Metabolic Syndrome and Obesity
Metabolic Syndrome
The metabolic syndrome (MetS) was originally described in the adult population, with the observance of the tendency for the aggregation of a number of cardiovascular risk factors that are associated with increased risk for atherosclerotic cardiovascular disease (ASCVD)1–3 and type 2 diabetes (T2DM).4 A central, albeit not exclusive, factor in the pathogenesis of MetS is insulin resistance.5,6 Other mechanisms that have been thought to play a role include the effect of obesity, increased inflammatory mediators, aggravated oxidative stress, endothelial dysfunction, and elevated cortisol levels. However, the exact definition of MetS has continued to be a center of debate. Only recently has there been harmonization of the definition in the adult population to include cut-off points for fasting glucose, HDL cholesterol, triglycerides, waist circumference, and blood pressure.7 Defining the condition in the pediatric population is a greater challenge, since it needs to incorporate considerations such as criteria across ages, take into account physiologic changes in metabolism throughout the life-cycle, and the requirement to define morphologic scores for truncal obesity. The International Diabetes Federation published its definition of MetS in 2007 as listed in Table 17.1 with the following parameters: (1) waist circumference, (2) triglyceride level, (3) blood pressure, and (4) fasting glucose. Other proposed criteria for MetS are listed on Table 17.1. A further hindrance to unified criteria is that prevalence rates vary greatly from 6% to 39% of the general population.8 The diagnosis of MetS also appears to be unstable as children age.9,10 Nonetheless, there is significant evidence that the presence of MetS and its attendant cardiovascular risk factors in childhood and adolescence are associated with markers for early and subclinical atherosclerosis11–14 and increased risk for the development of T2DM and ASCVD.15,16
Sleep and the Components of the Metabolic Syndrome (see also Chapter 31)
Sleep deprivation and sleep disruption have been observed to have associations with many of the parameters of MetS. In fact, the inclusion of obstructive sleep apnea (OSA) as a component of MetS in the form of syndrome Z has been proposed, because of the strength of the association and evidence of increased risk for ASCVD and DM.17 In the pediatric population, Redline et al. found a sixfold increase in risk for MetS in adolescents who were obese and had sleep-disordered breathing (SDB).18 Both sleep fragmentation19 and obstructive sleep apnea20 have been observed to be associated with insulin resistance. Dysregulation of lipid metabolism and its subsequent improvement have been shown with obstructive sleep apnea. A bidirectional relationship between obesity and disrupted sleep has also been demonstrated in models of artificial sleep disturbance and clinical OSA, as described in later sections.
Insulin Resistance
Insulin resistance has been considered a central mechanism in the increased risk for diabetes and ACVD in MetS. Early studies on sleep deprivation demonstrated a reduction in glucose utilization and insulin sensitivity with acute sleep deprivation.21 Epidemiologic data from cross-sectional studies have also demonstrated an association between short sleep and diabetes;22,23 while prospective studies have supported an increase in incident diabetes with baseline short sleep.24,25 Sleep stage deprivation and fragmentation have been observed to present a similar phenomenon. In one particular SWS deprivation trial that utilized acoustic stimuli, a 90% reduction of SWS over a 3-day period produced approximately a 25% reduction in insulin sensitivity without the expected compensatory rises in insulin secretion.19 The fragmentation and SWS curtailment that was produced during this study can be thought to correspond to what can typically be seen in the context of OSA.
OSA in the adult population has been demonstrated in the Sleep Heart Health Study, a large multicenter population-based study, to be associated with derangement in various aspects of glucose metabolism.26,27 The cross-sectional analysis employed data from fasting serum glucose samples and 2-hour oral glucose tolerance tests to evaluate utilization and responsiveness to a glucose challenge. The study revealed that insulin resistance and glucose intolerance were independently associated with SDB after controlling for age, sex, race, BMI, and waist circumference.27 In a separate sub-analysis involving obese/overweight individuals with a sleep-related breathing disorder (SDB) and non-obese subjects with SDB, those with a SDB had a higher prevalence rate and adjusted odds of having impaired fasting glucose (IFG), impaired glucose tolerance (IGT), IFG and IGT and occult diabetes, after controlling for the same factors.26 Another large cross-sectional study involving over 1000 subjects showed that there was an independent association between OSA and incident diabetes with increasing severity of OSA linked to higher risk of developing diabetes.28 As summarized by Tesali and Ip,29 the majority of adult cross-sectional investigations have shown similar findings with only a few small studies showing contradictory results. Unfortunately, pediatric data has not been as consistent and is plagued by small population sizes. An observational study from de la Eva et al. demonstrated a significant correlation between the apnea–hypopnea index (AHI) and fasting glucose, independent of BMI in an population of obese subjects.30 Unfortunately, other studies failed to show similar associations between SDB and impairment in glucose metabolism.31–33 In a study involving non-obese children, Kaditis et al. found no significant correlation between SDB and insulin resistance, fasting glucose levels or fasting insulin after controlling for BMI.32 In an investigation with both obese and non-obese children, Tauman et al. failed to show significant correlations between AHI, Spo2 nadir, arousal index, and parameters of insulin resistance such as the insulin/fasting glucose ratio (I/G ratio) and the homeostasis model assessment equation (HOMA).33 On the contrary, the same group later published an interventional study with 62 children (37 obese and 25 non-obese) with SDB who underwent adenotonsillectomy that did show an association between parameters of SDB and the I/G ratio, and found a significant improvement in insulin resistance after treatment.34
Dyslipidemia
The current evidence surrounding the relationship between sleep disturbance and dysregulation of lipid metabolism is primarily based on SDB and its accompanying hypoxemia. Much of it is founded on animal model data and theoretical mechanisms, with sparse clinical data partially supportive of a direct relationship. Mechanistically, there is evidence that intermittent hypoxemia stimulates the production of hypoxia-inducible factor-1 in the liver, which in turn activates sterol regulatory element-binding protein-1(SREBP-1) and stearoyl-CoA desaturase-1 (SCD).35 SCD is an important enzyme controlled by SRBEP-1 that is instrumental in triglyceride and phospholipid biosynthesis. In the above-cited study, in was demonstrated in lean mice that there was an increase in total cholesterol, high-density lipoprotein (HDL), triglycerides and phospholipids with intermittent hypoxemia for 5 days. This was not observed in the opposite arm of the study that involved obese mice. In another study involving 4 weeks of intermittent hypoxemia, there was an observed increase in low-density lipoprotein (LDL) levels.36
Cross-sectional data from larger adult studies have demonstrated a tendency for a decrease in HDL cholesterol and an increased in triglycerides with the presence of SDB.37–40 However, this is inconsistent, with a similar studies showing no significant association.41–44 In the pediatric population, Tauman et al.33 examined a convenience sample of 135 children (64 moderate to severe OSA, 56 mild OSA and 16 controls) with the complaint of snoring. In their study, they failed to demonstrate a difference in those with mild sleep apnea, moderate to severe sleep apnea, and matched controls, in terms of total cholesterol, triglycerides, HDL and LDL cholesterol. There have also been a number of interventional studies in adults and children examining the effect of the treatment of obstructive sleep apnea on profiles of cholesterol metabolism. Gozal et al.34 examined the response on the lipid profile of children with OSA after having an adenotonsillectomy. The study involved 62 individuals (35 obese and 27 non-obese) and demonstrated a significant reduction in total cholesterol and LDL with a reciprocal increase in HDL cholesterol after a 6–12-month follow-up in both obese and non-obese children. Similar findings were also seen in two adult population studies from Japan that utilized nasal CPAP as the primary treatment for SDB. Chin et al.45 observed a reduction in LDL and reciprocal increase in HDL with 6 months of CPAP therapy for OSA in two groups – a group with significant body weight reduction and a group with no significant reduction in body weight. This was accompanied with a reduction in visceral fat accumulation. In a subsequent study from the same group,46 CPAP therapy for 1 month demonstrated a statistically significant rise in HDL with a reduction in LDL. In a study of 32 individuals in Slovakia, nasal CPAP was associated with a reduction in total cholesterol and triglycerides without evident improvement in HDL.47 In terms of randomized control trials, Robinson et al.48 evaluated the response of the lipid profiles of 220 obese adults with severe sleep apnea, of which 108 individuals were placed on CPAP therapy and 112 were started on sham CPAP. The study demonstrated a significant reduction in total cholesterol as compared to baseline in those subjects on therapeutic CPAP; this difference was not observed with sham CPAP. In comparing CPAP and sham CPAP, the difference between the two groups only closely approached statistical significance (mean difference in change 0.2 mmol/L, 95% CI 20.12 to 0.41, p = 0.06). However, smaller randomized, controlled trials have failed to show significant changes to the lipid profile with therapy.49–51
Hypertension
Elevated blood pressure (BP) has been demonstrated to have contributions from both insulin resistance52 and obesity.53 Hypertension has a strong association with SDB in adulthood, with the condition being present in up to 50% of subjects.54 In children, there are a number of studies that have also reported an association between SDB and elevated systolic and diastolic blood pressure.55–63 However, evidence of the relationship is not extensive, and the magnitude of the blood pressure effect appears smaller.
The development of hypertension in the context of MetS is predominantly described as arising from the collaboration between increased sympathetic nervous system (SNS) tone, insulin resistance and derangement in vascular structure or function. As a parallel, SDB is also associated with a sympathetic predominance64 and has shown an association with insulin resistance, with subsequent reversibility with the treatment of SDB.20,65 Hypoxemia, which is commonly seen in SDB, has been postulated to trigger vascular remodeling and an increase in inflammatory markers as seen in atherosclerosis.66 Affectation of the renin–angiotensin–aldosterone system by hypoxemia in OSA has also been postulated, with animal trials supporting this model.67 Sleep deprivation and/or fragmentation separate from SDB may also play a role in the development of hypertension.
The physiologic profile of BP over a 24-hour period is characterized by a nighttime reduction described as the ‘nocturnal dipping’ phenomenon. This reduction in blood pressure has been associated with an overall increase in markers of vagal tone, and a decrease in sympathetic tone – even with the elimination of the effect of physical activity.68 In comparison, wakefulness is characterized by BP variation being primarily reactive to the physical activity and posture,69 while BP during sleep is controlled by posture and the transition into the stages of sleep.68 Non-rapid eye movement (NREM) sleep is associated with an overall reduction in blood pressure with an accompanying reduction in sympathetic tone, while rapid eye movement (REM) sleep is associated with bursts of sympathetic activation and an overall BP similar to wakefulness.70 With partial sleep deprivation, there is an increase in mean BP during 24-hour BP monitoring, with the finding being more pronounced during nighttime sleep – this normalizes with subsequent sleep. There is also an increase in early morning BP surge with sleep deprivations.71,72 In examining the effects of sleep fragmentation and SWS deprivation, there is evidence of attenuation in nocturnal arterial blood pressure dipping. However, subsequent day ambulatory blood pressure is not significantly elevated.73 The effect of poor sleep efficiency and short sleep on blood pressure has also been investigated in the adolescent population. Javaheri et al. performed a cross-sectional analysis of 238 individuals without obstructive sleep apnea to evaluate the odds of prehypertension (≥90th percentile for age, sex and height) for both short sleep (≤6.5 hours or sleep) and low efficiency sleep (≤85%). After adjusting for sex, body mass index percentile, and socioeconomic status, the odds of prehypertension increased 3.5-fold (95% CI, 1.5–8.0) for low sleep efficiency subjects, and 2.5-fold (95% CI, 0.9–6.9) for short sleepers. In those with a low sleep efficiency, there was an average increase in systolic blood pressure of 4.0 ± 2.1 mmHg.74 Naturally, short sleep and low sleep efficiency can be seen as a surrogate for primary sleep disorders such as obstructive sleep apnea.
As described earlier, obstructive sleep apnea in childhood is associated with the presence of elevated BP.55–63 In a multicenter community-based study including 306 subjects between the ages of 6 and 13 years, children diagnosed with obstructive sleep apnea had significantly higher nocturnal systolic (95% CI, 1.4–10.5) and diastolic (95% CI, 1.4–8.1) BP – this elevation was also noted to be present during wakefulness. There was no significant difference in nocturnal dipping.59 In comparing children referred to a specialty sleep clinic for snoring, Marcus et al. observed that children with obstructive sleep apnea had a higher diastolic BP index than those with primary snoring alone. There was no significant difference in systolic blood pressure index or the magnitude of nocturnal BP dipping.56 In a similar study using 24-hour blood pressure monitoring examining 19 children between the ages of 8 to 12 years who referred to a sleep clinic for suspected OSA, there was a significant increase in diastolic BP and mean BP, and a significant reduction in the degree of BP nocturnal dipping, among those with OSA versus primary snorers. In addition, the odds of non-dipping in nocturnal blood pressure was 6.66 times higher in children with OSA.58 A similar investigation evaluating data from consecutive patients between the ages of 6 and 15 years who were also referred for suspected OSA divided patients into two groups, high-AHI (>5 events/hour) and low-AHI (≤5 events/hour), and demonstrated elevated sleep-related diastolic and systolic blood pressures. In their sub-analysis of obese children, those with a high-AHI had a higher prevalence of hypertension (OR = 6.667; 95% CI, 1.004–44.284). However, the BMI characteristics for the two groups were not described.61 In 90 children between the ages of 4.4 and 18.8 years (mean = 10.7), Reade et al. noted a statistically significant elevation in office-recorded systolic and diastolic BP in the obese. However, they noted that children with obesity and hypertension had significantly higher hypopnea indexes, AHIs, and incidence of OSA, as compared to normotensive obese subjects. The BMI score (ratio of BMI to 95th percentile BMI for age, sex and race) was not significantly different between their hypertensive and normotensive obese subjects.62 The two latter studies suggest that obesity and OSA may have a synergistic effect in causing elevations in diurnal blood pressure. In supporting the hypothesis that OSA has a causal relationship in the development of hypertension, studies have demonstrated a significant reduction in blood pressure in subjects with OSA.75–77 Apostolidou et al. evaluated the effect of adenotonsillectomy (AT) on blood pressure in 58 subjects with OSA and 17 controls who underwent AT for recurrent tonsillitis or otitis media. In subjects who were able to attain an AHI ≤1 event per hour, there was a significant improvement in diastolic BP (p = 0.002).75 In a retrospective study involving 44 children with OSA who had undergone AT, 24-hour BP monitoring demonstrated a significant reduction in diastolic BP load (percentage of blood pressure readings above the 95th percentile for age, sex and height) in the overall analysis. In sub-analysis of 10 subjects who were noted to be hypertensive, there was a significant reduction in nocturnal systolic and diastolic BP.76 Unfortunately, a recent meta-analysis from Friedman et al. has demonstrated only a 59.8% success rate in the treatment of OSA with AT.78 Furthermore, AT is not as effective in the treatment of OSA in obese children and therefore likely to be less effective in the context of MetS.34,79,80
Obesity in the Context of the Metabolic Syndrome
Obesity has been associated with elevations in blood pressure,81 insulin resistance and impaired glucose tolerance,82–84 inflammatory markers,85–87 elevated triglycerides and low HDL,83 and arterial wall stiffness and endothelial dysfunction.88 Childhood obesity also confers an increased risk of future obesity and insulin resistance,89 further weight gain over expected growth, and risk for development of cardiovascular risk factors as young adults.90 In particular, visceral fat has more adverse health outcomes in comparison to BMI or subcutaneous fat and offers an increase in the odds of developing MetS.91 Although obesity has been demonstrated to have a larger role in the development of insulin resistance in comparison to OSA,33,34 there is evidence that OSA in the context of obesity may amplify the risk for insulin resistance and dyslipidemia.30,34 Gozal et al. demonstrated an improvement in insulin resistance with AT in the context of obesity, and not in their non-obese subjects, which supports additive interactions between OSA and obesity.34 Figure 17-1 describes a mechanistic model of the contribution of OSA and obesity to the MetS. Unfortunately, the potential advantages conferred by treating OSA might be limited by parallel evidence suggesting that the treatment of OSA is associated with weight gain even in overweight populations.92
Obesity
The significance of obesity in modern-day society cannot be overstated, with recent evidence demonstrating 31.7% of US children between the ages of 2 and 19 were at or above the 85th percentile for weight between 2007 and 2008.93 The consequences of obesity are broad and far-reaching, with implications on physical health and psychosocial well-being. As described earlier, there are associations with hypertension,81 insulin resistance and glucose dysregulation,82–84 inflammatory markers,85–87 elevated triglycerides and low HDL,83 and vascular dysfunction.88 There is also evidence of body image dissatisfaction, reduced quality of life and lower self-esteem.94 Recent interest has also been placed in the relationship between sleep fragmentation and deprivation, including a wealth of epidemiologic data supporting an association between short sleep and being overweight or obese.95 Experimental studies demonstrated an association between sleep deprivation and decreased leptin96,97 and elevated ghrelin;98 these findings correlate with increased appetite and food intake.99–101 Buxton and colleagues presented findings in adult subjects showing a reduction in resting metabolic rate with an extended sleep restriction coupled with a circadian disruption.102 The odds of have an elevated BMI in a short sleeper have also been demonstrated to increase in the context of genetic heritability of elevated BMI, suggesting less environmental control of weight with a plausible increase in obesity potential with short sleep.103 Behavioral obesogenic correlates of insufficient sleep include maladaptive habits such as increased snacking104 and general food intake.105 Among sleep disorders, obstructive sleep apnea has naturally been front and center, with evidence supporting a phenotypic subtype with obesity. Other disorders with significant relationships with obesity include narcolepsy and nocturnal eating disorders.
Hart et al.95 recently completed a systematic review of 30 studies from 16 countries examining the epidemiologic evidence of association between sleep duration and obesity. There was significant agreement across the studies, demonstrating the presence of an inverse relationship between sleep duration and obesity risk. There were also six prospective studies that helped clarify temporal characteristics of short sleepers versus longer sleepers. In a particular study involving 2281 children between the ages of 3 and 12 years, BMI at follow-up was lower by 0.75 kg/m2 for each hour of additional sleep during baseline recording.106 In a separate study involving 1138 children between the ages of 2.5 and 6 years, those who were persistent short sleepers (<10 hours a night throughout follow-up) were 4.2 times more likely to become overweight or obese (OR = 4.2; 95% CI 1.6–11.1, p = 0.003).107
Among sleep disorders, obstructive sleep apnea is most affected by significantly elevated BMI. Epidemiologic characteristics in the general population suggest that 2% of school-aged children,108 and 13% of preschool children are afflicted by the condition.109 In contrast, 46% to 59% of obese children have obstructive sleep apnea.110–112 Contributing anatomic factors include adenotonsillar hypertrophy113 and fat deposition in the lateral fat pads,114 uvula115 and tongue.116 There is also increased collapsibility of the upper airway with obesity in terms of the pharyngeal critical pressure of collapse (Pcrit).117 Pcrit improves significantly with weight loss.118 There is also increased work of breathing and lower oxygen reserves with truncal obesity.119 With the reverse relationship, observational data suggest that obstructive sleep apnea may predispose individuals to gain weight by derangement in adipocyte hormone functioning. Levels of the satiety hormone leptin are elevated in adults with obesity and OSA – this has been postulated to be secondary to leptin resistance.120–122 This relationship has also been demonstrated in children, with further elevations in leptin with increases in severity of hypoxemia independent of BMI Z scores.123 In support of a causal relationship between OSA and elevations of leptin, a fall in leptin has been observed in children with MetS and OSA with initiation of CPAP therapy.31 Unfortunately, treatment of OSA is complicated and less successful in this population. In a recent meta-analysis by Friedman et al., 59.8% of children undergoing AT were cured of the condition when cure was defined as a residual AHI < 1 event per hour.78 This is compared to a 12% cure rate (AHI < 1) demonstrated in a meta-analysis of AT in obesity-related OSA.124 Therefore, the American Academy of Sleep Medicine supports the implementation of a repeat polysomnographic study after AT in the context of obesity.125 CPAP is the commonly acceptable secondary treatment modality for residual OSA and has been demonstrated to be effective in pediatric OSA.126–129 The use of nasal steroids and leukotriene inhibitors is also associated with significant improvements in reducing SDB events.130,131 Their combination also increases the likelihood of resolution of residual OSA after AT.132 However, evidence of their benefit in the context of obesity needs further clarification. Rapid maxillary expansion (RME) is another therapy that may be effective in the treatment of OSA133,134 but it will need further studies to examine its effectiveness in the context of obesity. Guilleminault et al. published a pilot study with a randomized cross-over design utilizing RME and AT that showed great promise for the use of RME with AT for residual OSA.135 Naturally, weight loss should be addressed in weight-related OSA to impact SDB, secondary metabolic consequences, and cardiovascular risk – but the current body of evidence does not yet elucidate the effectiveness of this strategy in treating OSA in the pediatric population. A case series of six young adolescents with morbid obesity demonstrated reductions in their apnea index from 14.1 events per hour to 1.6 events per hour with an average weight reduction of 18.7 kg.136 Bariatric surgery in morbidly obese children has cautiously been investigated with the inherent concern about possible adverse effects on physiologic growth and bone maturity. However, evidence from Kalra et al. showed resolution of OSA in a population of 10 morbidly obese adolescents (mean BMI = 60.8 ± 11.07) who underwent laparoscopic Roux en Y gastric bypass that resulted in a mean weight loss of 58 kg (mean AHI at baseline versus follow-up, 9.1 versus 0.65).110
Narcolepsy has also been associated with obesity, particularly with rapid onset of weight gain in a number of case series.137–139A Indeed, rapid weight gain and ultimately obesity appear to be a relatively consistent feature of many children with narcolepsy, and have been described as far back as in the 1930s.140 The tendency for obesity has been further demonstrated in mouse models of the disease in which hypocretin (orexin) neurons were genetically ablated. The association with obesity occurred despite decreased food consumption in comparison to non-ablated mice.141 The exact mechanism has yet to be ascertained, with evidence leading towards a complex set of relationships that entail the involvement of neurotransmitters in diurnal activity, feeding and metabolism. In rats, the neuropeptide dynorphin is co-localized with hypocretin neurons and the loss of these specific neurons is associated with hypophagia and obesity in comparison to rodents with only a loss of the hypocretin peptide.142 In regards to sleep distribution, total sleep has been observed to be similar between narcoleptic and non-narcoleptic human subjects, with a tendency for more disrupted nocturnal sleep in narcoleptics, as well as increased daytime sleep and an earlier peak in propensity for daytime sleep as compared to habitual nappers.143
Nocturnal eating disorder (NED) and sleep-related eating disorder (SRED) are other conditions that have been described in the context of obesity. NED involves excessive eating during the habitual sleep period while SRED entails recurrent episodes of nocturnal awakenings with associated eating, with or without recollection. NED is the more common of these two conditions and is self-reported in 15.7% of adults enrolled in a weight-loss program,144 and in up to 27% of subjects having completed bariatric surgery.145 These elevated figures are clearly different from the 1.5% prevalence in the general population that was recorded through a survey sample.145 SRED is a less prevalent condition in the general population, but has a high propensity in individuals with eating disorders. In a study by Winkelman et al. examining a population of psychiatric and non-psychiatric subjects, 16.7% of in-patients and 8.7% of out-patients with eating disorders were noted to have the condition in comparison to 4.6% of a student population and 1% of obese controls.146 The condition can be quite severe in those afflicted, with up to half of subjects described in one study as having daily eating,147 and another citing a mean duration period of affliction spanning 15.8 years.148
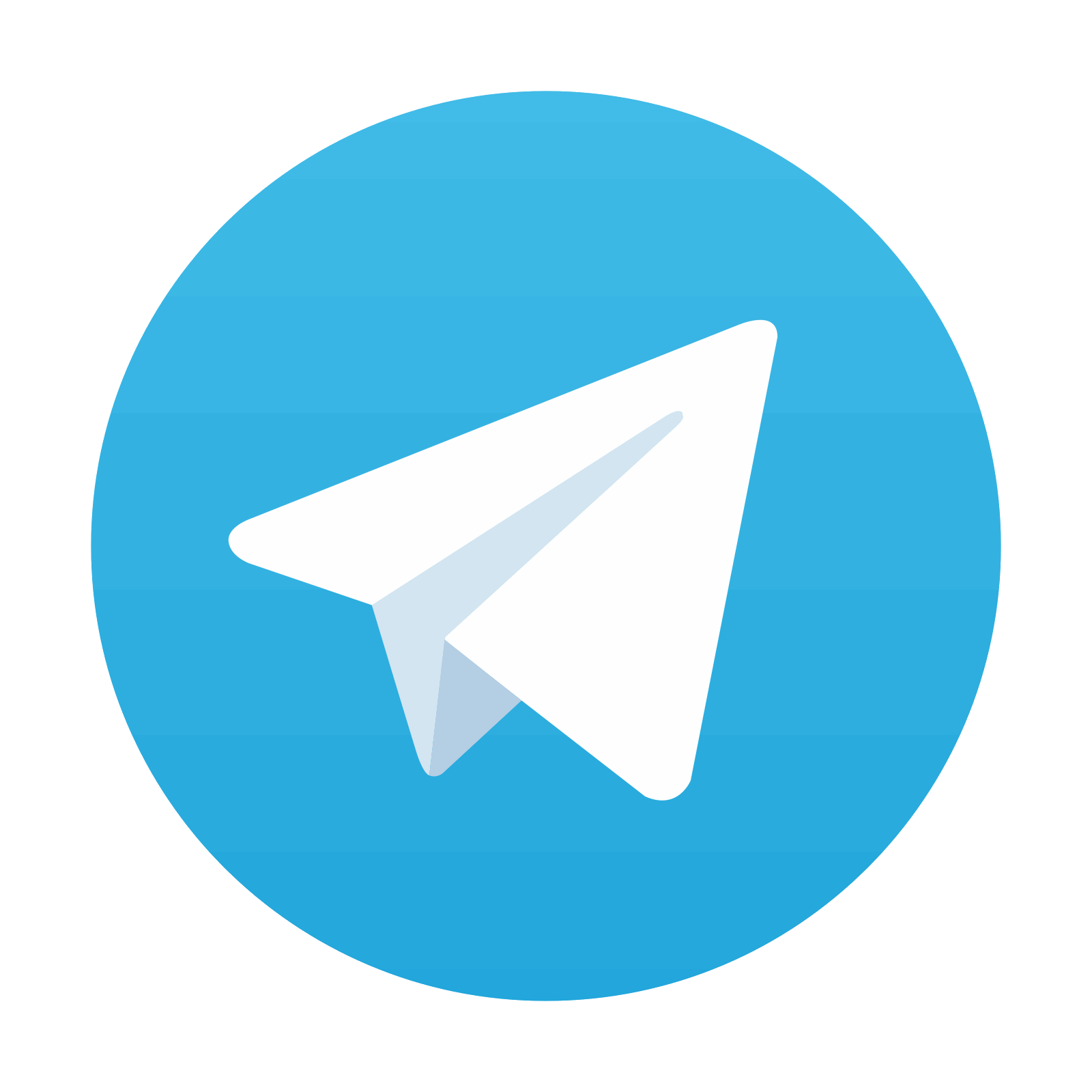
Stay updated, free articles. Join our Telegram channel

Full access? Get Clinical Tree
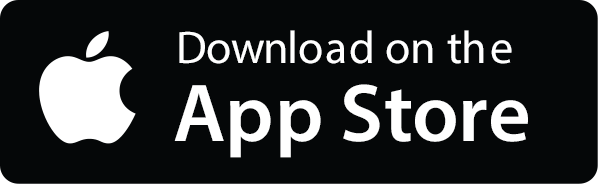
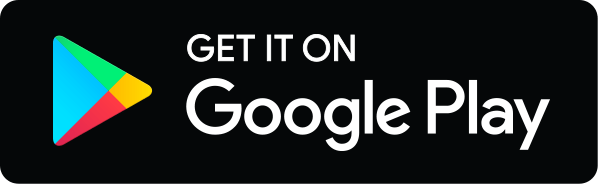