Fig. 55.1
The ability to sustain spontaneous ventilation can be viewed as a balance between neurological mechanisms controlling ventilation together with ventilatory muscle power on one side and the respiratory load, determined by lung, thoracic and airway mechanics, on the other. Weakness of the respiratory muscles, as observed in patients with neuromuscular disease, causes a respiratory imbalance, responsible for alveolar hypoventilation. PaO 2 partial arterial oxygen pressure, PaCO 2 partial arterial carbon dioxide pressure
The weakness of the respiratory muscles is the main contributor of the imbalance of the respiratory balance in patients with NMD. In patients with Duchenne muscular dystrophy (DMD), the weakness predominates on the diaphragm and the expiratory muscles and progresses ineluctably with age (Nicot et al. 2006). The spinal muscular atrophies (SMAs) are inherited autosomal recessive disorders. Severity is inversely proportional to the amount of survival motoneuron protein in the anterior horn cell. SMAs range from essentially total paralysis and need for ventilatory support from birth to the relatively mild muscle weakness presenting in the young adult. Diaphragmatic strength is generally preserved, and respiratory muscle weakness predominates on the other inspiratory muscles and the expiratory muscles (Nicot et al. 2006). Respiratory failure is less frequent in other muscular dystrophies, such as Becker, limb-girdle and facioscapulohumeral dystrophies. Patients with congenital myopathies represent a heterogeneous group of patients, with a various involvement of the respiratory muscles (Nicot et al. 2006). However, the respiratory condition of these children may deteriorate functionally with growth because weakened muscles are unable to cope with increasing body mass and energy demand.
Patients with NMD have normal lungs, airways and chest wall. Respiratory load is thus expected to be within the normal range. However, measurements of the oesophageal (PTPoes) and diaphragmatic pressure time product (PTPdi), which are correlated to the oxygen consumption of the global inspiratory muscles for PTPoes, and of the diaphragm for PTPdi, have shown values 2–3 times higher than normal (Nicot et al. 2006). This apparently abnormal increase in respiratory load may be explained by the chronic limitation of the respiratory movements, leading to progressive “micro-atelectasis” and stiffening of the rib cage. Weakness of the paravertebral muscles may promote thoracic deformity, such as scoliosis, which may also increase respiratory load. However, central drive is normal, and even increased in case of significant respiratory muscle weakness, as reflected by the increase in the pressure swing occurring 100 ms after the onset of the inspiration (P0.1) (Fauroux et al. 2009; Mulreany et al. 2003). Also, the ventilatory response to carbon dioxide (CO2), which is a strong respiratory stimulant, is directly correlated to the strength and the endurance of the respiratory muscles in patients with NMD (Fauroux et al. 2009; Toussaint et al. 2007).
55.2.2 Consequences of the Weakness of the Respiratory Muscles
Ventilation has to adapt to various physiological and pathological situations. Physiological situations are represented by sleep and exercise. Sleep is associated with changes in respiratory mechanics, such as an increase in ventilation-perfusion mismatch, an increase in airflow resistance and a fall in functional residual capacity (Fig. 55.2). Although the activity of the diaphragm is preserved, that of the intercostal and the upper airway muscles is decreased significantly. The physiological relaxation of the upper airway muscles contributes to a certain degree of upper airway obstruction that may aggravate the fall in alveolar ventilation. Finally, central drive and chemoreceptor sensitivity are less efficient during sleep than during wakefulness. All these abnormalities explain a physiological degree of nocturnal hypoventilation in healthy subjects, causing a rise in partial arterial carbon dioxide pressure (PaCO2) of up 3 mmHg (0.4 kPa). This decrease in alveolar ventilation predominates during rapid eye movement (REM) sleep.
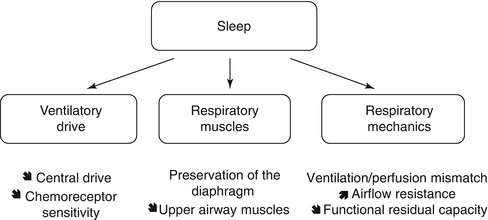
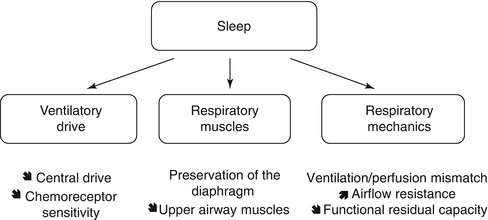
Fig. 55.2
Sleep is associated with a physiological degree of alveolar hypoventilation because of changes in respiratory mechanics, respiratory and upper airway muscles and respiratory drive
A correlation between respiratory muscle strength, represented by a global marker of respiratory muscle strength, i.e. vital capacity, and sleep-disordered breathing has been observed in patients with NMD (Ragette et al. 2002). Indeed, as the fall of inspiratory vital capacity progresses, hypoxia appears during REM sleep, total sleep time and daytime. The detection of nocturnal hypoventilation is thus mandatory after the occurrence of a certain degree of respiratory muscle weakness, which depends on the type of NMD. Markers associated with the occurrence of nocturnal hypercapnia have been identified for patients with DMD. Indeed, vital capacity and rapid shallow breathing have been shown to be sensitive predictors for daytime hypercapnia (Toussaint et al. 2007). However, predictors for nocturnal hypoventilation have not been clearly identified for other NMD.
Respiratory tract infection represents the most common situation that may precipitate respiratory failure in patients with NMD. Respiratory failure develops when tidal volume decreases, the patient being unable to generate a sufficiently negative intrathoracic pressure due to the weakness of his respiratory muscles. Metabolic demands are increased during a respiratory tract infection, and caloric intake is generally insufficient, leading to weight loss and malnutrition, which aggravate respiratory muscle weakness. Recurrent or prolonged chest infections are the signature of an insufficient respiratory muscle reserve and justify a respiratory muscle investigation and the implementation of cough-assisted techniques and eventually NPPV. Indeed, children with NMD having a history of recurrent chest infection had significantly lower inspiratory vital capacity, forced expiratory volume in 1 s (FEV1) and peak cough flow than those without a history of recurrent chest infections (Dohna-Schwake et al. 2006).
55.3 Benefits of NPPV in Patients with NMD
The role of NPPV is to assist or replace the patient’s respiratory muscles in order to normalise alveolar ventilation. Nocturnal NPPV is associated with a correction of gas exchange during sleep (Annane et al. 2007). Most interestingly, regular intermittent use of NPPV may correct gas exchange during spontaneous breathing (Mellies et al. 2003). This residual effect of NPPV may be explained by the resetting of ventilatory drive in case of chronic hypercapnia, the rest of the respiratory muscles and the increase of chest wall and lung compliance due to NPPV (Nickol et al. 2005). However, NPPV has not been shown to improve symptoms related to sleep-disordered breathing (Annane et al. 2007). Neither has any study shown that NPPV is associated with an improvement or a stabilisation of lung or respiratory muscle function (Annane et al. 2007).
By assisting the respiratory muscles, NPPV is expected to prolong survival. This major beneficial effect has been observed for patients with DMD and SMA type I. Indeed, data from the national Danish DMD registry over a period of 30 years observed an association between the decrease in mortality rate and the increase in NPPV use (Jeppesen et al. 2003). Also, survival of children with SMA type I increased over the period 1995–2006 as compared to the period 1980–1994, due to the combined use of mechanical ventilation, cough-assisted techniques and nutritional support by means of a gastrostomy (Oskoui et al. 2007).
Quality of life is a major issue for patients with chronic diseases who are proposed lifelong technological support such as NPPV. Several studies in children and adults have shown that the implementation of NPPV is not associated with a decrease in quality of life, except for the physical components, which reflect the progression of the peripheral muscle weakness (Kohler et al. 2005; Raphael et al. 2002; Young et al. 2007). Indeed, all social and emotional components remained stable after the start of NPPV. Most importantly, patients usually score their quality of life better than do their parents, grandparents or health care givers (Bach et al. 2003; Young et al. 2007).
Sufficient respiratory movements are necessary for normal lung and chest wall growth and even lung differentiation in the antenatal period. Abnormal development of the diaphragm and the intercostal muscles leads to lung hypoplasia in mice (Inanlou and Kablar 2003, 2005). In clinical practice, hypoplasia and deformation of the chest, and consequently of the lung, may be observed in infants and young children in whom the consequences of respiratory muscle weakness are the most pronounced (Bach and Bianchi 2003). The ability of NPPV to preserve normal lung and chest wall growth is thus an important question, which remains unresolved.
55.4 When to Start NPPV in Patients with NMD
All experts agree on the necessity to start NPPV in patients with NMD who have daytime hypercapnia or a history of a severe respiratory exacerbation (A Consensus Conference Report 1999; ATS Consensus Conference 2004; Robert et al. 1993). Indeed, these two situations are the signature of overt respiratory failure, the patient being unable to maintain sufficient minimal alveolar ventilation while awake in the first situation and having insufficient respiratory muscle reserve in the second case. As stated before, the regular use of nocturnal NPPV is associated with a normalisation of nocturnal and daytime gas exchange (Annane et al. 2007). However, the objective benefit of NPPV to reduce the hospitalisation rate is less clear (Annane et al. 2007).
In patients with NMD, alveolar hypoventilation develops insidiously, over periods of months or years. The two classical criteria are preceded by a variable period of nocturnal hypoventilation during which treatable symptoms, such as frequent arousals, severe orthopnea, daytime fatigue and alterations in cognitive function, may deteriorate the daily life of the patient. This situation represents clearly a risk situation as observed in a recent study in which only one out of ten patients with isolated nocturnal hypercapnia did not require NPPV for daytime hypercapnia or respiratory failure over a 24-month period (Ward et al. 2005). The effect of NPPV on sleep quality and especially arousals and sleep fragmentation has been poorly studied. Sleep-disordered breathing is associated with neurocognitive dysfunction in children, and it is possible that the use of NPPV may be associated with an improvement in memory, attention and mood in children with NMD and nocturnal hypoventilation. A major challenge is thus to determine the optimal timing for a sleep study in a paucisymptomatic patient (Fauroux and Lofaso 2005). A polysomnography should be realised without delay when the patient recognises symptoms related to sleep-disordered breathing, but patients with NMD tend to underestimate symptoms such as fatigue before using mechanical ventilation. Sleep-disordered breathing is difficult to establish in children because of reliance on parents and second-hand caregivers who have a different perception of the child’s disease. Future studies should thus try to determine the most pertinent criteria to schedule a sleep study, according to the type of NMD. But then, the difficulty remains concerning the definition of nocturnal hypoventilation. Most often, this definition is based on a cut-off PCO2 value, which is transgressed during a defined percentage of sleep time or of study period. But other respiratory events during sleep, as those recently recommended for the diagnosis of sleep-related breathing disorders, such as sleep fragmentation, may be important to be taken in account. Moreover, it may be possible that the optimal definition of nocturnal hypoventilation differs according to the underlying disease and also in children compared to adults. A definition exclusively based on the PCO2 value may be too restrictive and insufficiently relevant from a clinical perspective.
The weaning from invasive ventilation for a severe acute respiratory exacerbation by means of NPPV is also an important issue. A child with NMD on invasive ventilation may be extubated and switched to NPPV when the following criteria are fulfilled: an inspired fraction of oxygen (FiO2) close to room air, minimal respiratory secretions and an acceptable inspiratory and expiratory pressures on the ventilator (<20 and 6 cm H2O for the inspiratory and expiratory pressures, respectively). NPPV with cough-assisted techniques should be used intensively after extubation with similar pressure levels and backup rate. The weaning of NPPV should associate increasing periods of spontaneous breathing, separated by periods of NPPV without decreasing the pressure levels and backup rate. The occurrence of a respiratory exacerbation requiring invasive ventilation is the signature of insufficient ventilatory reserve and severe respiratory muscle weakness, justifying the long-term maintenance of NPPV in most cases.
Table 55.1
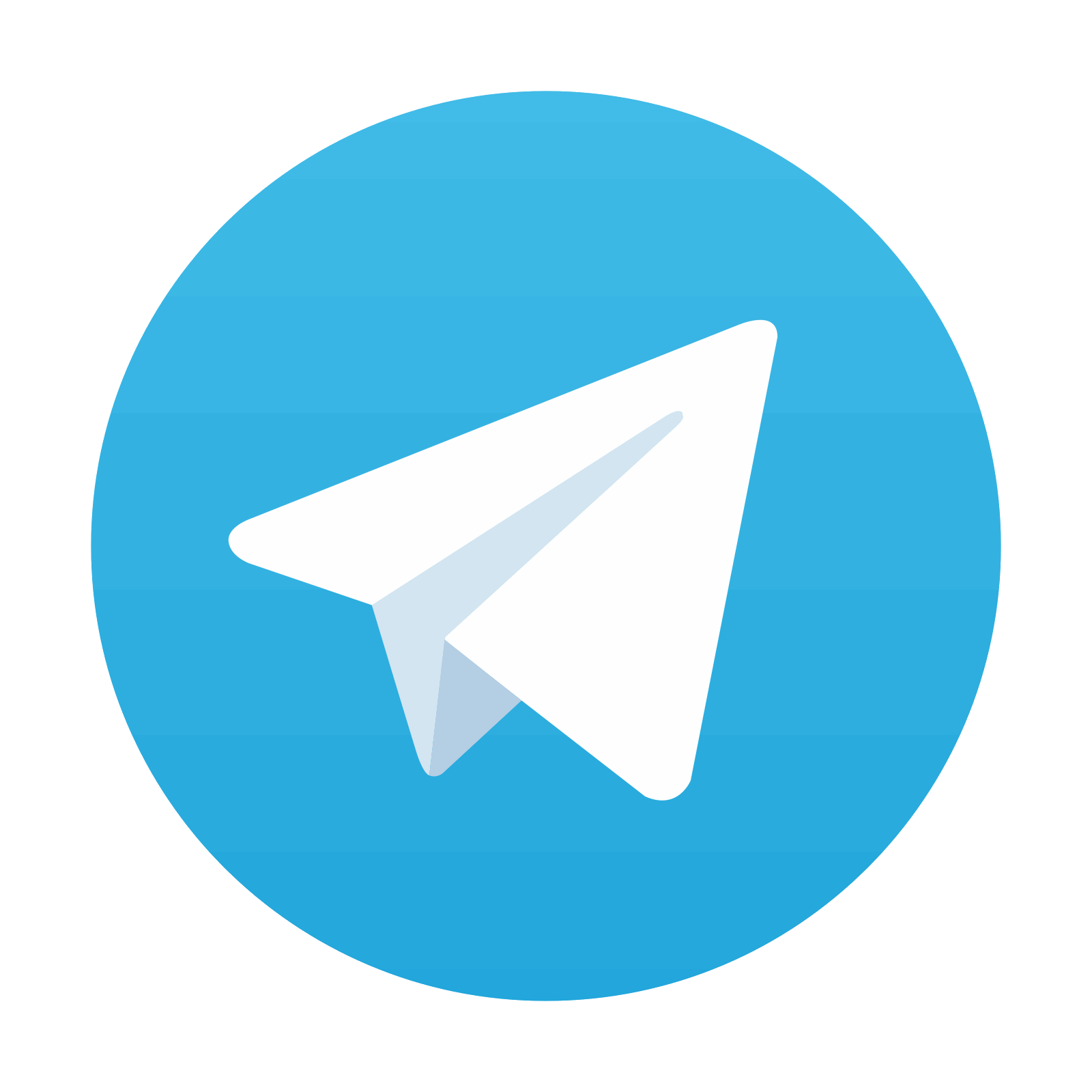
Contraindications for NPPV
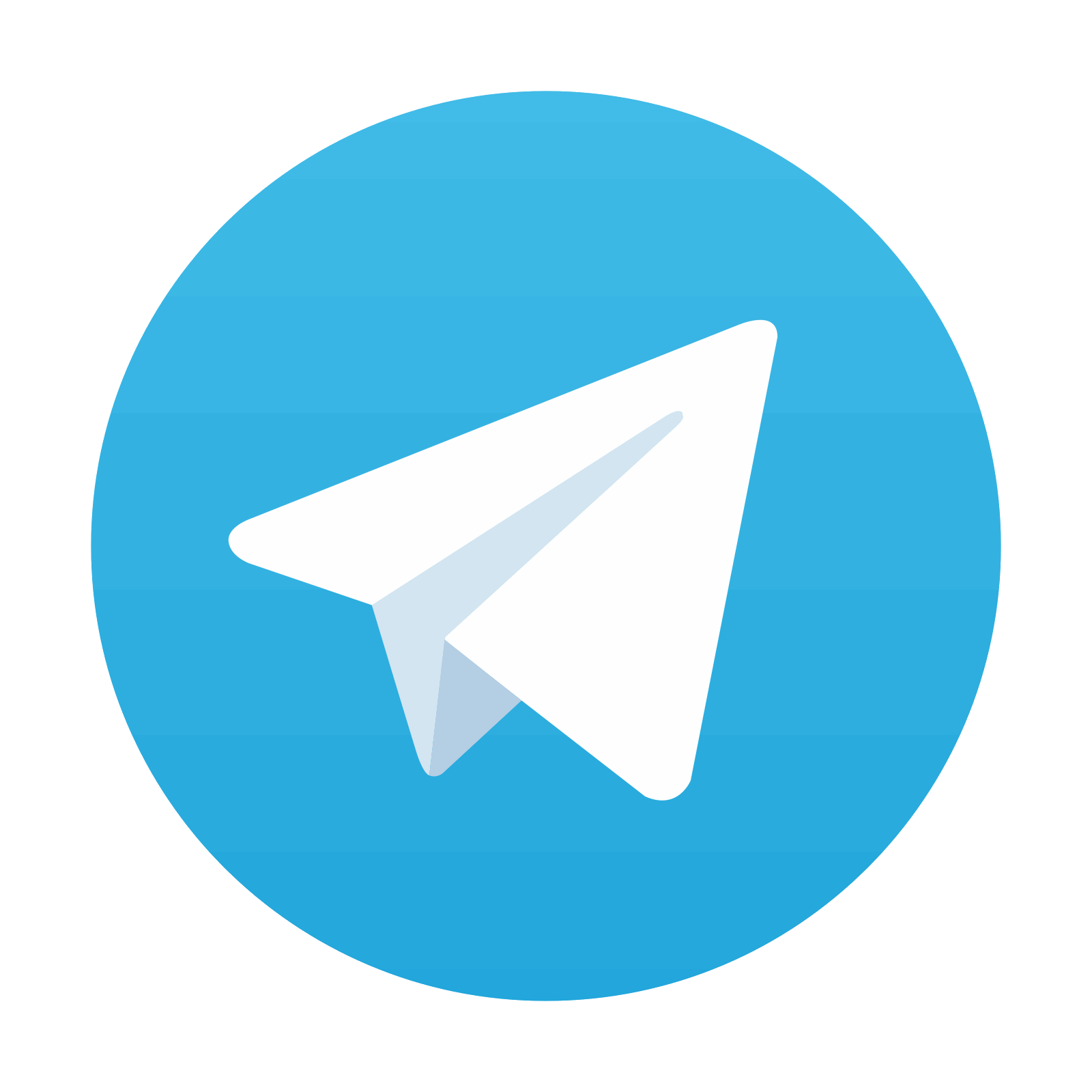
Stay updated, free articles. Join our Telegram channel

Full access? Get Clinical Tree
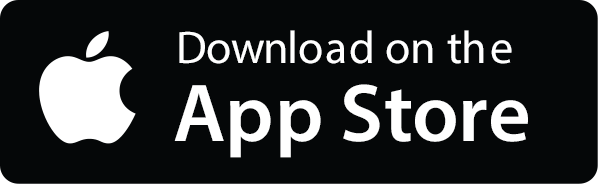
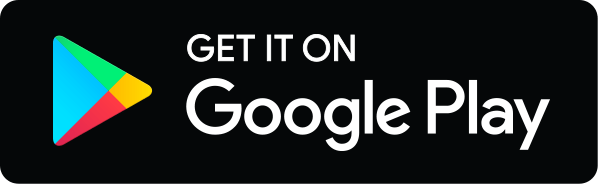
