Fig. 17.1
GMFCS levels. Reproduced with permission from copyright © Kerr Graham, Bill Reid, and Adrienne Harvey, The Royal Children’s Hospital, Melbourne
CP is also classified by the type of abnormal tone or movement disorder. The location and extent of brain involvement account for the type and severity of the abnormal tone and movement disorder as well as the topographical distribution of involvement of the trunk and limbs. Lesions involving the pyramidal tract are associated with spasticity or velocity-dependent hypertonia, whereas lesions of the basal ganglia are associated with dyskinesias including dystonia or choreoathetosis. Dystonia is characterized by jerky involuntary movements of the limbs or trunk and neck. Choreoathetosis is characterized by involuntary writhing movements. Cerebellar involvement is associated with ataxia and hypotonia. Some children have features of more than one type or “mixed”-tone abnormalities. The commonly used topographical classification, hemiplegic, diplegic (lower extremities involved more than upper extremities), and quadriplegic or total body involved, has some clinical and prognostic utility, but these terms are not very reliable. Instead, the regional involvement may be categorized as being “unilateral” or “bilateral,” which can be symmetric or asymmetric with one side more involved than the other.
Pathophysiology of Musculoskeletal Deformity in Cerebral Palsy
Musculoskeletal deformities in CP are acquired secondarily. Characteristically, upper motor neuron disorders are associated with hypertonia, hyperreflexia, and co-contraction of agonist–antagonist muscles. Other features can include loss of selective motor control, poor centrally mediated balance and coordination, and muscle weakness [6]. These children often have a delay in the development of the motor milestones.
Hypertonia is associated with “dynamic” muscle contractures. Muscles are taut due to the increased muscle tone, but they are of sufficient length. Muscles typically grow in response to the stimulus of stretch. In a child with CP, developmental delay prevents the child from engaging in the typical physical activities that would provide the source of stretch. The hypertonia further impedes the extent of any stretch. Consequently, muscle growth is impaired relative to bone growth. This results in the development of “static” contractures where the muscles are both tight and too short [7]. The acquisition of milestones, from rolling over into the prone position, sitting upright, crawling, pulling up to stand, cruising, and walking, typically occurs during the first 12–15 months of a child’s life. The growing skeleton undergoes significant changes during this time, in response to the natural stresses applied to the skeleton. The delay in motor milestones, or in some cases failure to achieve them at all, deprives the skeleton of these important corrective forces, resulting in the retention of infantile morphology of the skeleton (e.g., increased femoral anteversion and coxa valga in the proximal femur). Furthermore, the abnormal muscular forces acting on the growing skeleton contribute additionally to the development of bony deformities and joint instability over time. Joint instability in these patients is often a consequence of both the bony deformity and the unbalanced forces of the agonist–antagonist muscles that bridge across a joint. The skeletal deformities result in lever arm dysfunction [8]. In the lower extremity, the interaction of joint contractures, muscle weakness, bony deformities, and joint instability at multiple levels is associated with inefficient (higher energy consuming) pathologic and compensatory gait patterns in ambulant children. This leads to limitation in physical function or increase in symptoms such as pain and fatigue. In nonambulatory children, contractures and deformities of the lower limbs contribute to discomfort; difficulties with care, positioning, and mobility; and poorer quality of life. Although the primary lesion in the brain is not progressive, the secondary musculoskeletal consequences become worse with growth [9–12] (Fig. 17.2).
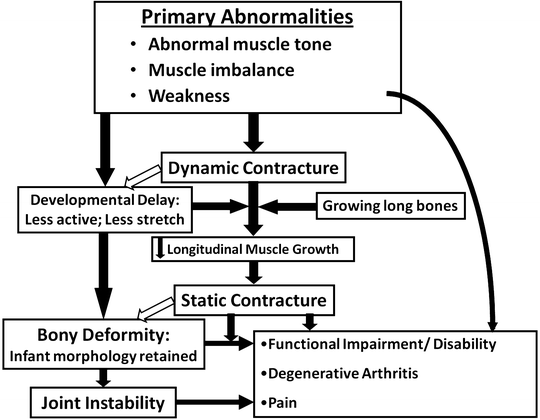
Fig. 17.2
Pathway to secondary musculoskeletal pathology in cerebral palsy. Adapted from Noordin S, Narayanan U. Neuromuscular Disorders, Chapter 64. In: Mercer’s Textbook of Orthopedics and Trauma, 10th Edition. Boca Raton: CRC Press; 2012
Specific Deformities of the Lower Extremity
Pelvis and Hip
Across the hip joint, adductors and flexor muscle groups are typically more involved, overpowering their antagonist counterparts, the hip abductors and hip extensors. This muscle imbalance often results in adduction and flexion contractures of the hip in the coronal and sagittal planes, respectively. The hip flexion contracture arising from the iliopsoas can be accompanied by anterior pelvic tilt with associated lumbar lordosis. Anterior pelvic tilt might also be secondary to weak hip extensors or weak anterior abdominals or a combination of these [13, 14]. In contrast, contracted hamstrings can pull down on the ischium resulting in a relative posterior tilt of the pelvis. In the transverse plane, the hip commonly lies internally rotated. This is more likely due to increased femoral anteversion rather than an internal rotation contracture as such [15]. In bilaterally involved children, the muscle contractures and bony deformities may not be symmetric. The more severely involved side might be more adducted and internally rotated. If this is accompanied by relative abduction and/or external rotation of the contralateral side, the deformity is categorized as “windswept” towards the abducted/externally rotated side (Fig. 17.3).
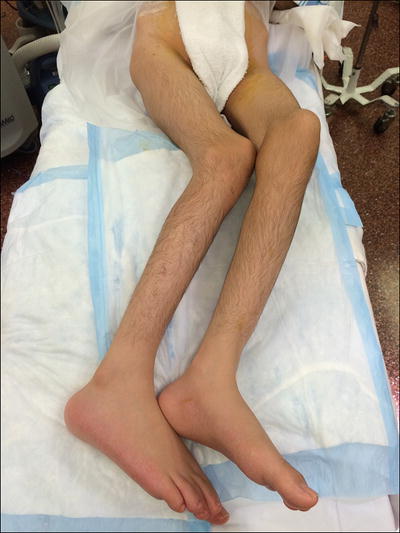
Fig. 17.3
Windswept hips
In CP, the morphology of the proximal femur is often characterized by a relative increase in the neck-shaft angle in the coronal plane (coxa valga) and excessive femoral anteversion in the transverse plane (Fig. 17.4a). Femoral anteversion is the angle in the transverse plane, by which the neck of the femur is directed (forward) from the transcondylar or coronal plane [16] (Fig. 17.4b). The terms femoral anteversion and femoral (medial) torsion are used interchangeably, the latter term suggesting that the orientation of the proximal femur relative to the coronal plane is a consequence of torsion occurring along the shaft of the femur rather than in the neck [17–19]. In the newborn, both femoral anteversion and neck-shaft angle are increased. This might be related to the fetal position. As the growing infant begins to roll over and crawl and pull up to stand and walk, the progressive extension of the hip is believed to push back the femoral neck axis to the physiologically normal values of older children and adults by the age of 1 or 2 years. The developmental delay of CP results in the retention of uncorrected higher degrees of fetal alignment [20]. This is borne out by the finding that the mean neck-shaft angle increases with the severity of CP by GMFCS level from 135.9° (range 130° to 145°) at GMFCS level I to 163.0° (range 151° to 178°) at level V [21]. Femoral anteversion also increases with GMFCS level but seems to plateau at level III to level V, where it averages 40.0° (range 25° to 67.5°). Whether the abnormal proximal femoral geometry is further affected by the abnormal forces associated with adduction/flexion contractures is less clear. The reduced femoral head coverage associated with increased femoral anteversion is compensated for by increased internal rotation of the femur and increased anterior tilt of the pelvis [15].
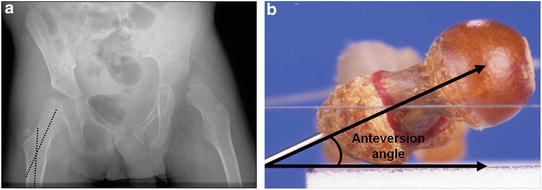
Fig. 17.4
(a) Coxa valga. (b) Femoral anteversion. Adapted with permission from Narayanan UG. Chapter 26. In: Weisel SW (editor). Pediatrics in Operative Techniques in Orthopedic Surgery. Vol. 2, Part 4. Philadelphia: Wolters Kluwer Health; 2011
At birth, the hip joint is typically normal in children with CP. Hip instability is usually acquired secondarily due to the interaction between the adduction and flexion contracture with the increased neck-shaft angle and anteversion, contributing to the (postero) lateral displacement of the femoral head. The magnitude of the hip displacement (subluxation) is quantified using the Reimer’s migration percentage (MP) [22] (Fig. 17.5). The “hip at risk” is defined as the hip that is likely to progress to hip dislocation if left untreated. This has been variously defined as the hip that reaches a migration percentage of 30–40 %. The proportion of children who will reach this threshold is directly correlated with increasing GMFCS level in three population-based studies [23–25]. Using 30 % as the definition of hip at risk, the incidence is virtually 0 % for GMFCS level I but increases to 90 % for level V [25]. If, however, a threshold of 40 % is used to define a hip at risk, the incidence is 65 % for GMFCS level V [24]. This is probably a more valid estimate of the risk because it has been observed that approximately one-third of hips with an MP between 33 and 40 % will improve spontaneously or will not deteriorate [24]. Progressive subluxation is very likely if the MP is greater than 40 % in a child still growing (Fig. 17.6a–c). The posterolaterally displaced femoral head pushes up against the superolateral surface of the acetabulum leading to increasing acetabular dysplasia. In association with hip flexion contracture, the acetabular deficiency tends to be more posterior [20]. The acetabular dysplasia is quantified by the acetabular index when the tri-radiate cartilage is open, or by the acetabular angle of Sharp when the tri-radiate cartilage is closed [26]. Initially the subluxated femoral head might reduce in the abducted position, but eventually the dislocation becomes irreducible. The uncovered femoral head is subject to the abnormal forces of the overlying capsule and abductors draped over the superolateral surface of the femoral head (Fig. 17.6d). This leads to loss of sphericity and progressive flattening of this surface leading to increasing femoral head deformity. Ultimately, there is erosion of the articular cartilage and degenerative changes consistent with osteoarthritis (Fig. 17.6e).
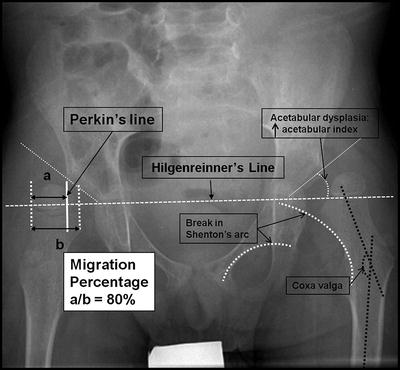
Fig. 17.5
AP radiograph of the pelvis with radiographic parameters quantifying hip displacement and acetabular dysplasia
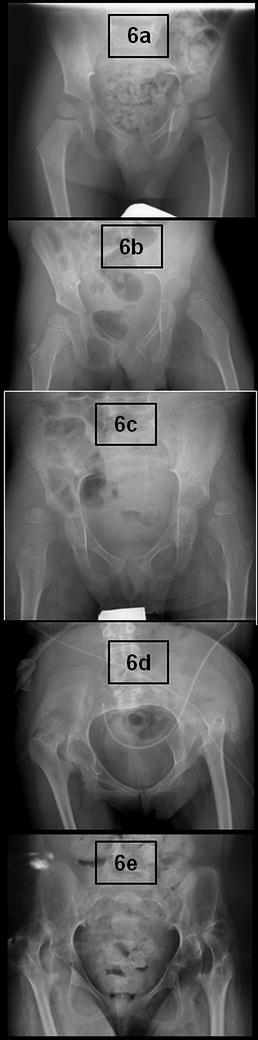
Fig. 17.6
(a–c) Hip at risk progressing to increased subluxation and acetabular dysplasia. (d, e) Dislocated femoral head leading to loss of sphericity and advanced degenerative changes
Knee and Lower Leg Segment
At the knee, the most common deformity is in the sagittal plane, knee flexion deformity. The knee flexion deformity has a wide range of etiologies. It can arise from contracture of the medial and/or lateral hamstrings. Since the medial hamstrings (semitendinosus and semimembranosus) and part of the lateral hamstrings (long head of biceps femoris) take origin on the ischium which is above the hip joint, a “functional” contracture of the hamstrings is possible even in the presence of normal or even excessive length of the hamstrings when there is an associated anterior pelvic tilt that raises the origin of these muscles [13, 14, 27]. Knee flexion deformity might also be consequence of a weak gastrocsoleus complex leading to the forward translation of the tibial shank over the foot, leading to the ground reaction force passing posterior to the knee resulting in a (external) knee flexion moment that has to be counteracted with a corresponding (internal) extension moment using the quadriceps. If the knee extensors are weak, the knee goes into progressively increased flexion. A gastrocnemius contracture can also lead to flexion deformity of the knee to take the tension off the gastrocnemius, especially if the foot is being held plantigrade in a brace. A combination of these factors can be at play. Initially a flexion deformity present during weight bearing or walking may not be associated with a fixed flexion contracture. Over time passive extension is likely to become restricted with increasing magnitude of fixed knee flexion contracture due to inadequate length of the hamstrings and/or a posterior capsular contracture. Weight bearing in the crouch position increases the joint reaction force on the patella-femoral joint which can cause anterior knee, patella alta, and avulsion fractures of the inferior pole of the patella (Fig. 17.7).
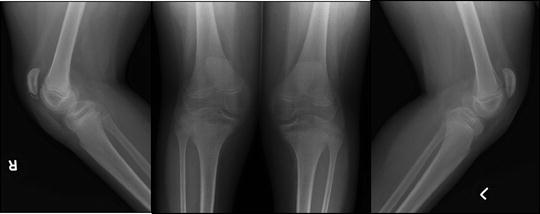
Fig. 17.7
Bilateral patella alta due to long-standing crouch with avulsion fractures of the inferior pole of patella
Box 17.1. Multiple Etiologies of Knee Flexion Deformity
1.
True contracture of the hamstring/s
2.
“Functional” contracture of hamstring/s: Increased anterior pelvic tilt due to any cause raises the origin (ischial tuberosity) of the medial hamstrings and long head of biceps, which in turn contributes to flexion at the distal insertion. Excessive anterior pelvic tilt can be due to:
(a)
Hip flexion contracture
(b)
Weak hip extensors
(c)
Weak anterior abdominal muscles
(d)
Compensatory strategy for excessive femoral anteversion
3.
Weak plantar flexion—Knee extension couple leads to forward lean of the tibia shank over the foot, leading to the ground reaction force passing posterior to the knee, causing an increased external flexion moment. Reasons for this include:
(a)
Weak gastrocsoleus complex, often iatrogenic due to over-lengthened tendo-Achilles, in a child with spastic diplegia
(b)
Hindfoot valgus with midfoot break and forefoot abduction
(c)
Excessive external tibial torsion
4.
Gastrocnemius contracture (dynamic or static): Knee bends to allow foot to be plantigrade by taking the stretch off the contracted gastrocnemius.
5.
Posterior capsular contracture of the knee joint.
6.
Combinations of above.
True coronal plane deformity at the knee is uncommon. However, the medial rotation of the hip, due to increased femoral anteversion, which is characterized by a corresponding medial rotation of the knee (patella), leads to an apparent (pseudo) valgus appearance of the (flexed) knee. This appearance is exacerbated if the femoral medial torsion or anteversion above the knee is accompanied by external tibial torsion below the knee. The tibial segment can be associated with normal torsion, and increased external or increased internal tibial torsion. At birth, the torsion of the tibia is relatively internal, with the bimalleolar axis typically being neutral in the coronal plane. The tibia undergoes external rotation maximally during the first 4 years and an average of 1 degree per year until skeletal maturity when it reaches an average of 28 degrees external [28]. In some children with CP, this internal torsion may persist due to the failure of the natural lateral rotation to occur. In others, significantly increased external tibial torsion can occur often in association with valgus hindfoot, flattening of the midfoot, and forefoot abduction. This is associated with a poor lever arm for push off in gait and is often accompanied by crouch position at the knee due to the weak plantar flexion-knee extension couple (Fig. 17.8).
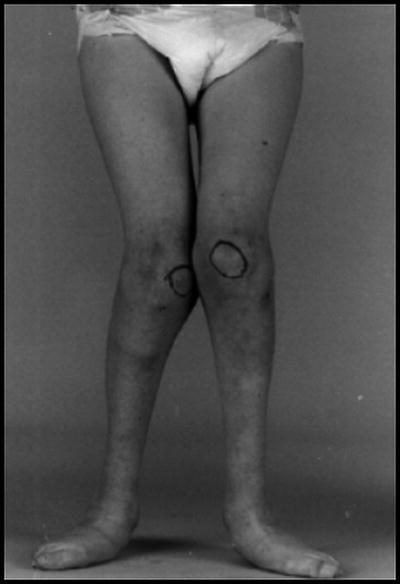
Fig. 17.8
Torsional malalignment: Pseudo-valgus due to increased femoral internal torsion and increased external tibial torsion
Ankle and Foot
Equinus of the ankle is the most common deformity of the lower extremity in CP. Equinus arises from an imbalance between the plantar flexors, which might be hypertonic and/or contracted, and the dorsiflexors due to weakness of the tibialis anterior. What begins as a dynamic contracture due to spasticity is likely to become fixed when the gastrocnemius-soleus complex fails to keep up with the tibial growth. In bilateral CP, the gastrocnemius is usually more involved than the soleus. Consequently, the ankle might have sufficient dorsiflexion when the knee is flexed but with the knee extended, the ankle equinus becomes evident due to the stretch of the gastrocnemius muscle at its origin above the knee (Fig. 17.9). In unilateral CP (hemiplegia) the soleus tends to be equally involved as the gastrocnemius, such that the equinus contracture is unchanged with the position of the knee. This distinction is important to assess as it will influence which musculotendinous structure should be targeted for intervention: gastrocnemius alone or both the gastrocnemius and the soleus or tendo-Achilles.
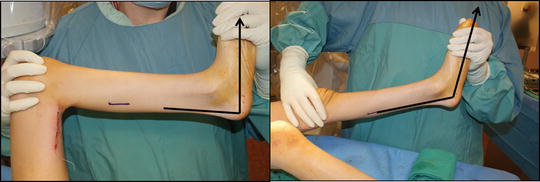
Fig. 17.9
Equinus contracture involving the gastrocnemius more than the soleus (Silfverskiold test)
In the coronal plane, the hindfoot might be in physiologic valgus position, or deformed into excessive valgus or varus. These coronal plane deformities typically arise in combination (coupled) with ankle equinus. Equinovalgus deformities are more common in bilateral CP (diplegics). With the hindfoot in valgus, the talus and calcaneus are typically plantarflexed. There is a collapse of the subtalar joint (midfoot break) with the prominence of the head of the plantarflexed talus, and the midfoot is everted taking the forefoot with it into an abducted position (Fig. 17.10a, b). There is a flattening of the longitudinal arch and a pronated appearance, although in fact the foot might be relatively supinated to compensate for the hindfoot valgus. Passive correction of the hindfoot out of the valgus position unmasks the supinated position of the foot. The abducted forefoot is often accompanied by a hallux valgus deformity that is acquired over time due to the foot rolling off the medial border during push off. To test the true dorsiflexion of the ankle, the hindfoot valgus must be passively corrected to lock the subtalar joint prior to dorsiflexing the ankle. Failing to do so allows dorsiflexion to occur about the unlocked subtalar joint and provide a spurious estimate of dorsiflexion. The equinovalgus foot with forefoot abduction provides a very ineffective lever arm to generate any power during push off (Fig. 17.11a, b). The tibial shank falls forward over the foot due to dorsiflexion through the subtalar joint, and shifts the ground reaction force behind the knee resulting in a flexed knee or crouched position.
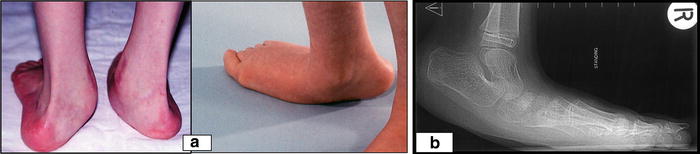
Fig. 17.10
(a) Equinoplanovalgus deformity with hindfoot valgus, midfoot break, forefoot abductus, hallux valgus. (b) Weight-bearing lateral radiograph of equinoplanovalgus deformity
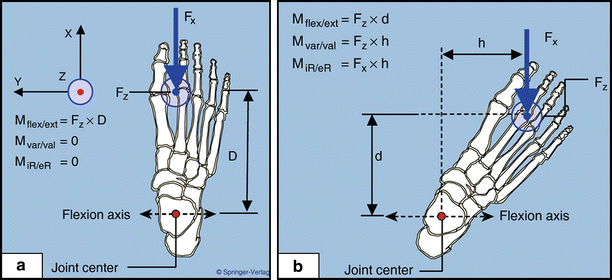
Fig. 17.11
(a) Lever arm of the normal foot. (b) Lever arm deficiency of the equinoplanovalgus and abducted foot. Figures reproduced with permission from Paley D. Gait considerations. Berlin, Heidelberg: Springer-Verlag; 2003, with kind permission from Springer Science and Business Media
Equinovarus deformities are more common in unilateral CP (hemiplegics). The hindfoot varus might be a consequence of an overactive tibialis posterior and/or tibialis anterior muscle in combination with weak peroneals. The deformity might be flexible or rigid. The hindfoot varus might be accompanied by midfoot cavus due to the tight plantar fascia or foot intrinsic muscles, and forefoot adductus. Weak tibialis anterior along with overactive peroneus longus might lead to increased plantar flexion of the first ray. This might be the primary problem resulting in a compensatory hindfoot varus to maintain a weight-bearing tripod. This can be tested using the simulated Coleman block test. In the prone position, the lateral border is pushed down on to simulate standing on the Coleman block [29]. The hindfoot varus corrects to normal valgus and the increased plantar flexion of the first metatarsal ray becomes apparent relative to the remaining rays, confirming that the hindfoot varus is secondary to the plantarflexed first ray. The hindfoot varus leads to increased weight bearing along the lateral border of the foot leading to callus formation and even stress fractures causing discomfort and difficulties with brace and shoe wear (Fig. 17.12).
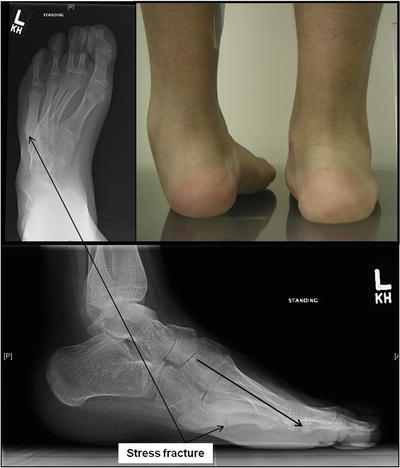
Fig. 17.12
Equinocavovarus deformity in a hemiplegic child. Note the plantarflexed first ray and the stress fracture of the 5th metatarsal due to excessive loading of the lateral border
Lower Limb Assessment in the Ambulant Child (GMFCS Levels I–III)
The physical examination of the ambulant child begins with a visual assessment of the gait to identify overall abnormal gait patterns and specific problems. This dynamic assessment is followed by an on-table examination of the lower limb tone abnormality, range of motion of the major joints, and skeletal alignment to explain the gait abnormalities identified during walking. These should in turn be linked to specific gait-related problems reported by the child during the elicitation of the history prior to the physical examination.
Box 17.2. Assessment of the Ambulatory Child
1.
Generate gait-related problem list from child and parent/s
2.
Observational assessment of the gait: Dynamic exam
(a)
With and without orthotics
(b)
With and without walking aids if possible
3.
Examination on the table: Static exam
(a)
Muscle tone
(b)
Muscle length: Passive range of motion
-
Fast stretch (R1)
-
Slow stretch (R2)
(c)
Muscle strength
(d)
Selective control
(e)
Bony alignment
4.
Radiographic imaging
5.
Video and 3-D gait analysis
Box 17.3. Five Prerequisites of Gait
1.
Stability of the weight-bearing side during stance
2.
Clearance of the foot in swing phase
3.
Appropriate pre-positioning of the swing-phase foot for the next step
4.
Adequate step length
5.
Energy conservation
Observation of Gait
Observation of GaitInspect the child’s walk from the front, back, and the side to best estimate the gait abnormalities in all three planes. The child should walk both in their usual orthotics and footwear and barefoot if possible. The assessment is both qualitative and quantitative. A useful framework to evaluate the global quality of the gait is to assess the five prerequisites or attributes of gait [30, 31]: (1) stability in stance; (2) (foot) clearance in swing; (3) correct pre-positioning of foot for the next step; (4) adequate step length; and (5) energy efficiency (minimizing the excursion of the body’s center of mass during walking). This is followed by a systematic assessment for specific abnormalities at each level: foot and ankle; knees, hips, and pelvis; and trunk and upper extremities, with due consideration of the coronal, sagittal, and transverse planes to estimate the reasons for any compromise in any five priorities of gait. This quantitative assessment can be greatly enhanced by capturing the gait with video (ideally split screen to see the frontal and sagittal planes simultaneously and in slow motion) [32].
Gait analysis provides a more sophisticated assessment of gait patterns and deviations. Three-dimensional gait analysis generated in a motion laboratory provides kinematic data that describes the dynamic position of each limb segment in each of the three planes; kinetic data which includes moments and powers about the joints; dynamic electromyography which documents the timing of specific muscle activation during the gait cycle; and pedobarographs which provide useful information about the weight-bearing characteristics of the stance-phase foot. 3-D gait analysis provides insight beyond what is derivable from observational analysis alone, and can help distinguish primary abnormalities (which might benefit from treatment) from secondary abnormalities that represent coping responses (which requires no treatment) [32–34]. Gait analysis has the potential to influence or alter treatment decisions for at least some patients [35–37]. Gait analysis has identified recognizable gait patterns which can be classified and used for making treatment decisions [38, 39]. Finally, the effectiveness of these interventions can be assessed objectively using gait analysis as a measure of gait outcomes [40].
Abnormal Gait Patterns in Cerebral Palsy
In bilateral CP, there are broadly recognizable patterns [38] (Fig. 17.13).
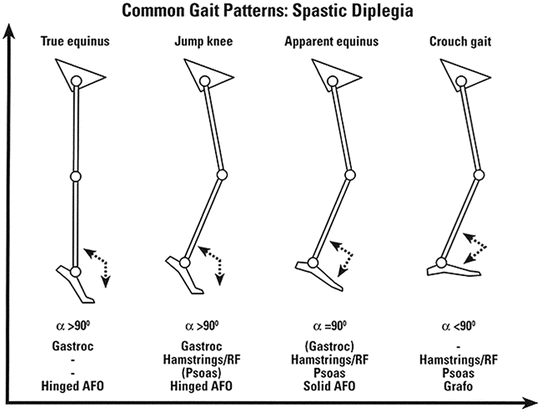
Fig. 17.13
Common gait patterns in bilateral (diplegic) cerebral palsy. Reprinted with permission from Rodda J, Graham HK. Classification of gait patterns in spastic hemiplegia and spastic diplegia: a basis for a management algorithm. Euro J Neurol 2001; 8 (Suppl 5): 98–108
True equinus pattern is characterized by the absence of a heel strike and a toe-toe pattern because the ankle remains plantarflexed throughout stance phase. If the equinus is not severe, initial contact may be with the forefoot and the heel might even come in contact with the ground in midstance. The knee can be flexed during stance phase to accommodate the equinus, or it may come to full extension or even hyperextend to allow the plantarflexed foot to remain flat on the ground for more stability (Fig. 17.14a). True equinus pattern can be associated with poor stability due to the narrow base of support and poor clearance if the knee does not flex enough to facilitate clearance of the plantarflexed foot, leading to foot drag and tripping. Step length is typically shortened because the plantarflexed foot leads to an early forefoot strike. The decreased step length might also be a consequence of poor stability in the stance-phase limb forcing the contralateral swing-phase limb to contact the ground sooner.
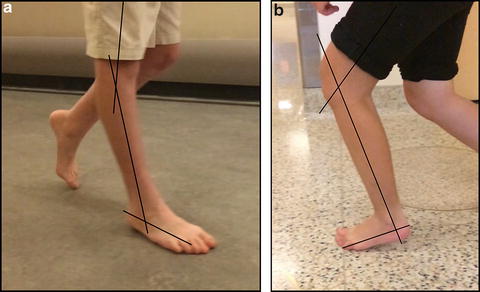
Fig. 17.14
(a) Knee hyperextended to accommodate equinus contracture with flatfoot contact despite plantarflexed position of ankle. (b) Forefoot contact (apparent equinus) to accommodate fixed flexion deformity of the knee
Jump gait pattern is characterized by toe-toe pattern due to equinus in combination with a pathologically flexed knee and flexed hip that resembles someone preparing to jump. All five priorities of gait are affected in this pattern. Step length is further compromised as the swing-phase knee might not be able to extend if increased hamstring activity prevents the knee from reaching full extension in terminal swing.
In apparent equinus pattern, the child’s heels do not come in contact with the ground even though the ankle is not in equinus (Fig. 17.14b). This happens when there is a fixed flexion deformity of the knee. If there was an adequate range of ankle dorsiflexion this fixed knee flexion deformity can be accommodated to keep the center of gravity over the base of support, which is what one finds in the crouch gait pattern. If, however, the ankle has only sufficient range to come to just neutral dorsiflexion, keeping the foot flat on the ground would cause the child’s center of gravity to fall well behind the base of support in the presence of an uncorrectable flexed knee. To stop from falling backward the body is forced to shift the center of gravity forward by flexing the hip and increasing forward trunk lean or tipping forward on tiptoe. This pattern is characterized by problems with all five priorities of gait.
Crouch gait is characterized by flexed knee during stance phase accompanied by dorsiflexed foot segment relative to tibial shank. This dorsiflexion can occur through the ankle (true calcaneus) when there is unrestricted range of dorsiflexion of the ankle, which could be iatrogenic following prior over-lengthening and weakening of the gastrocsoleus from tendo-Achilles lengthening (Fig. 17.15a). However, crouch pattern is often present even in the presence of equinus of the hindfoot, when the equinus is accompanied by increased hindfoot valgus (Fig. 17.15b). In this position, the subtalar joint collapses and “dorsiflexion” occurs through the subtalar joint accompanied by midfoot break and a rocker bottom-like foot. In either situation the tibial shank is flexed forward over the foot segment throughout stance. This shifts the ground reaction force well behind the knee, creating an external flexion moment that has to be neutralized by a corresponding internal extension moment by the activation of the quadriceps. In order to keep the center of gravity over the base of the support, the hips must flex (Fig. 17.15c). Crouch pattern seems to be the consequence of the natural history in children with more severe bilateral CP (GMFCS III or greater). All five priorities of gait are affected, and the energy consumption associated with this pattern is substantial. Weight bearing in the crouch position increases the joint reaction force on the patella-femoral joint which can cause anterior knee pain, patella alta, and avulsion fractures of the inferior pole of the patella.
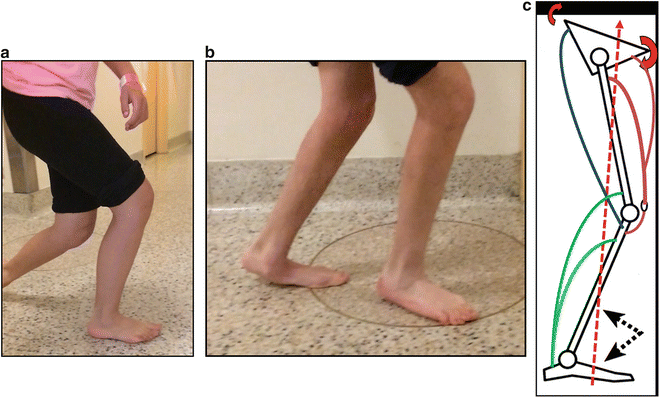
Fig. 17.15
(a) Crouch gait pattern: Flexed knee with forward translation of the tibia over the dorsiflexed ankle. (b) Crouch gait pattern in the presence of equinovalgus foot. (c) When in crouch the ground reaction force passes in front of the ankle, behind the knee and in front of the hip, resulting in ankle plantar flexion, knee extension, and hip extension (internal) moments to counteract the abnormal external moments generated by the ground reaction force
Each of the above patterns can be associated with a stiff knee pattern. Stiff knee gait is characterized by limited flexion in initial swing due to tightness of the rectus femoris, which affects clearance of the foot in swing phase. Consequently the feet might drag, slowing the gait, causing tripping and increased shoe wear.
Any of the patterns can also be associated with transverse plane abnormalities that can arise from one or more of the following levels: the forefoot (adductus or abductus), hindfoot (varus or valgus), tibial torsion (internal or external), femoral anteversion (or torsion), hip rotation (internal or external), or pelvic rotation (protraction or retraction). The foot position can be quantified by the foot progression angle (FPA), normally, an average of 5 degrees external [41]. The knee progression angle should also be assessed as this will help determine the source of rotational abnormality. A normal FPA does not rule out torsional malalignment. In children with bilateral CP it is not unusual to have increased internal rotation of the hips due to increased femoral anteversion characterized by internal knee progression angle (patella points medially) and apparent “scissoring” (Fig. 17.16). Foot progression angle may be internal, normal, or even external if accompanied by increased external tibial torsion and planovalgus abducted foot. Internal rotation of the hip in the face of external rotation of the tibial segment creates a pseudo-valgus appearance at the knee (miserable malalignment).
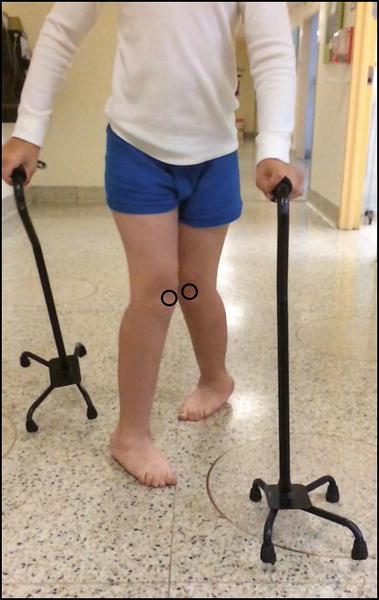
Fig. 17.16
Internal rotation gait pattern
In unilateral CP (hemiplegia) four types of gait have been described [38, 39] (Fig. 17.17). Type 1 pattern is characterized by equinus in swing phase only. This is a drop foot pattern due to weakness of the tibialis anterior which is unable to adequately dorsiflex the ankle during swing phase. Initial contact might be with the forefoot but the heel comes in contact with the ground early in stance with adequate dorsiflexion in midstance. Type 2 pattern is characterized by equinus both in swing and stance phases with a toe-toe or toe-heel pattern in stance phase. This is due to a contracture of the gastrocsoleus complex which might be dynamic due to spasticity or fixed due to static contracture. In Type 3 pattern, the knee is involved as well, showing abnormal flexion (jump) or stiff knee pattern. Finally, in Type 4 there is additional proximal involvement at the hip, including varying combinations of hip adduction, flexion, and internal rotation. This pattern can be particularly troublesome as it is often accompanied by progressive hip displacement and dysplasia.
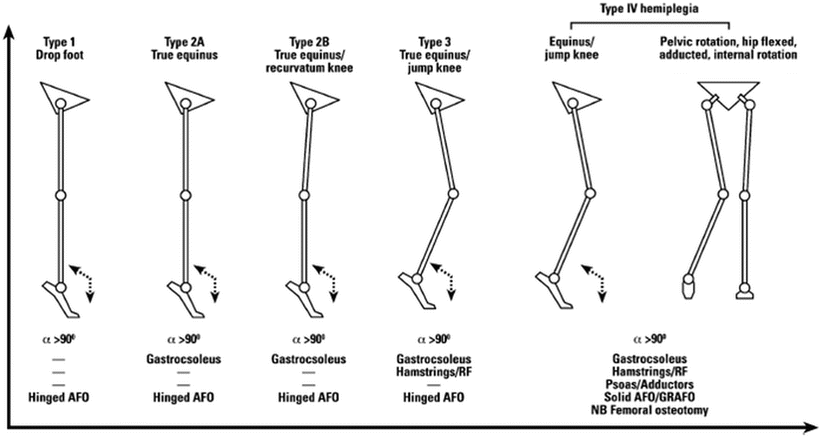
Fig. 17.17
Common gait patterns in unilateral (hemiplegic) cerebral palsy
These gait patterns are recognizable, but the distinctions are not always clear with many permutations and combinations involving one or both lower extremities.
On-Table Physical Examination
The static examination (on the table) includes assessment of muscle tone and length, bony alignment, muscle strength, and selective control.
Assessment of Muscle Tone and Length
Muscle tone tested is assessed for each muscle group by testing the relevant passive joint range of motion affected by that muscle group. Range of motion is assessed passively with a fast stretch that elicits a catch at an angle (R1) often well before the maximal passive range obtained by a slow, sustained stretch (R2) [42, 43]. This is a feature of spasticity, which is velocity-dependent hypertonia. The larger gap between the R1 and R2 represents the dynamic component of the contracture, and is more amenable to spasticity management. R2 represents the functional muscle length. AS R2 approaches R1, there is increasing static contracture or true shortening of the muscle tendon unit (in addition to tightness). Often, biarticular muscles are more involved than muscles that cross only one joint. It is therefore important to separately test the length of both groups of muscles (when possible) that contribute to range in order to intervene selectively on the biarticular muscles.
The following specific joints (muscle groups) of the lower extremity are tested in the supine position. Hip flexors are tested by extending the hip (Thomas test) while with the opposite hip is held sufficiently flexed to neutralize the anterior pelvic tilt and, but not overcorrect, the lumbar lordosis. Hip abduction is tested first with the knees flexed to test the adductor muscle length. Abduction is then tested with the hips and knees held in extension to assess the additional contribution of the gracilis which is a hip adductor and a knee flexor. The Galeazzi sign assesses the relative lengths of the thigh segment. A shorter thigh segment in combination with an adduction contracture might be indicative of hip subluxation on that side. Knee extension is quantified to rule out a knee flexion contracture. This could be due to one or more of the following: shortened hamstrings, shifted hamstrings due to increased anterior pelvic tilt, shortened proximal gastrocnemius, or a posterior contracture of the knee joint capsule. The popliteal angle tests the functional length of the hamstrings. This is first done unilaterally (one leg at a time) with the hip on the tested side held in 90° of flexion, while the contralateral hip is held in full extension. The bilateral popliteal angle or the hamstring shift test requires testing the popliteal angle with the contralateral hip and knee flexed to neutralize the pelvic tilt similar to the Thomas test [27]. This is a measure of the “true” hamstring contracture (Fig. 17.18). A large difference (reduction) in the popliteal angle (increased available hamstring length) suggests that anterior pelvic tilt might be responsible for the relative elevation of the ischium which is the origin of the medial hamstrings and the long head of the biceps femoris. Ankle dorsiflexion is tested both with the knee flexed and the knee extended (Silfverskiold test) to distinguish contractures arising from the gastrocnemius alone from those where the soleus is involved as well. At the time of testing ankle dorsiflexion, any hindfoot valgus must be corrected to neutral by supinating the foot to ensure that the subtalar joint is “locked” to ensure that dorsiflexion is occurring through the ankle joint rather than spuriously through the subtalar joint (see Fig. 17.9). Hindfoot is tested for its flexibility to correct any valgus or varus. If a cavovarus foot deformity is present, simulate a Coleman block test, as described earlier, to identify whether the hindfoot varus is fixed or not and if not confirm that it is secondary to the plantarflexed deformity of the first metatarsal ray or a primary problem [29]. In a cavovarus foot, the hindfoot might be in equinus, neutral, or even relative calcaneus with the forefoot plantarflexed relative to the hindfoot.
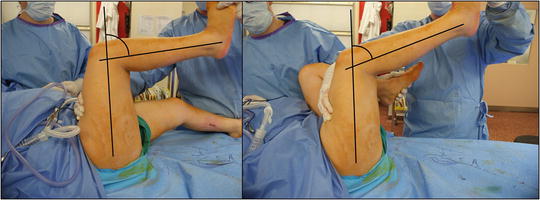
Fig. 17.18
Unilateral and bilateral popliteal angles: The hamstring shift test
In the prone position, knee flexion is tested rapidly and then slowly for the Duncan–Ely (DE) sign. A positive sign would be associated with a significant elevation of the buttock off the bed, due to an increase in hip flexion and anterior pelvic treat created when the short and/or spastic rectus femoris is being stretched and pulls down on its origin at the anterior inferior iliac spine causing the hip to flex and pelvis to tilt anteriorly.
Assessment of Bone Alignment
Assessment of the bony alignment includes the examination of the torsional profile of the lower limbs in the prone position to evaluate for excessive femoral anteversion and tibial torsion. The magnitude of femoral anteversion can be estimated using the trochanteric palpation test. In the prone position, the knee is flexed to 90° and held upright or perpendicular to the examination table. In this baseline position, the patella is pointing directly “anterior” into the table. The prominence of the greater trochanter is palpated in the proximal lateral thigh. In this baseline position, the maximum prominence of the greater trochanter sits more posteriorly. The lower leg (tibia) serves as a goniometer. The lower leg is rotated outward which corresponds to internal rotation of the hip, until the prominence of the greater trochanter is palpated most laterally, which corresponds to the femoral neck (axis) being horizontal and parallel to the floor. The number of degrees of outward rotation of the tibia relative to the vertical position at baseline represents the femoral anteversion on that side [44, 45] (Fig. 17.19). Tibial torsional alignment is based on thigh foot angle (TFA) and the transmalleolar (bimalleolar) axis (TMA). With the ankle held in neutral dorsiflexion, the TFA is the angle between the long axis of the foot (heel bisector to second toe) and the thigh axis, which is usually between 10 and 20 degrees external [41]. If the lateral border of the foot is straight, one can infer that there is no deformity arising from the forefoot and that an abnormal TFA is arising from the torsional alignment of the tibia and fibula. This is best quantified by the TMA which is the angle between the trans(bi)malleolar axis and the transverse knee axis, which is usually between 10 and 30 degrees external. TMA less than or greater than this range represent internal and external tibial torsion, respectively [46] (Fig. 17.20).
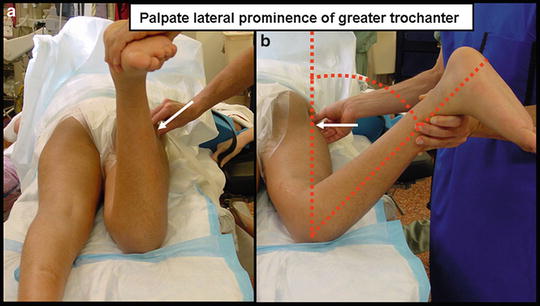
Fig. 17.19
Femoral anteversion with trochanteric palpation test
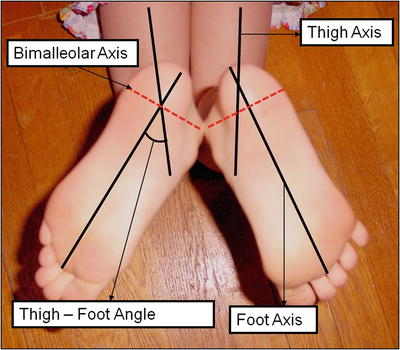
Fig. 17.20
Assessment of tibial torsional profile: The TFA and TMA
Assessment of Muscle Strength and Selective Control
In children with CP, there is increasing evidence that muscles are weak, and that motor function is directly associated with muscle strength [47]. It is therefore important, whenever possible, to assess muscle strength for prognostication and clinical decision making [48]. Muscle strength is tested for each of the lower limb muscle groups using manual muscle testing (MMT) [49]. These tests are somewhat subjective and reliant on the examiner’s experience, and are only possible in older children who are capable of cooperating with the manual testing. In the gait laboratory and in research settings, isometric muscle strength testing using dynamometers or isokinetic evaluations has some value.
Selective motor control is impaired in children with CP and contributes to ambulatory function and patterns [50, 51]. The ability to isolate movements can be graded into three levels of control: (0) no ability; (1) partial ability; and (2) normal ability to isolate movements [52]. These are tested and reported along with the strength for each muscle group tested.
Lower Limb Assessment in the Non-ambulant Child (GMFCS Levels IV–V)
In the non-ambulant child the physical examination should include an assessment of the child seated in the wheelchair or seating system, and assessment lying on the table. In the chair, specific attention is paid to the presence, flexibility, and impact of any spinal deformity in the coronal (scoliosis) and sagittal (kyphosis/lordosis) planes, and pelvic inclination on sitting balance, ischial weight bearing, skin integrity, and hygiene. Particular areas that are vulnerable to skin breakdown are over bony prominences including those due to kypho-scoliosis, sacrococcygeal area, ischial weight-bearing area, and prominences of the greater trochanter or dislocated femoral heads in the lateral proximal thigh. Additionally, in the presence of a large, rigid scoliosis there can be skin breakdown on the side of the concavity where the inferior aspect of the rib cage might rest against the iliac crest and skin crease in the flank might be hard to reach or maintain hygiene due to stiffness of the deformity.
The lower extremity examination of the non-ambulant child is done in the supine position. The examination of the hip joint, knee joint, and foot and ankle is identical to that described for ambulant children. Usually, there is little value in turning the child prone to assess the torsional profile, as these can be reasonably assessed supine.
Goals of Treatment in Cerebral Palsy by GMFCS Level
For ambulatory children (GMFCS levels I–III) the primary goals of treatment are to optimize gait efficiency in order to preserve or improve independence and physical function that will permit increased participation in real-world life activities. Functional problems of gait impact walking distance, speed, balance and stability, endurance, and fatigue, and may lead to increasing discomfort. Improving the appearance of gait and minimizing the reliance on walking aids and orthotics are also identified as important priorities by children with CP and their parents [53].
Box 17.4. Goals of Treatment: Ambulatory CP (GMFCS Levels I–III)
1.
Optimize gait efficiency (correct biomechanics) in order to optimize energy conservation
(a)
Preserve or improve physical function
-
Walk longer distance
-
Walk faster
-
Decrease fatigue
-
Better stability: Reduced tripping and fewer falls
-
Keep up with friends
(b)
Pain relief or pain prevention and increased endurance
(c)
Preserve or increase activities and participation
-
More physically active
-
More independence
-
More participation in sports/recreational activity
2.
Improve appearance of gait
(a)
Reduced reliance on walking aids
(b)
Reduced use of orthotics
(c)
Feet flat on the ground
(d)
Feet pointing forward
(e)
Reduced dragging of feet
(f)
Stand and walk taller (knees less bent)
(g)
More symmetry
Goals of treatment for nonambulatory children (GMFCS IV and V) are completely different from those for ambulatory children. These more severely involved children are at high risk of developing joint contractures, progressive hip displacement, and spinal deformity that interfere with comfort and caregiving, positioning, transfers, mobility, and seating. The musculoskeletal involvement occurs in the context of other associated comorbidities including seizure disorders, visual and hearing impairments, cognitive delays, swallowing difficulties, drooling, aspiration and pneumonias, gastroesophageal reflux, malnutrition, constipation, and incontinence. The goals of treatment are to prevent or relieve pain, facilitate caregiving, improve health, and optimize the quality of life [54].
Box 17.5. Goals of Treatment: Nonambulatory CP (GMFCS Levels IV–V)
1.
Relieve or prevent pain and discomfort
2.
Facilitate ease of care
(a)
Dressing, toileting, bathing/hygiene
(b)
Positioning: Seating and lying down
(c)
Transfers and mobility
3.
Preserve or improve health
4.
Improve quality of life
Management of Lower Extremity Problems: Principles of Treatment and Techniques
In general, the principles of treatment include (1) prevention or slowing the development of progressive joint contractures, bony deformities, and joint instability in the younger child, and (2) correction of these clinically significant bony deformities and joint instability when these have already occurred. These objectives are best achieved using a multidisciplinary approach.
Early intervention focuses on dealing with dynamic contractures in order to delay the need for more extensive surgical procedures (Fig. 17.21). Multiple strategies are used including physical therapy to stretch muscles, orthotics (braces) to maintain stretch or stabilize joints, and serial casting in an attempt to simulate the stretch that would normally arise from typical physical activity. Stretch is facilitated by local or systemic tone management by pharmacologic (botulinum toxin-A, phenol injections) or neurosurgical methods (selective dorsal rhizotomy, intrathecal baclofen). Effective tone management improves range of motion and tolerance of brace wear, and might prevent or delay the onset of static contractures and bony deformities [55–60]. Severe spasticity involving bilateral lower extremities in children with good balance and muscle strength might benefit from more extensive spasticity control from selective dorsal rhizotomy (SDR), which has been shown in randomized trials to produce significant reduction in muscle tone, but only modest benefits on physical function [61–64]. SDR does not necessarily reduce the need for subsequent orthopedic surgery [65–67]. In a systematic review, the functional effects and benefits of SDR in the long term remain uncertain, despite appearing promising in small series of selected patients [68–70]. Baclofen is a GABA agonist that can reduce hypertonia. The relatively large oral doses required for any meaningful effect can be associated with undesirable side effects. When administered intrathecally, very small doses (micrograms) are required to reduce severe generalized hypertonia due to spasticity or dystonia. Intrathecal baclofen administered continuously by an implantable pump is usually reserved for more severely involved children (GMFCS level V), as it can be associated with significant complications [71–73].

Full access? Get Clinical Tree
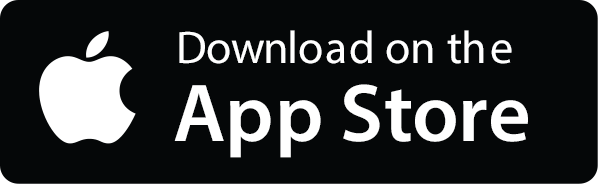
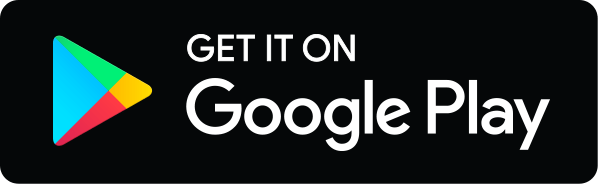