Mechanical Ventilation During Pregnancy
Nan H. Troiano
Thomas M. Jenkins
Mechanical ventilatory support is one of the major supportive modalities used in critical care. An essential element of cardiopulmonary resuscitation, it can be life-saving during a variety of acute and chronic disorders, when respiratory drive is depressed, or when the patient lacks the neuromuscular ability to breathe. Further, the lung is usually one of the essential organs involved in multiple organ-system dysfunction or failure. Thus, delivery of appropriate ventilatory support can be challenging. For a number of reasons, challenges are compounded when the need for ventilatory support occurs in a patient who is pregnant.
This chapter reviews indications for intubation and mechanical ventilatory support during pregnancy. Types and modes of both conventional and newer ventilatory adjuncts are presented. Issues related to use during pregnancy as well as specific care measures are also described. More thorough description of disease processes that may lead to respiratory or ventilatory failure during pregnancy may be found elsewhere in this text.
Indications
Patients with refractory hypoxemia or tissue hypoxia who are unable to ventilate sufficiently despite supplemental therapy will require intubation and mechanical ventilation. Mechanical assistance may be necessary because ventilation is inadequate to regulate pH, because adequate oxygenation cannot be achieved at the fraction of inspired oxygen (FiO2) available without manipulating the mode of ventilation, or because activity of the ventilatory muscles places excessive demands for blood flow on an already compromised cardiovascular system. General guidelines for the diagnosis of respiratory failure are presented in Table 5-1.1 It should be noted that determination of some of these criteria is based on results of pulmonary function tests, which quantify respiratory function by measuring lung volume during normal and maximal ventilation.
Conventional Types of Mechanical Ventilators
Generally, conventional ventilators can be divided into two types: volume-cycled and pressure-cycled. They are classified according to the mechanism that terminates the inspiratory phase. Both types are positive pressure systems. The specific ventilator selected for a given clinical situation may depend on a number of variables, including the models available in a specific institution and the familiarity or experience level of the patient’s care providers with one type over another. Irrespective of which type or model is used, a mechanical device used to sustain life is only as good as its design and the health care team using it.
Negative Pressure
Early ventilators were negative pressure systems known as iron lungs. The patient’s body was encased in an iron cylinder and negative pressure was generated, which enlarged the thoracic cavity. A modification of this system involves a suit or shell that is fitted over the patient’s chest with a hose connecting the device to a negative pressure generator. In either model, lung inflation is accomplished by reducing the pressure in the container to below atmospheric pressure. This causes the pressure surrounding the chest to drop below the pressure in the lungs, and the chest expands. The lungs then expand and the pressure inside them becomes less than atmospheric. Atmospheric gases are drawn into the lungs until lung pressure and atmospheric pressure reach equilibrium. Inspiration then ends. Once subatmospheric pressure surrounding the chest is released, the natural elastic recoil of the thoracic cage and lungs causes lung pressure
to exceed atmospheric pressure. Thus, gas leaves the lungs until pressures are again equal.
to exceed atmospheric pressure. Thus, gas leaves the lungs until pressures are again equal.
Table 5.1 Acute Respiratory Failure: Indications for Endotracheal Intubation and Mechanical Ventilatory Assistance | ||||||||||||||||||||||||||||||||||||
---|---|---|---|---|---|---|---|---|---|---|---|---|---|---|---|---|---|---|---|---|---|---|---|---|---|---|---|---|---|---|---|---|---|---|---|---|
|
Negative pressure ventilator systems are advantageous in that they mimic normal respiration. However, such systems are rarely used except in select out-of-hospital settings.
Positive Pressure
Volume-Cycled
Volume-cycled ventilators are the most frequently used type in most adult critical care settings. The basic principle behind this type of ventilator is that once a designated volume of air is delivered to the patient, inspiration is terminated.
At the moment of cycling, the time taken to deliver the volume, the pressure in the patient circuit (due to the resistance to air flow), and the flow rate may all vary from one respiratory cycle to the next. The only parameter that remains constant is the volume.
Traditionally, volume-cycling is accomplished with the use of a double-circuit ventilator.2 In these systems, the primary circuit is usually a blower and the secondary circuit comprises a bellows assembly. The volume-cycling mechanism itself can be either pneumatically or electronically operated. Advances in ventilator technology have produced reliable timing and flow-sensing devices that are electronically integrated to measure air volumes. These devices are now found in single-circuit, volume-cycled systems.
Use of a volume-cycled ventilator requires the clinician to set the volume, flow, and frequency of air delivered. The primary advantage to this type of system is that, despite changes in patient lung compliance, a consistent tidal volume (Vt) is delivered.
Pressure-Cycled
The pressure-cycled ventilator works on the basic principle that once a preset pressure is reached, inspiration is terminated. At this pressure point, the inspiratory valve closes and exhalation occurs passively. At the moment of cycling, the volume of air delivered, the time taken to deliver the volume, and the flow rate may all vary from one respiratory cycle to the next. The only parameter that remains constant is the preset cycling pressure. Pressure-cycling mechanisms in the ventilator may be pneumatic, electronic, or a combination of both.
Clinical judgment dictates the cycling pressure selected. Because the patient’s lung condition is not known when ventilatory assistance begins, there is no simple way of predicting the initial pressure requirements. Once the preliminary settings are made, the ventilator is connected to the patient. The volume delivered at that pressure and flow rate is determined with a volume-measuring device. Final adjustments to the cycling pressure and flow rate are then performed based on patient assessment data. If the Vt is too low, the cycling pressure is increased. If the Vt is too high, the cycling pressure is reduced. Clinically, as a patient’s lungs become less compliant, the volume of air delivered to the patient may decrease. Thus, to ensure adequate minute ventilation and to detect changes in lung compliance and resistance, inspiratory pressure, rate, and exhaled tidal volume must be monitored frequently.
Although volume-cycled ventilators remain the most frequently used type in most adult situations, pressure-controlled and time-cycled systems are increasingly chosen in select cases. Because high volumes and pressures can lead to lung damage, pressure-controlled ventilation allows the clinician to select and control mean airway pressure as well as peak airway pressure. This may be especially beneficial in patients refractory to conventional ventilation or those with acute respiratory distress syndrome.
Time-Cycled
The time-cycled ventilator works under the basic principle that once a preset time is completed, inspiration is terminated. Expiratory time is determined by inspiratory time and the rate of breaths per minute. This is expressed as inspiratory to expiratory ratio (I:E ratio). The typical I:E ratio is 1:2. This means that for every second of inspiration the ventilator delivers, it allows twice that time for expiration.
Time-cycled machines limit the pressure achieved in the system and cause the ventilator to cycle at a preset interval, thereby setting frequency. Tidal volume (Vt) is varied by adjusting the flow rate and inspiratory time, or by setting minute ventilation and frequency. Many ventilators, especially those used in neonatology, are pressure-limited but time-cycled, allowing the delivered Vt to be highly variable.
Modes of Mechanical Ventilation
The mode of ventilation generally refers to the interaction between the patient’s ventilatory effort and mechanical assistance. There are a variety of modes from which to choose, once the type of ventilator has been selected. Conventional modes include control, assist-control (AC), intermittent mandatory ventilation (IMV), synchronized intermittent mandatory ventilation (SIMV), and continuous positive airway pressure (CPAP). A schematic representing the ventilatory pattern for each is presented in Figure 5-1.2
Control
With controlled mandatory ventilation (CMV), the ventilator delivers a preset Vt at a preset number of times per minute. The patient cannot spontaneously ventilate or “trigger” breaths; thus, the machine controls ventilation. This mode is restricted to patients who are apneic as a result of brain injury, sedation, or muscle paralysis. If the patient is conscious or is not paralyzed, this mode can provoke high anxiety and discomfort. Properly functioning ventilator alarms are crucial to the safe use of CMV.
Assist-Control
In the assist-control (AC) mode, the ventilator delivers a breath either when triggered by the patient’s inspiratory effort or independently, if such an effort does not occur within a preset period of time. All breaths are delivered under positive pressure by the machine, but unlike CMV, the preset rate can be exceeded by the patient’s triggering of additional breaths. This triggering is accomplished by setting a sensitivity detector that identifies a patient breath by the negative pressure the patient uses to initiate inspiration. Particular attention must be paid to proper adjustment of the level of sensitivity of the triggering mechanism. If the ventilator is too sensitive, it may autocycle, whereby it delivers a breath when the patient did not try to initiate inspiration. If it is not sensitive enough, it may require the patient to generate large negative pressure in order to receive additional breaths.
The primary complication associated with this mode of ventilation is respiratory alkalemia. Because every breath, whether triggered by the patient or independently delivered by the ventilator, is at the preset Vt, hyperventilation is possible. This effect may be exacerbated in the obstetric patient because of the compensated respiratory alkalemia that normally accompanies pregnancy. It should be recalled that alkalemia results in a left shift of the oxyhemoglobin dissociation curve, thus making it more difficult for oxygen to be released from hemoglobin to tissues. Concepts related to oxygen transport physiology are presented in Chapter 4.
Intermittent Mandatory Ventilation
Intermittent mandatory ventilation (IMV) allows interspersion of spontaneous breaths by the patient with machine breaths. Spontaneous breaths are at the patient’s own Vt, and machine breaths are delivered at the preset Vt. IMV was first introduced in 1971 for use with neonates with respiratory distress syndrome (RDS), because conventional ventilators were unable to deliver the rapid breath rates associated with this condition. Shortly thereafter, it was proposed by Downs and colleagues as an alternative method for weaning adults from mechanical ventilation.3
A schematic representation of an IMV circuit is depicted in Figure 5-2.4 The patient is connected to a common source of oxygen through two parallel circuits. The first contains a volume-cycled ventilator. The other contains a reservoir bag filled with the inhaled gas mixture. A unidirectional valve in the circuit allows the patient to breathe spontaneously from the reservoir bag when a ventilator breath is not being delivered. The pattern of ventilation is such that a machine breath may be delivered at the same time the patient is spontaneously breathing. This concept, referred to as “stacking of breaths,” is uncomfortable for the patient and increases volume and pressure in the airway. Current IMV systems eliminate this problem by synchronizing patient and machine breaths.
Synchronized Intermittent Mandatory Ventilation
Synchronized intermittent mandatory ventilation (SIMV) allows spontaneous breathing between mechanically delivered ventilator breaths. In addition, when the ventilator delivers a breath, it will wait until the patient starts inhalation and synchronize the delivered breath. This technique was introduced because of concern that a mechanical breath might be superimposed on a spontaneous breath. It prevents stacking of breaths and concomitant increases in peak inspiratory pressure (PIP), mean airway pressure, and mean intrapleural pressure.
It remains one of the most common modes used in obstetric critical care situations.
It remains one of the most common modes used in obstetric critical care situations.
Continuous Positive Airway Pressure
In continuous positive airway pressure (CPAP), pressure at the airway opening is maintained above atmospheric level throughout the respiratory cycle during spontaneous breathing. CPAP may be delivered through a special mask to patients who do not have an endotracheal or tracheostomy tube. However, the mask must fit exactly so as to prevent air leaks. This mode of ventilation was first used as positive-pressure oxygen breathing to help keep the lungs expanded in patients with crushing chest injuries and to treat infants with RDS.
Gas exchange across the alveolar capillary membrane is promoted as functional residual capacity (FRC) is increased. However, the patient maintains a high level of work in breathing with this mode because she is responsible for all ventilatory effort. The CPAP mode is used more often during weaning from mechanical ventilatory assistance.
Adjuncts to Mechanical Ventilation
Pressure Support Ventilation
Pressure support ventilation (PSV) augments a patient’s spontaneous breaths during mechanical ventilation. At the onset of every spontaneous breath, the negative pressure generated by the patient opens a valve that delivers the inspired gas at the desired pressure, usually 5 to 10 cm H2O. This is designed to increase the Vt and reduce the work of breathing. In essence, the pressure boost is delivered to overcome resistance in the endotracheal tube and ventilator circuit tubing.
Newer microprocessor-driven mechanical ventilators include pressure-support modes that operate in conjunction with their demand-flow valve systems. The principal advantages of PSV are decreased work of breathing for the patient and improved subjective reports of comfort.
Positive End-Expiratory Pressure
Mechanical ventilation with positive end-expiratory pressure (PEEP) is designed for any pulmonary condition with widespread alveolar collapse. A pressure-limiting valve in the expiratory tubing prevents pressure in the airways from returning to atmospheric pressure at the end of expiration. This positive pressure in the alveoli at end-expiration helps prevent alveolar collapse and promotes gas exchange across the alveolar–capillary interface. This ventilatory adjunct increases the FRC, the lung volume at end-expiration. In select patients, this increases alveolar participation in gas exchange and allows the fractional concentration of inspired oxygen (FiO2) to be lowered.
Newer Methods of Mechanical Ventilation and Oxygenation
The primary purposes of mechanical ventilation are to achieve adequate alveolar ventilation and to improve oxygen exchange. Traditionally, volume-limited ventilation used alone or with spontaneous breathing has been the only form of mechanical assistance commonly used in adults. In addition, enrichment of FiO2 and the addition of positive end-expiratory pressure have been the principal means of improving oxygenation. Over time, newer techniques have been introduced to clinical practice. Because the majority of these newer modes are pressure-cycled, independent volume monitoring is highly desirable.
Pressure Control and Inverse I:E Ratio
It has been common practice in mechanically ventilated patients to allow at least as much time for exhalation as for inhalation. The rationale for this concept is to prevent the trapping of gas in the airways. However, gas exchange may be markedly improved when the I:E ratio is forced to values greater than 1:1. This principle is known as inverse ratio ventilation (IRV) or inverse I:E ratio ventilation. It has been applied clinically to neonates with hyaline membrane disease as well as adults with refractory acute respiratory distress syndrome and other forms of hypoxemia.
This method of ventilation may be accomplished in at least two ways. First, the rate of flow delivery during conventional volume ventilation may be decreased. More commonly, pressure-controlled ventilation is utilized. This involves maintenance of pressure at a controlled level for a fixed period of time.
The mechanism for the improvement of oxygenation with this method remains uncertain, although increased mean intrathoracic pressure and increased lung volume are most likely involved. Sustained tethering forces may recruit lung units that would otherwise remain collapsed, and units with very slow time constraints may be given sufficient time to ventilate.5
Advantages of IRV include improved oxygenation and reduced peak cycling pressures. A disadvantage of
IRV is a higher mean intrathoracic pressure, which may impede venous return and contribute to barotrauma. It should also be noted that patient cooperation is essential; thus, adequate sedation is crucial.
IRV is a higher mean intrathoracic pressure, which may impede venous return and contribute to barotrauma. It should also be noted that patient cooperation is essential; thus, adequate sedation is crucial.
High-Frequency Ventilation
High-frequency ventilation (HFV) is a collective term that refers to modes of ventilation in which tidal volumes smaller than anatomic deadspace are moved at frequencies that range from 60 to 3,000 cycles per minute. High-frequency positive pressure ventilation (HFPPV) is identical in concept to conventional ventilation, with the exception that tidal volumes are very small and cycling frequencies are very fast. High-frequency jet ventilation (HFJV) works in a somewhat different way. With this method, a small-diameter injecting catheter is positioned in the central airway. This catheter then pulses gas along the axis of the lumen under high pressure at a rapid cycling rate, usually between 60 and 240 cycles per minute. HFJV is the most common type of HFV used in adult settings.
Yet another type of HFV is high frequency oscillation (HFO). This method works on the principle that very small volumes of gas (1 to 3 mL/kg) are moved back and forth by a piston at extremely high frequencies (500 to 3,000 cycles per minute).
All forms of HFV are characterized by lower peak airway pressures than conventional ventilation. Peripheral airway pressure is generally higher than measured central airway pressure, and mean alveolar pressure may not differ greatly from those observed during conventional ventilation. With rare exception, HFV does not produce cardiovascular side effects associated with conventional ventilation. HFV is generally utilized in carefully selected clinical situations, and data regarding use during pregnancy have not been reported.
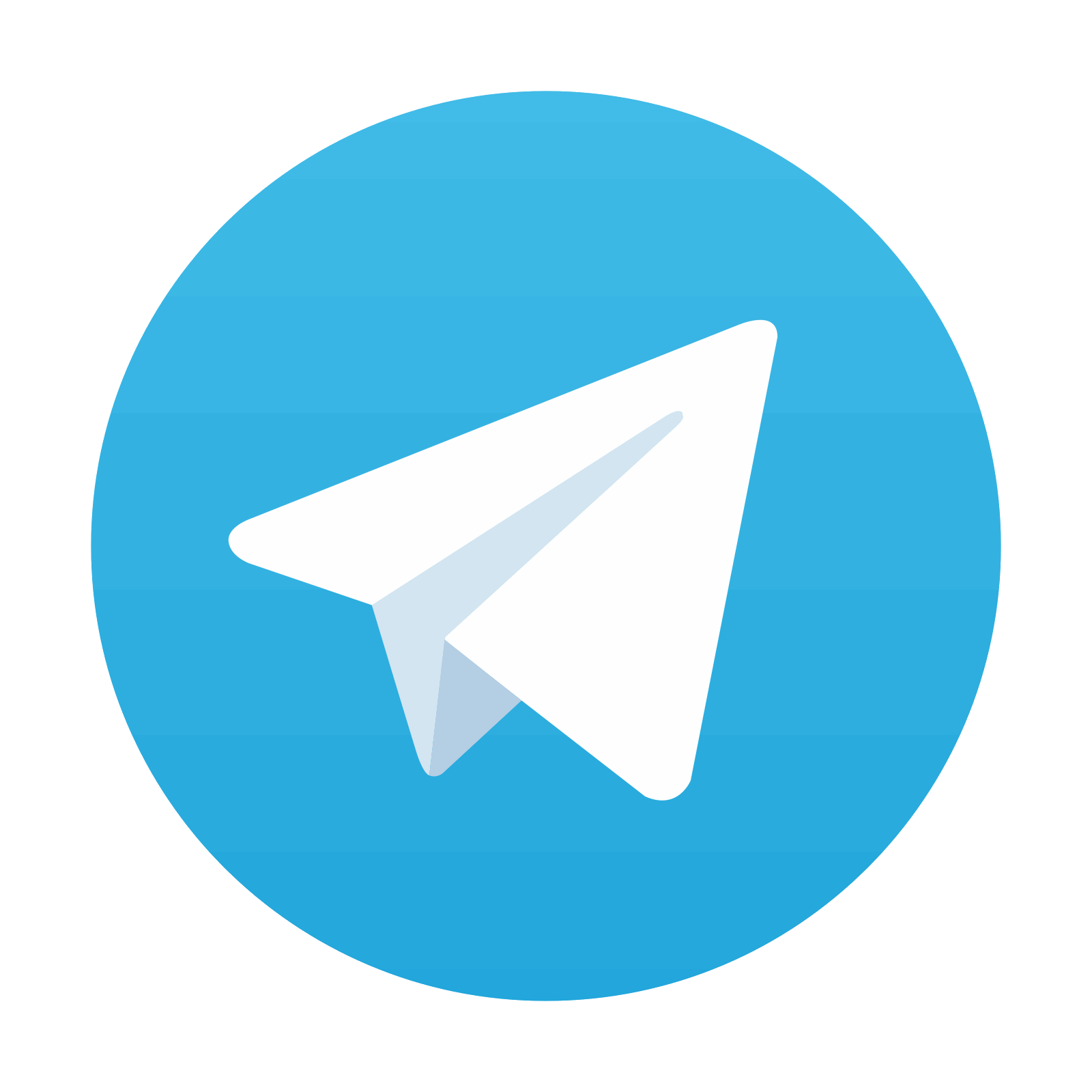
Stay updated, free articles. Join our Telegram channel

Full access? Get Clinical Tree
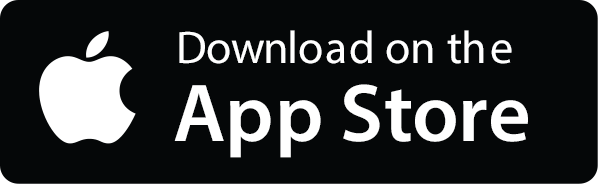
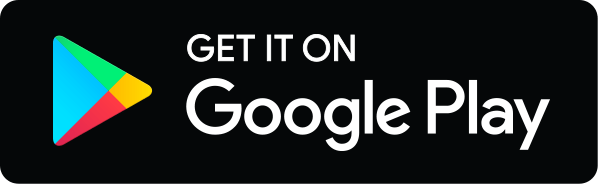