When noninvasive respiratory support is insufficient to achieve adequate gas exchange, insertion of an endotracheal tube and mechanical ventilator support may be necessary. Once it is determined that mechanical ventilation is needed, a variety of factors should be considered in choosing the mode of support. One factor is the type of mechanical ventilator to use: specifically either one of the “conventional approaches” or high-frequency ventilation. There are a number of conventional and high-frequency devices from which to choose; most are covered in greater detail in other chapters and will not be specifically addressed here. A second factor to be considered is the mode of ventilator support to be applied. Specifically, for conventional mechanical ventilation several different support modes may be selected on each device; again these are covered in more detail elsewhere in this book. A third factor to consider is the presumed benefits related to targeted gas exchange values and decreased work of breathing versus the relative risk of ventilator-induced lung injury (VILI). Perhaps most important, one needs to consider the underlying pathophysiology and its potential evolution over time. Finally, even from the time of initiating mechanical ventilator support, it is essential that the clinician has an active approach or plan for weaning and extubation (see also Chapter 24 ).
This chapter will discuss our approach to ventilator management in several of the most common neonatal respiratory disorders. It should be understood that there are a variety of devices and approaches other than those described here that one could employ resulting in safe, effective respiratory support of the ill neonate. Therefore, we will emphasize the key pathophysiologic features of each of the disorders and how the pathophysiology relates to the specific approach to mechanical ventilation. We firmly believe that the single most important factor associated with safe and successful ventilator management of the neonate is the person operating the device, not the device itself. A thorough understanding of the device, the mode applied, and the pathophysiology being managed, as well as a consistent, attentive approach to the specific infant being cared for, is essential to any success in managing respiratory problems in critically ill neonates.
Respiratory Distress Syndrome
It is indeed ironic that over 40 years after the introduction of continuous positive airway pressure (CPAP) as the first effective therapy for neonatal respiratory distress syndrome (RDS), and despite the marked technological advances that have been made during that time span, there has been a renewed emphasis on the application of noninvasive approaches, such as nasal CPAP, for respiratory support of neonatal lung disease. Despite the increasing success with noninvasive respiratory support, many neonates still require mechanical ventilator support for RDS, particularly those at the lowest gestational ages, and these are the ones that will be addressed here.
Key Pathophysiologic Features ( Table 23-1 )
Surfactant
A comprehensive overview of surfactant and its role in neonatal RDS is beyond the scope of this presentation. The reader is referred to Chapter 31 of this book and other publications for additional information. Quantitative, qualitative, and metabolic disturbances in surfactant play key roles in the pathophysiology of neonatal RDS. The net effect is decreased compliance of distal airspaces that can lead to atelectasis, ventilation:perfusion mismatch, and intrapulmonary shunt. Surfactant proteins play a critical role not only in the function of surfactant but also in the lung’s response to infection. The indications for and approach to surfactant replacement therapy for neonatal RDS continue to be an area of very active investigation and will not be addressed in this chapter .
Factor | Effect | Possible Intervention |
---|---|---|
Surfactant | Reduced quantity | Antenatal steroid therapy |
Impaired metabolism | Surfactant replacement | |
Reduced surfactant proteins | Surfactant-specific proteins | |
Disrupted function–proteins | Additional surfactant therapy | |
Lung liquid | Reduced clearance | Antenatal steroid therapy |
Sustained production | Postnatal steroid therapy | |
Mechanical | Reduced airspace compliance | Surfactant therapy |
High chest wall compliance | Positive end-expiratory pressure | |
Increased airway compliance | Low inspiratory tidal volumes | |
Development | Canalicular–saccular stage | Antenatal steroid therapy |
Thickened mesenchyme | Maternal stress | |
Immature capillary development | Effect of subclinical chorioamnionitis (?) | |
Inflammation | Altered surfactant metabolism | Antenatal steroid therapy |
Disrupted membrane integrity | Prevention/treatment of chorioamnionitis | |
Interrupted lung development | Postnatal steroids and other antiinflammatories |
Lung Liquid
Normal lung growth is regulated, in part, via fluid secreted into the potential airspace across the alveolar epithelial cells. Lung liquid secretion is generated via upregulated Cl − channels that actively transport Cl − into the lung lumen, with Na + and H 2 O following via an osmotic gradient. During fetal life the epithelial Na + channel that promotes Na + and fluid absorption from the airspace in postnatal life is downregulated. Delayed upregulation of all three subunits of the epithelial Na + channel has been found in preterm infants with RDS, persisting in some to at least 1 month of age. Additional factors that may contribute to persistent fetal lung liquid formation and delayed reabsorption of airspace fluid following preterm delivery include variable expression and activity of aquaporin channel proteins and persistent function of the secretory Cl − channels.
Developmental Lung Biology
Development of the mammalian lung is a complex, highly orchestrated process that is subject to interruption from numerous insults, particularly premature birth. The progressive stages of lung development are well described and include embryonic, pseudoglandular, canalicular, saccular, and alveolar stages. Vasculogenesis and angiogenesis, processes critical to lung growth and differentiation, are tightly connected throughout lung development. Postnatal viability first becomes possible for the human fetus during the latter phase of the canalicular stage, which occurs between 20 and 28 weeks’ gestation, or approximately 50% to 70% gestation. During this stage, rudimentary air sacs begin to form off the terminal airways, simple interstitial capillaries begin to organize around these potential airspaces, and type I and type II epithelial cells begin to differentiate, with type II cells beginning to produce surfactant. It must always be remembered that preterm birth, with subsequent exposure to increased ambient oxygen, unplanned gaseous inflation of the distal airspace, microbial colonization associated with prolonged tracheal intubation, and disturbances in nutrition, initiates a dramatic change in lung growth and development. The effects on lung growth and function, particularly at gestations of <30 weeks, may be lifelong, even for infants not diagnosed with bronchopulmonary dysplasia. It is in the context of this immature stage of lung development, and the potential for adverse effects, that the following discussion on ventilatory support for neonatal RDS should be considered.
Relevant Principles of Ventilation
In our neonatal intensive care unit (NICU) we most commonly use high-frequency oscillatory ventilation (HFOV) as the initial mode of support for those infants that require mechanical ventilation for neonatal RDS, at any gestational age. It is important to emphasize that there is no clear evidence that HFOV provides increased benefit (nor risk) compared to more conventional approaches to mechanical ventilation (i.e., volume targeted, surfactant treated) in terms of short-term outcomes such as initial gas exchange and subsequent diagnosis of bronchopulmonary dysplasia (BPD) prior to initial discharge. Our approach to conventional ventilation, which is primarily volume targeted in nature, will also be discussed. Regardless of the mode of ventilation employed, the primary objective in the management of neonatal RDS is to minimize the initial use and/or duration of exposure to any form of invasive mechanical ventilation through aggressive application of early noninvasive modes of respiratory support ( Tables 23-2 and 23-3 ), as well as the application of written guidelines to promote weaning and extubation from mechanical ventilation when applied ( Table 23-4 ). The key to management includes recognition of the predominant pulmonary pathophysiology, which for RDS is typically a diffuse “alveolar” disease, coupled with the potential to disrupt immature lung development through pathways leading to or associated with VILI. The management of the very preterm infant is additionally confounded by the underlying inflammatory milieu that is often present in association with clinical/subclinical chorioamnionitis and impaired intrauterine growth. The key to all lung-protective ventilation strategies in infants with diffuse alveolar disease (i.e., diffuse microatelectasis) is the recruitment and maintenance of optimal lung inflation and avoidance of excessive tissue stretch. In our NICU, we are more comfortable achieving these goals with HFOV, although similar strategies can be achieved with conventional ventilation.
Indication | Comment |
---|---|
Consider noninvasive respiratory support initially for: |
|
After 10 minutes of resuscitation if: |
|
After surfactant administration if: |
|
While on mechanical ventilation if: |
|
Other |
|
Indication | Comment |
---|---|
Infant of <26 weeks’ gestation | Consider for prophylactic surfactant therapy (NB: recent evidence no longer supports this) |
Absent/poor respiratory effort | Inadequate/sporadic effort, poor air entry |
Apnea/bradycardia | Refractory; recurrent; requiring PPV |
Hypoxemia | FiO 2 > 0.4-0.6 to maintain targeted PaO 2 /SpO 2 |
Hypercarbia | PaCO 2 > 60-65 mm Hg with pH < 7.20 |
Severe distress | Marked retractions on noninvasive support |
Suspected airway obstruction | Severe micrognathia, oropharyngeal mass, other |
Cardiovascular collapse | Heart rate <60 or shock; CPR |
Congenital malformations | Diaphragmatic hernia, choanal atresia, other |
WEIGHT (G) | ||||
---|---|---|---|---|
<1000 | 1000-2000 | 2000-3000 | >3000 | |
High-Frequency Ventilation | ||||
<SPAN role=presentation tabIndex=0 id=MathJax-Element-1-Frame class=MathJax style="POSITION: relative" data-mathml='P¯aw’>P⎯⎯⎯awP¯aw P ¯ aw | 8 | 9-10 | 10-12 | 12 |
Δ P /amp | 16 | 18 | 20 | 22 |
FiO 2 | <0.40 | |||
PC-SIMV and PSV | ||||
PIP | <16 | 16-20 | 20 | |
PEEP | <6 | <7 | <8 | |
PS | <6-8 | |||
FiO 2 | <0.40 | |||
Rate | 16-20 bpm | |||
SIMV + VG and PSV | ||||
PIP | <16 | 16-20 | 20 | |
PEEP | <6 | <7 | <8 | |
V T | 4-5 mL/kg | |||
PS | <6-8 | |||
FiO 2 | <0.40 | |||
Rate | 16-20 bpm |
P ¯ aw
, mean airway pressure; Δ P /amp, change in pressure (amplitude); PIP , peak inspiratory pressure; PEEP , positive end-expiratory pressure; PS , pressure support; PC-SIMV , pressure control–synchronized intermittent mandatory ventilation; PSV , pressure support ventilation; V T , tidal volume; VG , volume guarantee.
High-Frequency Ventilation
With HFOV the key is to achieve initial airspace recruitment and then to maintain optimal lung inflation and gas exchange at the lowest acceptable mean airway pressure ( <SPAN role=presentation tabIndex=0 id=MathJax-Element-3-Frame class=MathJax style="POSITION: relative" data-mathml='P¯aw’>P⎯⎯⎯awP¯aw
P ¯ aw
) ( Tables 23-5 and 23-6 ). The process for achieving this goal includes (1) an initial stepwise escalation in <SPAN role=presentation tabIndex=0 id=MathJax-Element-4-Frame class=MathJax style="POSITION: relative" data-mathml='P¯aw’>P⎯⎯⎯awP¯aw
P ¯ aw
to recruit atelectatic airspaces indicated by the ability to significantly reduce FiO 2 (commonly referred to as the “opening pressure” for the lung); (2) a subsequent stepwise reduction in <SPAN role=presentation tabIndex=0 id=MathJax-Element-5-Frame class=MathJax style="POSITION: relative" data-mathml='P¯aw’>P⎯⎯⎯awP¯aw
P ¯ aw
to a point at which FiO 2 needs to be again escalated to maintain targeted SpO 2 (commonly referred to as the “closing pressure” for the lung); and (3) increasing the <SPAN role=presentation tabIndex=0 id=MathJax-Element-6-Frame class=MathJax style="POSITION: relative" data-mathml='P¯aw’>P⎯⎯⎯awP¯aw
P ¯ aw
back above the closing pressure (typically by 2 to 3 cm H 2 O in surfactant-treated infants) to maintain an end-expiratory lung volume that allows effective gas exchange while minimizing pressure/volume effects on the cardiovascular system, thus “optimizing” oxygen delivery at the tissue/cellular level. A number of studies have described this approach using such measurements as SpO 2 , respiratory inductance plethysmography, high-resolution computed tomography (CT) scan, and forced oscillatory technique. Other than SpO 2 , these tools are not currently available in most practice settings. We typically provide early surfactant replacement therapy to all preterm infants intubated for RDS and then begin the process of optimizing lung inflation. We do not usually reduce <SPAN role=presentation tabIndex=0 id=MathJax-Element-7-Frame class=MathJax style="POSITION: relative" data-mathml='P¯aw’>P⎯⎯⎯awP¯aw
P ¯ aw
to closing pressure but more commonly will incrementally reduce the <SPAN role=presentation tabIndex=0 id=MathJax-Element-8-Frame class=MathJax style="POSITION: relative" data-mathml='P¯aw’>P⎯⎯⎯awP¯aw
P ¯ aw
by 1 to 2 cm H 2 O once FiO 2 has been reduced to <0.25 ( Table 23-7 ). Although radiographic lung volumes may not be ideal for assessing optimal lung inflation, when combined with clinical observations such as heart rate and blood pressure, as well as the temporal changes in FiO 2 and SpO 2, one can usually maintain adequate lung inflation and gas exchange while minimizing the risks of either overinflation or atelectasis.
Respiratory Disorder | Conventional Ventilation (Volume-Targeted, SIMV + PS, or A/C) | High-Frequency Ventilation |
---|---|---|
RDS | Surfactant therapy Volume target (V T ) 4-6 mL/kg Rate 30-60 bpm I-time 0.30-0.35 seconds PEEP 5-8 cm H 2 O PS to achieve ∼¾ set V T | Surfactant therapy Oscillator: Frequency 8-10 Hz; <SPAN role=presentation tabIndex=0 id=MathJax-Element-9-Frame class=MathJax style="POSITION: relative" data-mathml='P¯aw’>P⎯⎯⎯awP¯aw P ¯ aw 10-16; Δ P ∼2× <SPAN role=presentation tabIndex=0 id=MathJax-Element-10-Frame class=MathJax style="POSITION: relative" data-mathml='P¯aw’>P⎯⎯⎯awP¯aw P ¯ aw —adjust to vibrate chest/abd Jet: Rate 360-420; PEEP as needed to optimize lung inflation (typically 7-10); minimal or no backup rate |
MAS | Surfactant therapy; ±iNO V T 5-6 mL/kg Consider rate ≤30 I-time 0.35-0.50 seconds PEEP 4-7 cm H 2 O; set/adjust Based on lung inflation PS to achieve ∼¾ set V T | Surfactant therapy; ±iNO Oscillator: Frequency 6-8 Hz w/Δ P to vibrate chest/abd; <SPAN role=presentation tabIndex=0 id=MathJax-Element-11-Frame class=MathJax style="POSITION: relative" data-mathml='P¯aw’>P⎯⎯⎯awP¯aw P ¯ aw as needed for ∼9 rib lung inflations Jet: Rate 240-360; may need increased I-time; minimal or no backup rate; PEEP as needed Flow interrupter: Rate 240-360; convective rate 6-12; convective I-time ≥1 second; PEEP as needed |
Lung hypoplasia/diaphragmatic hernia | V T 4-5 mL/kg; PIP <26 cm H 2 O Rate 40-60 bpm I-time 0.25-0.40 seconds PEEP 4-6 cm H 2 O Surfactant only for RDS features | Oscillator: Frequency 8-10 Hz; <SPAN role=presentation tabIndex=0 id=MathJax-Element-12-Frame class=MathJax style="POSITION: relative" data-mathml='P¯aw’>P⎯⎯⎯awP¯aw P ¯ aw @ 10-13 depending on weight; Δ P ∼2× <SPAN role=presentation tabIndex=0 id=MathJax-Element-13-Frame class=MathJax style="POSITION: relative" data-mathml='P¯aw’>P⎯⎯⎯awP¯aw P ¯ aw —adjust to vibrate chest/abd; I:E 33% Jet: Rate 360-420; PEEP 5-8 cm H 2 O as needed to optimize lung inflation; minimal/no backup rate |
BPD, early/mild–moderate form BPD, chronic–severe form | Volume-targeted: V T 5-8 mL/kg; rate 20-40 bpm I-time 0.35-0.45 seconds PEEP 5-8 cm H 2 O PS to achieve ∼¾ set V T V T : May need 6-10 mL/kg (or higher) owing to increased dead space I-time 0.50-0.70 seconds; longer to overcome airway resistance Rate 20-30 bpm; slower to allow adequate lung emptying PEEP: Quite variable; may need 8-12 cm H 2 O to “stent” airway open | Oscillator: Similar to MAS Jet: Similar to MAS except consider minimal backup rate to optimize lung recruitment HFV not commonly applied for managing chronic–severe BPD; anecdotal reports suggest HFJV used with the “MAS approach” may be more effective than HFOV |
PPHN | iNO as indicated Avoid lung hyperinflation, correct atelectasis Adjunct therapies | iNO as indicated Optimize lung inflation, avoid both over- and underinflation Adjunct therapies |
P ¯ aw
, mean airway pressure; PEEP , positive end-expiratory pressure; PPHN , persistent pulmonary hypertension of the newborn; PS , pressure support; PIP , peak inflation pressure; Δ P , change in pressure (amplitude); RDS , respiratory distress syndrome; SIMV , synchronized intermittent mandatory ventilation; V T , tidal volume.
WEIGHT (G) | |||
---|---|---|---|
Mode | <1000 | 1000-2500 | >2500 |
HFOV | Initial Settings | ||
Rate | 10 Hz | 10 Hz | 8-10 Hz |
<SPAN role=presentation tabIndex=0 id=MathJax-Element-15-Frame class=MathJax style="POSITION: relative" data-mathml='P¯aw’>P⎯⎯⎯awP¯aw P ¯ aw (cm H 2 O) | 10-12 | 10-14 | 12-16 |
Δ P | 2 × <SPAN role=presentation tabIndex=0 id=MathJax-Element-16-Frame class=MathJax style="POSITION: relative" data-mathml='P¯aw’>P⎯⎯⎯awP¯aw P ¯ aw | 2 × <SPAN role=presentation tabIndex=0 id=MathJax-Element-17-Frame class=MathJax style="POSITION: relative" data-mathml='P¯aw’>P⎯⎯⎯awP¯aw P ¯ aw | 2 × <SPAN role=presentation tabIndex=0 id=MathJax-Element-18-Frame class=MathJax style="POSITION: relative" data-mathml='P¯aw’>P⎯⎯⎯awP¯aw P ¯ aw |
SIMV | Initial Settings | ||
Rate | 30-60 | 30-40 | 20-40 |
V T (mL/kg) | ∼5 | ∼5 | ∼5 |
PEEP (cm H 2 O) | 5-8 | 5-8 | 6-9 |
I-time (s) | Start at 0.3-0.4, adjust PRN based on graphics | ||
PS (cm H 2 O) | Start at 8-12, adjust to ∼⅔ PIP for V T |
P ¯ aw
, mean airway pressure; Δ P , change in pressure (amplitude); SIMV , synchronized intermittent mandatory ventilation; V T , tidal volume; PEEP , positive end-expiratory pressure; PRN, as needed; PS , pressure support; PIP , peak inspiratory pressure.
Parameter | Adjustment | |
---|---|---|
Rate | Typically no change in frequency except: | |
↓ if Δ P > 2-3 × <SPAN role=presentation tabIndex=0 id=MathJax-Element-20-Frame class=MathJax style="POSITION: relative" data-mathml='P¯aw’>P⎯⎯⎯awP¯aw P ¯ aw | ↑ if Δ P < <SPAN role=presentation tabIndex=0 id=MathJax-Element-21-Frame class=MathJax style="POSITION: relative" data-mathml='P¯aw’>P⎯⎯⎯awP¯aw P ¯ aw | |
<SPAN role=presentation tabIndex=0 id=MathJax-Element-22-Frame class=MathJax style="POSITION: relative" data-mathml='P¯aw’>P⎯⎯⎯awP¯aw P ¯ aw (cm H 2 O) | Increase/decrease as follows based on FiO 2 : | |
↑ by 2-3 if FiO 2 >50% | ||
↑ by 1-2 if FiO 2 25%-50% | ||
No change or ↓ by 1 if FiO 2 <25% | ||
↓ by 2-3 after surfactant therapy | ||
Δ P | Increase/decrease based on P co 2 or TcP co 2 : | |
↑ 5-10 if P co 2 >65 mm Hg | ||
↑ 2-5 if P co 2 55-65 mm Hg | ||
↓ 2-5 if P co 2 35-45 mm Hg | ||
↓ 5-10 if P co 2 <35 mm Hg |
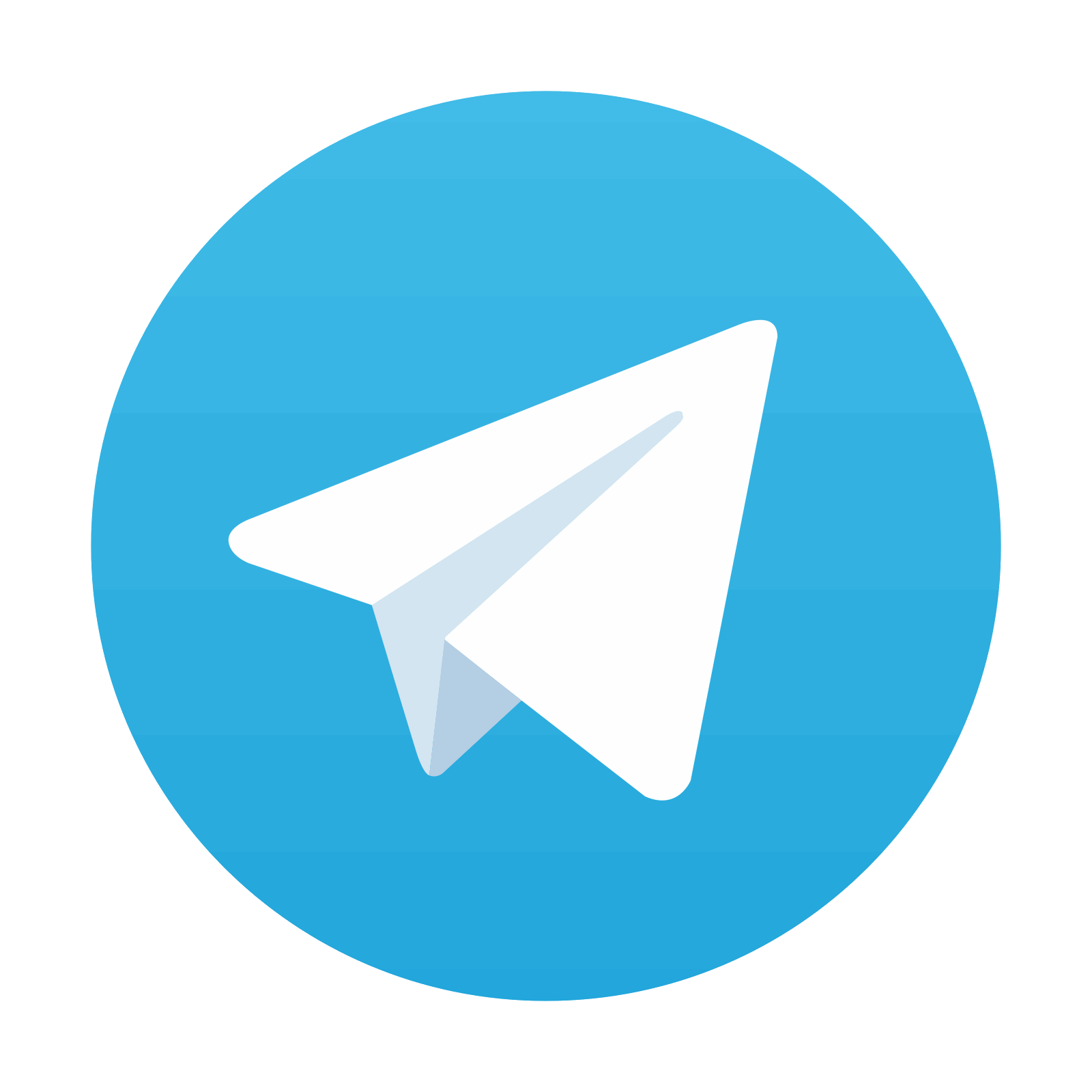
Stay updated, free articles. Join our Telegram channel

Full access? Get Clinical Tree
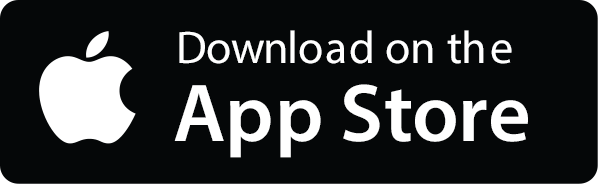
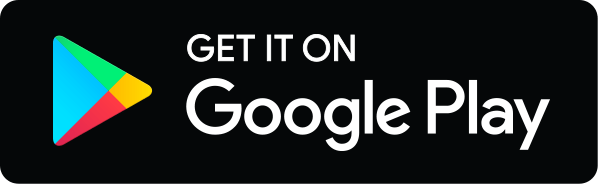
