some women with gestational dyslipidemia have normal outcome, fitting with a multi-factorial syndrome.

Consistent with a pathogenic role, dyslipidemia becomes evident during the first and second trimester, antedating the clinical detection of preeclampsia. Higher mean concentrations of the major FFAs (oleic, linoleic, palmitic) are found as early as 16 to 20 weeks gestation in women who later develop preeclampsia compared to women who do not.
Fasting triglycerides are elevated at earliest reported measurement (10 weeks of gestation) and the difference from controls increases with advancing gestation. Early hypertriglyceridemia is mainly associated with increased risk of early-onset preeclampsia (preeclampsia developing before 36 weeks of gestation). HDL-cholesterol is reduced at earliest measurement (20 weeks gestation) and then throughout gestation in women who later develop the disease. Decreased HDL may result from increased triglycerides since the two are metabolically linked.
Total cholesterol and LDL cholesterol levels are not typically elevated during preeclampsia compared to normal pregnancy, nor are they generally a component of the MetS. Nevertheless, women with raised total cholesterol during the first trimester are at increased risk of preeclampsia. Furthermore, high levels of LDL-cholesterol, or non-HDL-cholesterol, or triglycerides occurring 4 to 5 years before first pregnancy are each associated with significantly increased odds of developing preeclampsia. This is consistent with the hypothesis that pre-existing dyslipidemia, perhaps beyond the umbrella of MetS, contributes to development of preeclampsia.
Free Fatty Acids and Vascular Dysfunction in Preeclampsia
When sequestered by albumin or ceruloplasmin, copper is usually redox-inactive (incapable of producing reactive oxygen species). On average, however, plasma samples from women with preeclampsia display an elevated endogenous redox-cycling activity of copper that is inhibited by copper chelators. This activity may result from increased free fatty acids in the circulation ( Fig. 7.3 ). Circulating FFAs are complexed with albumin. The molar ratio of plasma FFA to albumin is two- to three-fold greater in preeclampsia (up to 2.5:1) than normal pregnancy (0.8:1 to 1:1). The excess binding of FFA to albumin in preeclampsia is accompanied by conformational change in albumin such that the copper bound to albumin becomes redox-active. The reactive oxygen species generated after excessive FFA binding to Cu-albumin are capable of destroying nitric oxide. Vitamin C actually accelerates this free radical redox cycling process, fueling the generation of reactive oxygen species ( Fig. 7.3 ).
When endothelial cells are exposed to preeclampsia plasma or 2.5:1 molar ratio FFA:human serum albumin complex, they take up the FFA in proportion to extracellular concentration; this results in a dramatic increase in cytoplasmic lipid droplets (also called cytoplasmic lipid inclusions). The accumulation of cytoplasmic lipid droplets is associated with decreased secretion of the vasodilator prostaglandin PGI 2 (prostacyclin) and a reduction in the nitric oxide-induced cGMP level. Lipid droplets have made recent headlines as dynamic, complex structures that are major contributors not only to lipid homeostasis but also to diverse cellular functions. Beyond adipocytes and macrophages, all cell types observed to date have the ability to generate cytoplasmic lipid droplets in response to elevated FFA, and to metabolize and disperse these droplets when conditions are reversed. The role of lipid droplets in the vascular pathology of obesity and preeclampsia should be fertile ground for research.
Triglycerides and Vascular Dysfunction in Preeclampsia
Both VLDL and atherogenic triglyceride-rich lipoprotein remnant particles are increased in women with preeclampsia. Triglyceride-rich lipoproteins have prothrombotic activity. Human VLDL is toxic to human umbilical vein endothelial cells in culture and this toxicity is prevented by the addition of normal human serum albumin, but not fatty acid-laden human serum albumin, to the culture medium.
Several prospective studies have shown that a preponderance of small-diameter LDL is associated with the MetS and increased risk of coronary heart disease. Measured by nondenaturing polyacrylamide gradient gel electrophoresis, the rise in plasma triglyceride during normal pregnancy is accompanied by a progressive shift from predominantly large-diameter LDL to predominantly intermediate and small-sized LDL, with reversal by 6 weeks postpartum. Three studies have reported that the average diameter of LDL particles is further decreased in preeclampsia relative to normal pregnancy. LDL isolated from plasma of women with preeclampsia is more susceptible to oxidation upon exposure to exogenous free radicals than normal pregnancy LDL. This is consistent with a sub-type of LDL capable of impairing vascular function.
Angiogenic Factors
The balance of antiangiogenic factors (soluble Flt-1 (sFlt-1) and soluble endoglin) and proangiogenic factors (placental growth factor (PlGF) and vascular endothelial growth factor (VEGF)), are altered in preeclampsia compared with normal pregnancy even weeks prior to the onset of the clinical condition. PlGF concentrations are lower in preeclamptic women, probably due to the higher circulating concentration of sFlt, which binds and inactivates PlGF and VEGF. Soluble endoglin serves as an antagonist to the angiogenic factor TGF-β and is increased with and prior to preeclampsia (see Chapter 6 for a detailed discussion). In the non-pregnant population, obesity is associated with increased circulating angiogenic factors including VEGF, which may represent excess from adipose tissue production, particularly visceral fat. Due to the very high circulating concentrations of sFlt-1 in pregnancy, free VEGF is essentially absent while free PlGF is measurable. PlGF concentrations are significantly lower in both overweight and obese pregnant women compared to normal-weight controls, and this difference is maintained in both women who develop preeclampsia and those who do not. Others have reported that PlGF, sFlt-1, and soluble endoglin are all inversely correlated with BMI. In contrast, Faupel-Badger and colleagues found higher sFlt-1 and sFlt-1/PlGF ratio in women with higher BMI, suggestive of an antiangiogenic milieu. Although inconsistent, the angiogenic milieu associated with obesity may have implications in the development of preeclampsia.
Uric Acid and ADMA
Uric Acid
Uric acid, the end-product of purine metabolism in humans, is an independent risk marker (and may be an important risk factor) for cardiovascular disease, particularly in women. Serum uric acid concentrations are frequently elevated in obese patients with the MetS, and concentrations increase with the number of components of MetS. The hyperuricemia of MetS has been related to increased uric acid production or decreased renal uric acid excretion, the latter mediated by enhanced proximal tubular sodium reabsorption and hyperinsulinemia.
Hyperuricemia in rats (by pharmacologic inhibition of the enzyme uricase) results in hypertension and renal injury, mediated by stimulation of the renin-angiotensin system and inhibition of nitric oxide synthase. Hyperuricemia may also activate the immune response; uric acid release from damaged cells during infection enhances macrophage activation. Uric acid released from damaged cells following an acute ischemia-reperfusion event in mice results in mobilization of endothelial progenitor cells into the circulation; conversely, chronic elevation of uric acid in mice blunts ischemia-induced endothelial progenitor cell mobilization and could thereby limit endothelial repair.
Most women with preeeclampsia are hyperuricemic, typically defined as greater than one standard deviation above the norm for gestational age. Average uric acid values are higher as early as 10 weeks of gestation in groups of women who later develop preeclampsia compared to women whose pregnancies remain uncomplicated. Women with preeclampsia and hyperuricemia have a more severe form of preeclampsia with an increase in indicated preterm births and smaller infants for gestational age. Adjusting for differences in glomerular filtration by serum creatinine accounts for part, but not all, of the increase in serum uric acid among women with preeclampsia, suggesting that hyperuricemia is not entirely due to decreased glomerular filtration, and changes in tubular urate handling and/or uric acid production may play a role. (See also Chapter 16 .) More than a decade ago it was suggested that hyperuricemia be “revisited” as a candidate player in the pathogenesis of preeclampsia; this concept may be gaining momentum. The elevated uric acid that many women with the MetS bring into pregnancy might contribute significantly to gestational hyperuricemia, and it seems biologically plausible that gestational hyperuricemia would adversely affect placental and maternal vascular function.
Asymmetric Dimethylarginine
Asymmetric dimethylarginine (ADMA) is an endogenous inhibitor of nitric oxide synthase (NOS). Plasma ADMA concentrations are elevated in association with various components of the MetS, including obesity. The mechanism that mediates this association, however, is unknown. There is evidence, however, that dimethylarginine dimethylaminohydrolase, the enzyme that metabolizes ADMA to citrulline and methylamines, is sensitive to inactivation by reactive oxygen species.
ADMA may be a major cause of impairment of the nitric oxide-mediated response in several disease states by its ability to compete with l -arginine, the substrate for NOS. ADMA may also “uncouple” endothelial NOS such that molecular oxygen becomes the substrate for electron transfer rather than the guanidino nitrogen of l -arginine. Under these conditions, endothelial NOS generates superoxide anions which in turn can oxidize and thus destroy nitric oxide, and thereby induce additional endothelial dysfunction. There is substantial evidence that ADMA regulates vascular resistance in humans. ADMA induces endothelial dysfunction and impaired angiogenesis in vivo and in vitro , and is closely correlated with coronary disease and acute coronary events. ADMA has been coined an “über marker,” as increased ADMA appears to reflect the summative effects of various risk factors (age, hypertension, obesity, insulin resistance, hypertriglyceridemia, hypercholesterolemia, hyperhomocysteinemia) on endothelial health.
Plasma ADMA concentrations are greater in women with preeclampsia compared to uncomplicated pregnancy, and interestingly this difference manifests before clinical evidence of preeclampsia. Our recent findings indicate that plasma ADMA concentrations are greater in obese pregnant women compared to lean pregnant women, and ADMA is elevated during the first half of pregnancy more often in obese than lean women who later develop preeclampsia (unpublished data).
Lifestyle Factors
In addition to metabolic and inflammatory factors, lifestyle factors such as diet, sleep disorders, and physical activity are associated with obesity and cardiovascular disease. Many of these factors are also implicated in preeclampsia, thus raising the possibility of a mechanistic link through which obesity may increase the risk of preeclampsia. A detailed discussion is beyond the scope of this chapter, but is reviewed extensively in Chapter 3 .
Later-Life Cardiovascular Risk (also see Chapter 3 )
Clinical risk factors common to both coronary artery disease and preeclampsia include adiposity, hypertension, diabetes mellitus, systemic lupus erythematosus, polycystic ovarian syndrome, lack of physical activity, and family history of heart disease. Although the hypertension and proteinuria that define preeclampsia typically resolve within a few days after delivery, women with a history of preeclampsia are at significantly increased risk of developing hypertension and coronary artery disease in later years. A study of 37,000 women who delivered in Jerusalem showed that women with a history of preeclampsia have a two-fold increased risk of death (mainly due to cardiovascular disease) compared to women with a history of normal pregnancy. The increase in cardiovascular disease-related mortality becomes evident about 20 years after delivery in women with a history of non-recurrent preeclampsia (women whose other pregnancies were without preeclampsia), substantially later than women with a history of recurrent preeclampsia. These findings do not mean that every preeclampsia survivor is destined to develop heart disease, but, rather, that a history of preeclampsia may identify a population at increased risk. On the other hand, women with a history of normal pregnancy may have lower blood pressures on average, and may be less prone to develop cardiovascular disease, than the general (at-large) female population.
Endothelial dysfunction is consistently apparent years after preeclamptic compared to normotensive first pregnancy. In a study of non-pregnant women 1 year postpartum, by Agatisa et al., the response to mental stress-induced forearm blood flow after venous occlusion was influenced both by pregnancy history and by presence or absence of obesity. Nonobese women with prior uncomplicated pregnancy had twice the percent increase in forearm blood flow exhibited by obese women with prior preeclampsia; intermediate between these two groups, nonobese women with prior preeclampsia had only slightly poorer vasodilatory function than obese women with prior uncomplicated pregnancy.
A study was performed comparing the remote cardiovascular outcomes of postmenopausal Icelandic women who had had eclampsia (the convulsive stage of preeclampsia) to women who had experienced only uncomplicated pregnancies. Icelandic women in both arms were matched for date of birth, age at pregnancy, and parity. The groups did not differ by average BMI but nevertheless the previous-eclampsia group showed significant elevations in plasma apolipoprotein B (an index of total atherogenic lipoprotein particles) and a reduction in the predominant diameter of LDL particles. The combination of high apolipoprotein B and small-sized LDL commonly accompanies the MetS in the general population, and it confers an especially high cardiovascular risk. The Icelandic postmenopausal women with a history of eclampsia were also distinguished from their controls by three-fold elevations in median C-reactive protein, an inflammation marker linked to the MetS. The Icelandic women with prior eclampsia clustered into two evenly populated subgroups, those with high-risk C-reactive protein values and those without ( Fig. 7.7 ). Although these eclampsia subgroups did not differ by BMI, the prior-eclampsia subgroup with high-risk C-reactive protein values evidenced significant increases in fasting insulin, HOMA values, systolic blood pressures, and decreased HDL cholesterol compared to controls, whereas the prior-eclampsia/low C-reactive protein subgroup did not. This suggests a close link between dyslipidemia and inflammation in women with a history of eclampsia.

Full access? Get Clinical Tree
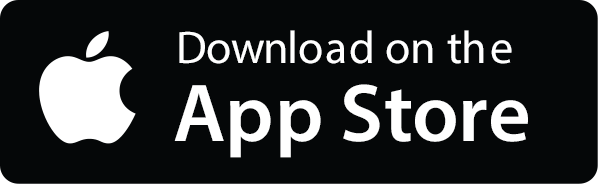
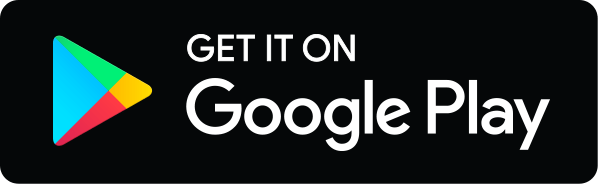