KEY POINTS
- 1.
Neonatal encephalopathy is an alteration in consciousness or neurologic exam in newborn infants. Hypoxic-ischemic encephalopathy accounts for nearly 50% of all cases.
- 2.
Clinical presentation depends on the duration, timing, and severity of the insult and may evolve over the subsequent hours to days. Clinical staging of encephalopathy is usually based on the Sarnat criteria.
- 3.
Disruption of blood flow causes ischemic injury; abrupt disruption typically damages the basal ganglia and thalamus, whereas subacute, less severe loss of perfusion may damage the watershed areas.
- 4.
Patients may be stratified for risk usingfactors such as gestational age, encephalopathy scores, electroencephalography signatures,near infrared spectroscopy, imaging, and biomarkers.
- 5.
Current clinical management is largely supportive. Seizure control is important.Several potential therapeutic strategies areunder investigation, which brings hope for thefuture.
Definition, Diagnosis, and Differential
Definition
Neonatal encephalopathy (NE) is an alteration in consciousness or neurologic exam in the neonate. The possible etiologies of NE are broad ( Table 47.1 ) but it is most commonly (approximately 50%) caused by hypoxic-ischemic encephalopathy (HIE). , The following sections are focused on HIE, although many details are applicable to other NE etiologies.
Hypoxic-ischemic encephalopathy |
Metabolic derangements (inborn errors of metabolism, hypoglycemia) |
Intracranial hemorrhage |
Perinatal stroke |
Kernicterus |
Infection |
Sinovenous thrombosis |
Maternal toxins |
Diagnosis
The initial diagnosis of HIE relies on evidence of an acute or subacute (prolonged) perinatal event leading to brain injury and exam findings consistent with encephalopathy. Initial evaluation of an infant with suspected HIE should include perinatal history, neurologic exam, and in some cases, electroencephalogram (EEG) and imaging, when available.
Perinatal History
Evidence of intrauterine distress such as an abnormal biophysical profile, decreased fetal movements, fetal bradycardia, or acidosis on umbilical cord/initial infant blood gas (within 1 hour of birth), along with need for extensive resuscitation and/or low Apgar scores (<5) at 5 and 10 minutes, are needed for the diagnosis. ,
Neurologic Examination
The clinical presentation depends on the duration, timing, and severity of the insult and may evolve over the subsequent hours to days. Although it is not the only scoring system, clinical staging of encephalopathy is often based on the Sarnat criteria ( Table 47.2 ). Using the modified Sarnat criteria, which do not include EEG, the current recommendations are to offer therapeutic hypothermia (TH) to infants with moderate to severe encephalopathy, although the efficacy of TH in severe encephalopathy is the subject of ongoing studies (see Chapter 32 ). The Sarnat score was used as an inclusion criterion in the first randomized controlled trials of TH , , and is still used by most neonatal intensive care units for evaluation of the neonate needing TH. Longitudinal follow-up of Sarnat scoring suggests that worsening staging regardless of the initial staging is more predictive than the initial score alone.
Sarnat Score From 1976 | Thompson Score From 1997 | Encephalopathy Score From 2004 | |
---|---|---|---|
Scoring | Three stages of encephalopathy: mild, moderate, severe | Score 0 (best) to 2 or 3 (worst) on each component (max 22, >7 moderate-severe encephalopathy) | 6 categories, scored 0–1 (total possible score 6) |
Components | |||
Consciousness | X | X | X |
Reflexes | X | X | X |
Tone | X | X | X |
Autonomic function | X | ||
Seizures/EEG | X a | X | X |
Respiratory drive | X | X | |
Fontanelle | X | ||
Feeding | X | ||
Prognostic use | Higher stage associated with “major disability” during early adolescence Associated with 18-month ND outcomes | Day 3 score >15 with high specificity (96%) for abnormal outcome at 1 year (sensitivity 71%, PPV 92%, NPV 82%) Day 1 score linked with mortality and morbidity Peak score associated with IQ, motor outcome, survival without ND impairment in 4- to 5-year-olds | Higher score associated with worse 30-month ND outcomes Max score in first 3 days of age highly associated with outcome |
a Modified Sarnat staging does not include seizures/EEG findings. EEG, Electroencephalogram; ND , Neurodevelopmental; NPV , Negative predictive value; PPV , Positive predictive value.
EEG
EEG was part of the originally described Sarnat score, but it is not included in the modified version, now the most often used. Regardless, in cases where there is clinical suspicion for HIE but the neurologic examination is equivocal, early EEG is useful for encephalopathy staging. Early EEG (<6 hours after birth) was used as an inclusion criterion for many of the early randomized controlled trials on TH. , A normal or mildly abnormal EEG background is highly predictive of normal neurodevelopmental outcomes at age 2 years.
Pathophysiology
Acute Phase
The fetal brain requires blood flow to deliver oxygen and glucose for cellular energy and metabolic homeostasis. Interruption of blood flow leads to hypoxemia and eventually decreases cardiac output. Depending on the severity and timing of the disruption of blood flow, cerebral injury may occur and lead to HIE. Abrupt disruption of cerebral blood flow is classically associated with deep grey matter (basal ganglia and thalamus) injury. Chronic and less severe (partial) disruption of cerebral blood flow is associated with cortical injury, particularly in the watershed regions.
The initial disruption of cerebral blood flow leads to mitochondria failure. The resulting adenosine triphosphate (ATP) depletion and lactic acid build-up impairs the function of excitatory amino acid transporters within the astrocytic membrane forming the synaptic cleft. As a result, excitatory amino acids such as glutamate accumulate and activate N-methyl-D-aspartate and α-amino-3-hydroxy-5-methyl-4-isoxazolepropionic acid receptors, leading to an intracellular influx of sodium, calcium, and water. Cell death results from the progression of cytotoxic edema, protease activation, and free radical production ( Fig. 47.1 ).

Latent/Chronic Phase
Restoration of cerebral blood flow, or reperfusion, after disruption and injury begins the latent phase of injury. The latent phase is the therapeutic target of TH. Secondary energy failure, which signals the end of the latent phase, is marked by irreversible mitochondrial failure and involves oxidative stress, intracellular calcium accumulation, inflammation, and cell death. This neuronal death includes cell death along the entire cell death continuum, including apoptosis, autophagy, continuum cell death, necroptosis, and necrosis. Many of the therapeutic agents tested in the past decade have targeted many of these downstream mechanisms ( Figs. 47.2 and 47.3 ).


Stratification of Patients by Risk
Gestational Age/Prematurity
HIE is classically reported in full-term and late preterm infants and rarely in preterm infants. Although HIE occurs at all gestational ages, the assessment of encephalopathy in preterm infants is difficult and the clinical presentation of seizures may be subtle. There have been several small studies on preterm HIE, , which have found that due to differences in neurodevelopmental stage, injury mechanisms and patterns differ from those of the full-term neonate. Oligodendrocyte maturity, vascular and blood-brain barrier permeability, and selective vulnerability of certain cell lines such as developing oligodendroglia, astrocytes, and microglia likely determine the severity and type of injury. Preterm infants appear to exhibit greater susceptibility to white matter injury, most often associated with concurrent basal ganglia and thalamic injury, than do full-term infants (see Fig. 47.8 ahead).
Encephalopathy Score
Multiple scoring systems have been developed to assess severity of encephalopathy and predict long-term risk of neurodevelopmental disability (see Table 47.2 ). The first of these was the Sarnat scoring system. Clinically, in settings where other prognostic tools (magnetic resonance imaging [MRI], EEG) can be used, these scores are most commonly used to assess eligibility for TH. Regardless of the scoring or staging system used, trending the score/exam over time while in the acute period is important, because the examination will evolve over time and persistently high encephalopathy scores are generally predictive of worse outcomes. Of note, administration of sedation and/or antiepileptic drugs may alter the exam, which should considered.
Findings of moderate or severe encephalopathy on exam are inclusion criteria for treatment with TH; traditionally, children with mild encephalopathy were not thought to be at significant risk for neurodevelopmental disability, but there is growing evidence that neonates with mild encephalopathy may be at risk for long-term neurodevelopmental abnormalities. Neonates with mild HIE have been described to have abnormal short-term outcomes such as longer time to reach full feeds, seizures, need for a surgical feeding device, or abnormal MRI or neurologic exam findings at discharge. In a retrospective analysis of infants who had received TH, MRI abnormalities after TH were equally common among infants with mild HIE compared with those with moderate or severe HIE. Mild encephalopathy on early EEG is associated with lower IQ at age 5 years. The PRIME (Prospective Research in Infants with Mild Encephalopathy) study revealed that over half of infants with mild HIE had an abnormal short-term outcome. A recent meta-analysis showed that 20% of infants with mild encephalopathy have adverse neurologic outcomes at 18 months. Study of long-term outcomes in infants with mild HIE as well as the efficacy of TH for this group is ongoing.
Amplitude Integrated EEG/Continuous Video EEG
Encephalopathy on neonatal EEG has been standardized by the American Clinical Neurophysiology Society. Depending on available resources and setting, amplitude integrated EEG (aEEG; Fig. 47.4 ) or full montage EEG can be used ( Table 47.3 ). A normal or mildly abnormal early EEG background is highly predictive of normal neurodevelopmental outcomes at age 2 years. Recovery of EEG background within the first 24 hours is associated with better outcomes. Persistently abnormal EEG background activity 36 to 48 hours after injury has been associated with worse neurodevelopmental outcomes. aEEG is used by units particularly when continuous full montage neonatal EEG services are not available for monitoring throughout TH. A systematic review of aEEG use in HIE including 17 studies (both with and without TH) concluded that the aEEG background during the first 72 hours after HI injury has strong predictive value for long-term neurologic outcome.

Amplitude Integrated EEG | Full Montage EEG | |
---|---|---|
Measurement | Superficial, biparietal electrodes Derived from reduced EEG | Multielectrode array Greater depth of detection |
Advantages | Greater accessibility—unit staff can place electrodes and interpret information | Greater information (more channels, depth of detection) |
Disadvantages | Limited information provided (few electrodes, reduced depth) | Requires specialized staff to place electrodes and interpretation by a neurologist |
Ideal clinical scenario | During transport Limited-resource settings | Larger centers with support staff and neurologists available |
Near-Infrared Spectroscopy
Near-infrared spectroscopy (NIRS) is a bedside monitoring technique that continuously provides information on mixed arterial and venous saturations in the brain. In some cases cerebral saturations may be compared with control tissue such as the kidney ( Fig. 47.5 ). Mixed saturation (rSO 2 ) is a measure of the differential between oxygen delivery to the brain tissue and oxygen extraction by the brain tissue. Small studies have examined the use of NIRS in infants with HIE undergoing TH, with varying results. Higher rSO 2 values in the first 10 hours of TH were associated with injury on MRI ; however, other studies have not found a relationship between rSO 2 values during TH and 18-month outcomes. Ongoing studies are examining the use of NIRS both in guiding management of blood pressure and cerebral autoregulation as an neuroprotective strategy and as a prognostic tool and in trending a number of NIRS parameters as a prognostic tool. ,

Imaging
Head Ultrasound
Although much of the literature regarding imaging in HIE has focused on MRI, head ultrasound has been shown to be a useful tool for prognosis and evaluation of severity. Signs of HI injury that may be detectable on head ultrasound include small, effaced ventricles, which may be indicative of cerebral edema (although they can be a normal variant); echogenicity of the basal ganglia and thalamus can also be a sign of ischemia. Doppler measurements of the anterior cerebral artery resistive index have been associated with 2-year neurodevelopmental outcomes. , The ratio of white matter to gray matter echogenicity has also been described as a prognostic tool ( Figs. 47.6 and 47.8 ).

Magnetic Resonance Imaging
When available, MRI is the gold standard imaging technique in neonates with HIE. A specific MRI protocol for neonates is important and should be discussed with pediatric neuroradiology. Depending on the timing of imaging after injury, findings will be present on different sequences. Restricted diffusion on diffusion weighted imaging (DWI) will be present for the first 3 to 5 days after injury, with the exact timing dependent on the severity of injury. Evolution of the MRI findings occurs over the first several weeks; nearing the end of the first week, pseudonormalization may occur. Pseudonormalization of the MRI is when the diffusion weighted changes are no longer visible and injury on T1/T2 sequences are not yet apparent. , There are two major patterns of HI injury, depending on the length and severity of the insult. Acute, more complete compromise results in basal ganglia-thalamic involvement, and more prolonged, less severe compromise is associated with a watershed/cortical injury pattern. In severe, prolonged hypoxic-ischemic events, extensive HI injury, which affects both the deep gray matter and the cortex, may be present. EEG and MRI may be useful in preterm infants to assess long-term risk for neurodevelopmental deficits.
Certain findings and scoring systems on MRI in HIE have been predictive of outcome. One highly predictive finding on MRI is abnormalities of the apparent diffusion coefficient in the posterior limb of the internal capsule, which is highly associated with survival and neuromotor outcome in infants with HIE. One MRI scoring system, specifically the grey matter injury score, is highly predictive of adverse motor and cognitive outcomes at age 2 and at school age. Conversely, absence of injury on MRI is not necessarily predictive of a favorable outcome, because infants with minimal or no injury on MRI may still have moderate to severe delays (see Figs. 47.6 , 47.7, and 47.8 ).


Biomarkers
The search for a reliable, testable biomarker for outcome in HIE is the topic of ongoing research. A number of serum biomarkers have undergone investigation, with mixed prognostic utility. Those with the greatest evidence are summarized in Table 47.4 . Ongoing trials are examining various biomarkers for feasibility of testing, specificity, and sensitivity for long-term neurodevelopmental outcomes (BiHiVE2 NCT 02019147; BANON study NCT03357250).
Biomarker | Outcome Association |
---|---|
Brain derived neurotrophic factor (BDNF) | 12 months ND outcomes |
Glial fibrillary acidic protein (GFAP) | MRI injury , Moderate to severe HIE , Abnormal Bayley III scores at 5–10 months and 15–18 months |
S100B | MRI injury score Clinical grade of encephalopathy |
Myelin basic protein (MBP) | |
Ubiquitin carboxy-terminal hydrolase-L1 (UCH-L1) | MRI injury score , Clinical grade of encephalopathy , , Abnormal Bayley III scores at 5–10 months |
Tau protein | MRI injury score 12 months ND outcomes , |
Phosphorylated axonal neurofilament heavy chain (pNF-H) | MRI injury |
Interleukin (IL)-1β | MRI injury score , Abnormal Bayley III scores at 15–18 months |
IL-6 | MRI injury score , Abnormal Bayley III scores at 15–18 months Death or severe MRI injury |
IL-8 | Abnormal Bayley III scores at 15–18 months |
IL-10 | MRI injury score , Death or severe MRI injury |
IL-13 | MRI injury score , |
IL-16 | All perinatal asphyxia, including those who develop HI; did not correlate to grade of HIE or 2-year ND outcomes |
Interferon (IFN)-γ | Abnormal Bayley III scores at 15–18 months , |
Tumor necrosis factor (TNF)-α | Abnormal Bayley III scores at 15–18 months , |
Vascular endothelial growth factor (VEGF) | Abnormal Bayley III scores at 15–18 months |
Transport
Neonates with HIE should be cared for at a tertiary care center in order for appropriate monitoring, treatment, and follow-up to occur. Because subclinical seizures are common in this population, especially after the administration of antiepileptic drugs, continuous EEG monitoring is crucial throughout TH and rewarming. EEG monitoring with either full montage neonatal EEG or aEEG is important, as is interpretation by a pediatric neurologist. In addition, the ability to obtain MRI with a neonatal-specific sequence protocol is important, and interpretation with pediatric neuroradiology maximizes yield from the study. Besides pediatric neurology and neonatology, involvement of other pediatric specialists in the care and follow-up of infants with HIE is important and should include developmental pediatrics, pediatric neuropsychology, pediatric therapies (physical therapy, occupational therapy, and speech-language pathology), and pediatric neurology.
Transport of infants with HIE undergoing TH has a special set of challenges. Prompt initiation of TH is key and has been linked to better outcomes. For infants requiring TH who are born at referral hospitals, this means cooling should be initiated prior to and during transport to a tertiary care center. , Caution should be exercised in providing passive cooling, because infants, particularly those with severe encephalopathy, may become severely hypothermic. , If possible, active cooling during transport is optimal, because this is associated with reaching target temperatures quicker and maintaining the temperature in the goal range. ,
Supportive Management
Multiorgan dysfunction is present in up to 50% to 88% of infants with HIE. A summary of supportive management by system is presented in Table 47.5 .
Associated Diagnosis/Comorbidity | Pathophysiology/Clinical Signs | Management | |
---|---|---|---|
Respiratory | Meconium aspiration syndrome/respiratory failure | Meconium-stained amniotic fluid → aspiration Respiratory distress/hypoxemia | Support oxygenation and ventilation to achieve normoxemia and normocarbia Adjust blood gas values based on temperature during TH |
Central apnea | Injury to respiratory control centers (brainstem injury—severe HI injury) Respiratory depression secondary to medications (antiepileptic, sedation) Seizures | Mechanical ventilation Close monitoring of blood gases | |
Cardiovascular | Myocardial ischemia and dysfunction | Ischemia to myocardium → ventricular dysfunction → shock/hypotension Elevated cardiac enzymes (troponin I or CKMB) | Echocardiogram Pressor support for hypotension/shock |
Persistent pulmonary hypertension | Initial hypoxia prevents normal relaxation of pulmonary vascular bed → pulmonary hypertension Hypoxemia associated with longer length of stay and higher mortality | Pulmonary vasodilators Extracorporal membrane oxygenation | |
Fluid, electrolytes, nutrition, gastrointestinal | Fluid overload | Fluid resuscitation Acute kidney injury | Gentle fluid restriction (60–80mL/kg/day) Monitor urine output, electrolytes, and weight |
Nutrition/feeding | Ischemic gut injury | NPO Total parenteral nutrition Some evidence that small-volume enteral feeds may be safe in stable infants during TH , | |
Hepatic injury | Ischemic liver injury → elevated liver enzymes (peak 24–72 hours after injury, normalize in 6–12 days) , Synthetic dysfunction → coagulopathy | Monitoring liver enzymes and coagulation studies Correct coagulopathy with products | |
Electrolyte disarray , | Hypo- or hyperglycemia (adrenal insufficiency, or stress response) Hypocalcemia (intracellular influx during excitotoxicity) Hypercalcemia (secondary to decrease influx in patients receiving TH) Hypomagnesemia (consumption or renal loss) | Aim for euglycemia Both hypo- and hyperglycemia have been associated with worse ND outcomes , Electrolyte management with supplementation and TPN | |
Hematology | Coagulopathy | Liver injury → synthetic dysfunction → coagulopathy | Closely monitor clotting studies in the first 48–72 hours (PT, PTT, INR, fibrinogen) Transfuse products (FFP, cryoprecipitate) as needed Goal INR <2 or if symptomatic |
Thrombocytopenia | Bone marrow suppression secondary to ischemia Effects of therapeutic hypothermia | Monitor platelet counts Transfuse for platelets <50,000 or bleeding (transfusion thresholds unit dependent and controversial) | |
Leukopenia | Bone marrow suppression secondary to ischemia | Monitor CBC with differential | |
Renal | Acute kidney injury | HI injury to kidney → ATN → oliguria → fluid overload and electrolyte disarray , , | Close monitoring of urine output, electrolytes, weight, and creatinine (peaks in first 24–48 hours, declines over next week) Gentle fluid restriction Nephrology consultation and follow-up , AKI associated with worse ND outcomes , |
Syndrome of inappropriate antidiuretic hormone | CNS HI injury → disordered release of ADH → oliguria/hyponatremia | Fluid restriction and sodium correction Close monitoring of urine output, electrolytes, weight | |
Neurologic | Shivering/sedation | Shivering during TH—may increase metabolic rate → counteract neuroprotective effects of TH , Agitation (especially in acute phase of mild HIE) | Use of routine sedation is controversial and practices vary Morphine is most commonly used and some data show improved ND outcomes Other medications (clonidine, dexmedetomidine) are being studied , |
Immunologic, infectious disease | Sepsis | Maternal and neonatal infection is a risk factor for/is associated with HIE NE has been linked to worse injury , and ND outcomes TH may not be as neuroprotective in the context of infection | Infectious work-up as clinically indicated |
Neuroprotective Strategies
Seizure Control
Status epilepticus, seizures lasting >5 minutes, or more than two 30-second seizures per hour are accepted guidelines to start antiseizure drugs, particularly when encephalopathy is secondary to stroke. Phenobarbital is the most common agent used to control seizures in this setting, but evidence guiding the duration and the combination with other antiseizure drugs is limited. One of the few points of agreement among experts is that the duration of phenobarbital (and phenytoin) treatments should be as brief as possible after controlling the initial seizures, due to the suspected harmful effects on the developing brain. Between 45% and 70% of clinical seizures respond to phenobarbital (loading of 20 mg/kg), and its metabolism is grossly unchanged by TH. In rodent models of neonatal HI injury, the addition of phenobarbital to TH may provide additional protection concerning the early extent of the injury, although it does not prevent motor impairments. This lack of motor protection may be because phenobarbital, along with valproate and phenytoin, produces increased neuronal loss, even without a superimposed brain insult. Thus, other alternatives such as levetiracetam and topiramate, which do not have those effects, are being explored in the developing brain.
Levetiracetam (Roweepra, Spritam, and Keppra)
Although levetiracetam appears to be as effective as phenobarbital in controlling pediatric seizures, the preliminary results of the NEOLEV2 trial ( www.clinicaltrials.gov ; NCT01720667), in which 280 infants diagnosed with neonatal seizures were randomized to phenobarbital or levetiracetam, suggested superior effectiveness of phenobarbital in achieving 24-hour seizure control (80% versus 28%). Regardless of those findings, levetiracetam may provide neuroprotective effects not fully linked to the control of seizures. Although still controversial, levetiracetam does not appear to increase neuronal death as valproate, phenobarbital, and phenytoin do, and in fact it decreases cell death and injury after neonatal hypoxic-ischemic (HI) brain insult in rodents. Furthermore, levetiracetam used after termination of status epilepticus with benzodiazepines provides neuroprotection against functional failure of the blood-brain barrier and neuroinflammation in rodents. Accordingly, the antiseizure effect of benzodiazepines, specifically diazepam, is enhanced by levetiracetam in rodent models of status epilepticus. Of note, the metabolism of levetiracetam during the first 7 days of life changes significantly. The mean half-life of levetiracetam, after a 20- to 40-mg/kg bolus followed by 5 to 10 mg/kg/day as maintenance, decreases between day 1 and 7 of life from 18.5 to 9.1 hours, suggesting that more frequent dosing is required in older infants to maintain stable levels in blood. The effects of TH on the pharmacokinetics of levetiracetam in neonates with NE, specifically HI insult, are unknown. Currently there are no randomized controlled trials (RCTs) studying the neuroprotective effects of levetiracetam in patients suffering from HIE.
Topiramate (Trokendi XR, Qudexy XR, and Topamax)
Case reports suggest that topiramate controls neonatal seizures refractory to phenobarbital and other first-line antiseizure agents. , Similar to levetiracetam, topiramate has demonstrated neuroprotective effects , if used immediately after injury at doses of 20 mg/kg, followed by maintenance at 10 mg/kg, in small and large animal models of neonatal HI brain injury. , Of note, high doses of topiramate (50 mg/kg) increase neuronal death in the white matter after neonatal HI in some of these studies. , From the few human studies, it is known that the metabolism of topiramate at a dose of 5 mg/kg/day is not altered by moderate hypothermia and does not have significant short-term adverse effects. Except for incidence of epilepsy, which is lower in patients treated with topiramate during TH (NeoNATI trial, NCT01241019), there are no differences in the degree of injury on MRI or in blindness, hearing loss, or neurodevelopmental disability at 18 to 24 months of age. However, the most significant limitation of this RCT is the small sample size (n = 44); thus the result of the other ongoing phase 1 and phase 2 RCTs (NCT01765218) may provide additional information about the safety and neuroprotective effects of this drug.
Shivering Control
Shivering exacerbates brain injury in large animal models of neonatal HI brain injury, likely due to increased glucose metabolism. Morphine is often used to control shivering in the setting of NE in many neonatal intensive care units, following the protocols used in large RCTs. However, opiates do not specifically target the mechanism of shivering and have significant adverse effects including respiratory depression, hypotension, and later withdrawal, effects that are potentiated due to impaired clearance during TH after an HI insult. α-2-Agonist agents such as clonidine or dexmedetomidine control shivering and thus may be potential adjuvant therapies to TH. , Although studies are limited, treatment with clonidine has been shown to decrease brain injury after neonatal HI in rodents. Similarly, neonatal clonidine treatment delays the development of epilepsy kindling in rodent models. One phase 1/2 RCT studying clonidine in NE has been completed supporting the safety of intravenous (IV) clonidine up to 1 μg/kg/dose every 8 hours in neonates receiving TH, with decreased need for morphine PRN (NCT01862250), and a second RCT is still enrolling (SANNI project, NCT03177980).
A second α-2-agonist agent under evaluation is dexmedetomidine. The results in different rodent models of perinatal brain injury and analysis of neurodegeneration suggest dexmedetomidine has neuroprotective properties, with an unknown link to the control of shivering. However, in a piglet model of neonatal HI, dexmedetomidine at clinically relevant doses (loading of 2 μg/kg at 10 minutes followed by 0.028 μg/kg/h for 48 hours) and plasma levels (within 1 μg/L) in combination with 48 hours of TH produced significant cardiovascular instability, increased mortality, and worsened neuronal death. Additionally, in a case report, dexmedetomidine (without TH) was temporally associated with development of seizures in an infant diagnosed with NE. An early phase 1 clinical study assessing the pharmacokinetics of dexmedetomidine (the Cool DEX study; NCT02529202) demonstrated slower rise in dexmedetomidine in patients receiving TH, with a good safety profile up to a dose of 0.4 μg/k/h. Until more safety data are available, caution must be exercised in the routine use of dexmedetomidine and clonidine.
Future Adjuvant Therapies
A search in the World Health Organization’s International Clinical Trials Registry Platform ( http://apps.who.int/trialsearch/default.aspx , which includes studies in the US-based www.clinicaltrials.gov ) using the search term “neonatal encephalopathy” provided information about 36 unique active clinical trials worldwide ( Table 47.6 ). Therapies with greater progress toward translation to the clinical arena are summarized in more detail in the following sections and in Figs. 47.2 and 47.3 .
NCT Number (Date of Completion) | Phase Sample Size Randomized (Masking) | Primary Outcomes | Secondary Outcomes | References |
---|---|---|---|---|
Epoietin | ||||
NCT02811263 (09/22/2019) | 3 500 Y (Q) | Death or neurodevelopmental impairment at 22–26 months of age |
| Wu et al. Wu et al. Juul et al. |
NCT01913340 (09/01/2016) | 1 & 2 50 Y (Q) | Markers of organ function |
| Wu et al. Wu et al. Mulkey et al. |
NCT00719407 (11/01/2012) | 1 24 N | Serious adverse event within 14 days of life |
| Wu et al. Rogers et al. Shankaran et al. |
NCT02499393 (12/01/2014) | 2 & 3 75 Y (N) | Death to discharge |
| Merchant et al. |
NCT03163589 (06/01/2020) | 3 40 Y (N) | Death or long-term major neurodevelopmental disability according to Griffith score at age 1 |
| Murray et al. Frymoyer et al. Kurinczuk et al. Jacobs et al. Zacharias et al. Zhu et al. Elmahdy et al. Cirelli et al. Bednarek et al. |
NCT03079167 (12/01/2021) | 3 300 Y (Q) | Composite measure of death or moderate/severe disability at age 2 |
| No results posted |
NCT01732146 (12/01/2017) | 3 120 Y (T) | Survival without neurologic sequelae at age 2 |
| Zhu et al. Goodarzi et al. |
NCT01471015 (01/01/2014) | 1&2 30 Y (T) | Pharmacokinetic profile of darbe after the first and second dose |
| Roberts et al. Baserga et al. Rogers et al. Rangarajan et al. Davidson et al. Juul et al, Nair et al. Larpthaveesarp et al. Messier et al. Schober et al. |
NCT03071861 (No reported) | 2 40 Y (Q) | Neurodevelopmental outcome at age 1 |
| No results posted |
Caffeine | ||||
NCT03913221 (06/01/2021) | 1 18 N | Area under plasma concentration-time for caffeine |
| Shankaran et al. |
Magnesium sulfate | ||||
NCT02499393 (12/01/2014) | 2 & 3 75 Y (N) | Death |
| Davidson et al. |
Sildenafil | ||||
NCT02812433 (06/01/2022) | 1 80 Y (Q) | Serious adverse events between day 1–14 |
| No results posted |
Autologous cord blood | ||||
NCT00593242 (01/01/2017) | 1 52 N | Adverse event rates |
| Cotten et al. |
NCT02256618 (02/01/2018) | 1 6 N | Adverse event rates during the first 3 days of life |
| Tsuji et al. Ohshima et al. Taguchi et al. |
NCT02551003 (12/01/2019) | 1 & 2 60 Y (S) | Mortality and disability rate |
| No results posted |
NCT02881970 (09/01/2020) | 1 & 2 20 N | Adverse clinical or paraclinical event rates |
| No results posted |
NCT02434965 (1/01/2022) | 2 20 N | Infusion reaction as a measure of safety and tolerability |
| No results posted |
NCT03352310 (12/01/2020) | 1 40 N | Mortality rate Change from baseline hematocrit |
| No results posted |
Melatonin | ||||
NCT02621944 (01/01/2022) | 1 40 N | Tolerance to maximum dose of melatonin Bayley-III Index Scores Peak plasma concentration (Cmax) of melatonin |
| No results posted |
NCT03806816 (12/01/2022) | 1 & 2 100 Y (S) | Bayley III scale |
| No results posted |
Allopurinol | ||||
NCT03162653 (12/01/2020) | 3 846 Y (Q) | Death or severe neurodevelopmental impairment versus survival without severe neurodevelopmental impairment at age 2 |
| Maiwald et al. |
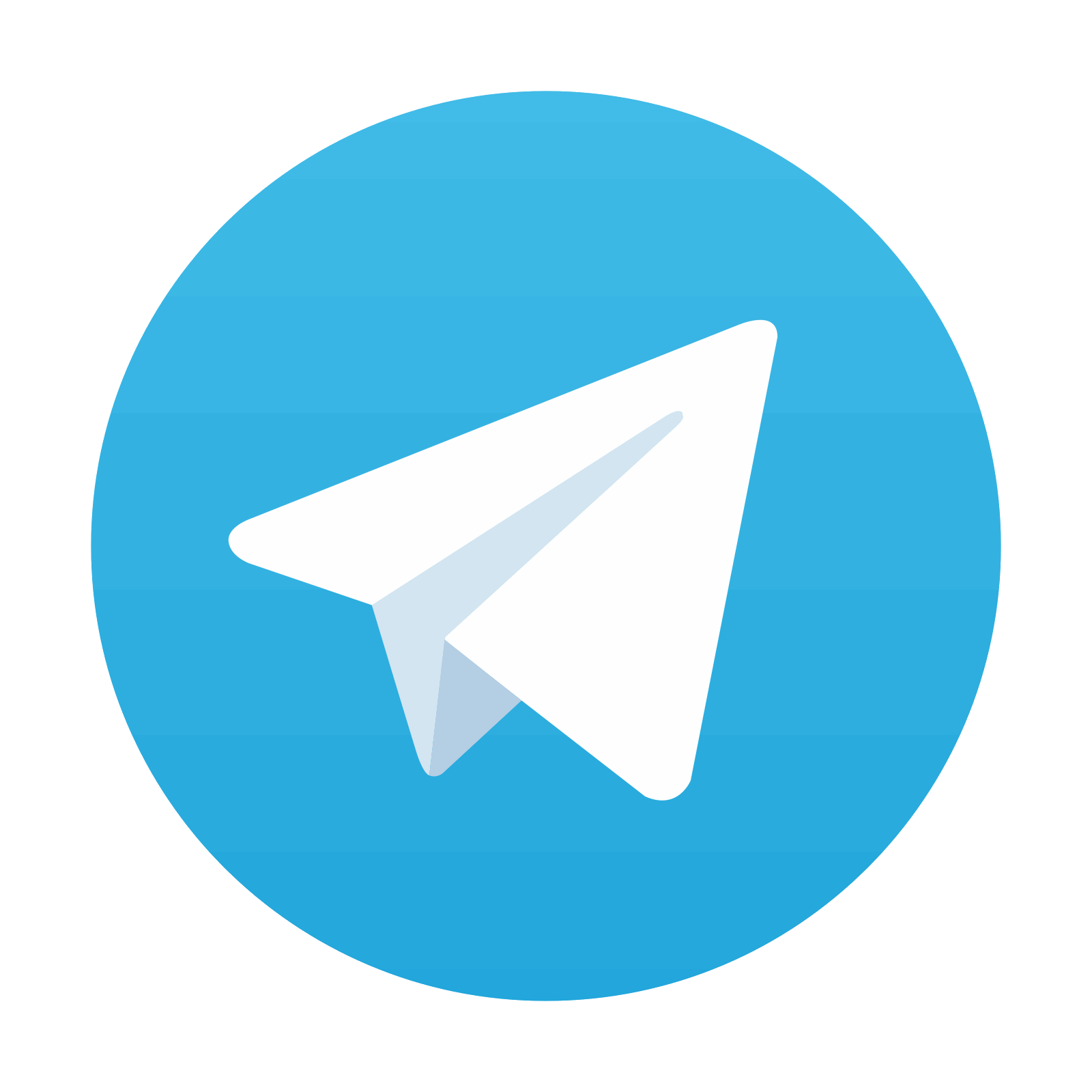
Stay updated, free articles. Join our Telegram channel

Full access? Get Clinical Tree
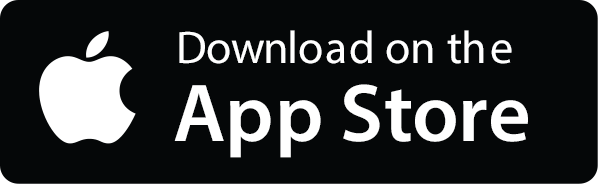
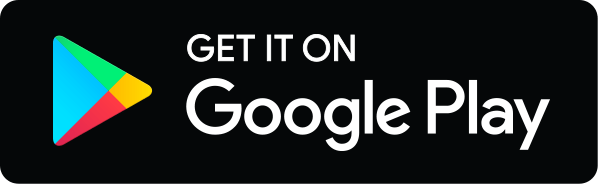
