Key Points
- •
Amniocentesis is used from 15 weeks of gestation onwards for prenatal diagnosis of chromosomal abnormalities, single-gene disorders, fetal lung maturity, fetal infections and inflammation.
- •
Chorionic villus sampling (CVS) is used from 10 weeks of gestation onwards for prenatal diagnosis of single-gene defects and chromosomal abnormalities.
- •
Early amniocentesis (<15 weeks) and early CVS (<10 weeks) have been proscribed because of increased risk for fetal loss rate and structural abnormalities
- •
The procedure-related fetal loss rates associated with amniocentesis and first trimester CVS performed by trained specialists at appropriate gestations appear to be low (∼0.1% and 0.2%, respectively).
- •
In equally experienced hands, both the transcervical and the transabdominal routes for CVS have a similar fetal loss rate.
- •
The indications for diagnostic fetal blood sampling and other invasive procedures are now limited because of the availability of less invasive or noninvasive methods.
Introduction
Chorionic villus sampling (CVS), amniocentesis and to a lesser extent fetal blood sampling are the most common invasive diagnostic procedures performed prenatally. Amniocentesis was first introduced in the early 1880s as a therapeutic procedure to treat polyhydramnios. The technique evolved over the years to be used for the assessment of fetal well-being, including monitoring of Rh-alloimmunised pregnancies. The use of amniocentesis for exclusively genetic indications began in the mid-1950s from early work on fetal sex determination by X-chromatin analysis of amniotic fluid cells (AFCs), to several reports of successful diagnosis of a wide variety of chromosomal and metabolic disorders over the next few years. CVS was introduced and has been used solely for genetic diagnosis and remains the invasive procedure of choice in the first trimester. Fetal blood sampling is used less frequently for genetic diagnosis (generally after 18 weeks), but its main role is in confirming the diagnosis fetal anaemia.
This chapter addresses current technique and the safety of genetic amniocentesis, CVS and fetal blood sampling. Indications and methods of prenatal diagnosis are considered in detail throughout this text. In addition, a brief consideration of the impact of noninvasive prenatal testing on the role of these diagnostic procedures is included.
Whenever possible, preconception counselling to discuss genetic risks and available antenatal testing options before pregnancy should be made available to every couple. When an indication for an invasive diagnostic test has been identified, the couple must be informed about the risks associated with such procedures, the accuracy and limitations of prenatal diagnosis, the time required before results become available, technical problems potentially necessitating a repeat procedure, and the rare possibility of an inability to make a diagnosis.
Amniocentesis
Amniocentesis should be performed only by a trained obstetrician who has acquired adequate skill and experience in this procedure, has the availability of high-quality ultrasonography and has access to a laboratory with experience in performing prenatal diagnostic tests. The recommendation that the procedure should be performed by an obstetrician is not because of technical difficulty but because the operator must always be prepared to deal with the potential complications of the procedure. According to the American College of Obstetricians and Gynecologists (ACOG), if a serious abnormality is detected and the couple elects to terminate the pregnancy, the obstetrician must either perform the abortion or refer the family to a provider who will act on their request.
Amniocentesis can be performed from about the 15th week of gestation, when the ratio of viable to nonviable cells is greatest. Early amniocentesis (EA) performed before 14 weeks’ gestation, and transvaginal amniocentesis is only of historical interest because of the technical difficulty as well as associated risks of infection and spontaneous abortion.
Technique of Amniocentesis
After a thorough ultrasonographic examination, a needle insertion site is selected. Under continuous real-time ultrasound guidance, the needle is inserted to the optimal pocket of amniotic fluid (AF) while avoiding the fetus. Avoiding needle insertion through the placenta is desirable but not mandatory. Although Tabor and coworkers reported that transplacental needle insertion increased the risk for the procedure, this has not been confirmed by others.
Local anaesthetic is typically not needed. It is uncertain if the site of needle placement affects the level of pain. Counselling before amniocentesis should emphasise that the actual pain and anxiety experienced during the procedure are significantly lower than expected.
The maternal skin is cleansed with an iodine- or alcohol-based solution; sterile drapes are then placed around the needle insertion site to help maintain an aseptic field. A disposable 22-gauge spinal needle with stylet is most frequently used. During the entire procedure, the needle tip should be continuously visualised using two-dimensional real-time ultrasound monitoring. In view of the extensive anterior placenta, this procedure was performed transplacentally. Use of four-dimensional ultrasound guidance has been suggested, but there are no objective data to indicate improved outcomes ( Fig. 23.1 and ).

After confirming that the needle is in its proper location, the stylet is removed, and a 10- or 20-cc syringe is attached to the hub of the needle. The initial 1- to 2-mL sample is usually discarded. Ten to 20 mL of AF is usually aspirated into sterile disposable plastic syringes, although as little as 3 to 5 mL of AF has been shown to suffice for reliable prenatal cytogenetic results. Maternal cell contamination appears to occur more frequently in genetic amniocentesis samples that are obtained by physicians who perform fewer than 50 genetic amniocentesis annually. Investigators have described using a vacuum container aspiration technique for amniocentesis, but there appears to be little, if any, advantage over using a syringe technique.
Amniocentesis had been reported to be unsuccessful with rates as high as 5.9% to 10.6%, especially in the era when concurrent ultrasound guidance was not the norm. Because real-time ultrasound guidance has become routine, failure to obtain AF occurs far less. However, this is much more problematic with EA. When performed at 15 to 16 weeks of gestation by experienced practitioners, failure to obtain AF should occur in fewer than 1% of cases.
Training in performing amniocentesis has traditionally been by trainees observing experienced operators followed by the trainees performing the procedure under direct supervision of the mentors. Modern high-fidelity simulator-based models might be useful for teaching amniocentesis because trainees’ performance has been shown to improve with experience on the simulator.
Amniocentesis in Twins and Higher-Order Multiple Pregnancies
Amniocentesis can be performed successfully in most twin pregnancies perhaps with no increased risk compared with singleton gestations undergoing amniocentesis, although the risk is difficult to quantify accurately because of the lack of randomised studies.
Separate amniocentesis of each sac is used in most centres in the United States to assess individual fetuses irrespective of the chorionicity. Each amniotic sac may be identified if the clinician injects a dye (indigo carmine) immediately after aspiration of the first AF sample but before withdrawal of the needle. After completion of the first amniocentesis, a second amniocentesis is performed in the ultrasonographically located area of the other fetus. Aspiration of clear AF indicates that the second sac was successfully entered; aspiration of blue-tinged AF indicates that the original sac was reentered. However, this is not necessary to perform routinely because visualisation of the membranes separating the sacs is generally possible. Methylene blue dye is proscribed because it has been associated with high risk for small intestine atresia and fetal death.
Single-needle insertion under ultrasound guidance to sample both sacs in twins has been reported. The main concern is that the single-puncture technique could lead to cross-contamination between sacs, resulting in diagnostic inaccuracy. The technique described by Jeanty and colleagues uses a single myometrial needle puncture into the first amniotic sac and then through the membranous septum into the second sac. This technique has been validated by Sebire and coworkers, with no increase in cell contamination between twin fetuses or increased risk for pregnancy loss.
Using the above techniques, experienced investigators have been successful in obtaining information regarding both fetuses in more than 90% to 95%. The reported loss rates after amniocentesis in twins varies from 0.6% to 2.7%.
Amniocentesis has been performed in several triplet pregnancies, with successful aspiration of fluid from all gestational sacs. Still, data are insufficient to make any statement regarding risks of amniocentesis in triplet gestations.
Maternal Risks of Amniocentesis
Life-threatening maternal risks are extremely rare. Amnionitis occurs in approximately 1 per 1000 women who undergo amniocentesis. This may lead to fetal loss but is seldom life threatening to the mother.
Minor maternal problems, however, are not rare. Approximately 2% to 3% of women experience transient vaginal spotting or leakage of AF after amniocentesis. Although almost always limited in amount and duration, AF leakage could persist and lead to oligohydramnios and pregnancy loss. Oligohydramnios is a well-known cause of fetal deformation and pulmonary hypoplasia.
Uterine contractions or cramping immediately after amniocentesis are not rare. Again, expectant management and reassurance are generally all that is required.
Fetal Risks of Amniocentesis
Potential fetal risks include spontaneous abortion, injuries caused by needle puncture, placental separation, chorioamnionitis, premature labour and injury caused by the withdrawal of AF (e.g., amniotic bands). Rare but reported direct needle injuries include ileocutaneous fistula, peritoneoparietal fistula, gangrene of an arm, ocular trauma, ileal atresia, porencephalic cysts, patellar disruption, brain injuries, peripheral nerve injury and umbilical cord haematoma. Some of these problems are more logically attributed to amniocentesis than others, and all except a few of these case reports are from the era before concurrent use of real-time ultrasound guidance.
HIV Transmission After Amniocentesis
Amniocentesis has been associated with an increase in the rate of vertical transmission of human immunodeficiency virus (HIV) type 1. However, with the advent of retroviral chemoprophylaxis, the risk for transmission as a result of amniocentesis has been markedly reduced. Bucceri and coworkers reported nine HIV-infected women who underwent amniocentesis between 16 and 20 weeks of gestation. Six of these women were on chemoprophylaxis, and none of 10 infants born to these women were infected. The International Perinatal HIV Group reported that five of nine HIV-infected women not on chemoprophylaxis undergoing amniocentesis delivered infected infants, but none of five infants born to women taking zidovudine were infected. Recently, a 4-year report from an Italian registry including selected HIV-infected pregnant women receiving combined antiretroviral prophylaxis found no cases of vertical transmission after amniocentesis or CVS. It appears from this limited evidence that HIV-infected women electing to have amniocentesis may benefit from chemoprophylaxis.
Pregnancy Losses After Amniocentesis
Although most spontaneous abortions occur during the first trimester, these may also occur during the second trimester. Moreover, older women are relatively more likely to have a spontaneous abortion than are younger women. Age-related phenomena could possibly influence frequencies of premature delivery and other adverse pregnancy outcomes. Thus the only reports to which any real weight can be attached are those in which participants undergoing amniocentesis are matched with control participants not undergoing the procedure, after which the excess fetal loss in the subject group may thus be assessed. Four major national collaborative studies (US, UK, Canadian and Danish studies) of the risks of amniocentesis have been published.
The UK study reported a higher loss rate, but the participants were significantly older than the control participants, and age alone might account for some of the increased fetal losses and antepartum haemorrhage. Indeed, in comparison with the US and Canadian studies, the British study showed an apparent deficit of fetal loss among the control participants rather than excess among the participants. Longitudinal studies in the United Kingdom and North America of ultrasonographically monitored pregnancies revealed surprisingly few losses in pregnancies that were viable at 8 to 16 weeks.
Tabor and colleagues published results of the only randomised controlled study in 1986 of amniocentesis performed on 4606 women aged 25 to 34 years who were at low risk for fetal genetic abnormalities. Amniocentesis was performed under real-time ultrasound guidance with an 18-gauge needle. Thus this was the first collaborative study of amniocentesis safety that routinely required ultrasound. The spontaneous abortion rate after 16 weeks was 1.7% in patients who had undergone amniocentesis compared with 0.7% in control participants ( P < .01), with a 2.6-fold relative risk for spontaneously aborting if the placenta was traversed.
Tongsong and coworkers reported a large-scale cohort study from Thailand in which singleton pregnant women between 15 and 24 weeks of gestation undergoing amniocentesis were matched prospectively to control participants on a one-to-one basis for maternal age, parity and socioeconomic status. A total of 2256 pairs were recruited. There were no significant differences in fetal loss rates, premature deliveries or placental abruptions between the two groups ( P > 0.5).
Papantoniou and colleagues reported a retrospective analysis of 1006 women undergoing amniocentesis with singleton pregnancies. Control participants consisted of 4024 women undergoing amniocentesis and who had no risk factors. In both groups, amniocentesis was performed between 16 and 18 weeks of gestation. When cases and control participants were stratified according to maternal age, a statistically significant difference in the fetal loss rate was observed between women aged 20 to 34 years (2.54%) and women older than 40 years (5.1%). Women with a history of vaginal bleeding during the current pregnancy also had a higher fetal loss rate (6.5%) compared with control participants (2.8%). Women with a history of previous spontaneous abortions or terminations had a fetal loss rate of 8% compared with a 2.8% loss rate among control participants.
In 2006, Eddleman and coworkers reported the procedure-related fetal loss rate after midtrimester amniocentesis using the database from the National Institute of Child Health and Human Development-sponsored multicentre first and second trimester evaluation of risk (FASTER) trial designed to compare first trimester Down syndrome screening to second trimester screening. Among a total of 35,003 patients who were enrolled in the FASTER trial, 3096 underwent midtrimester amniocentesis (study group), and 31,907 did not (control group). The rate of fetal loss before 24 weeks’ gestation was compared between the two groups, and multiple logistic regression analysis was used to adjust for potential confounders. The spontaneous fetal loss rates were 1.0% in the amniocentesis group and 0.94% in the no amniocentesis group. The difference between these groups was not significant ( P = .74; 95% confidence interval (CI) –0.26% to 0.49%). Several studies have subsequently reported lower loss rates, thereby validating the findings of the FASTER trial group.
Overall, we can conclude that the conventionally stated pregnancy loss rate of 0.5% is no longer appropriate in experienced hands. Surely, it is illogical to counsel the same 0.5% risk offered a quarter century ago when ultrasound was not available. In support, Armstrong and coworkers followed the outcome after 28,613 procedures performed by obstetricians throughout the United States, mostly for advanced maternal age. The total loss rate (combined background plus procedure related) was only 1 in 362. In comparing 11,746 women undergoing genetic midtrimester amniocentesis and 39,811 women who did not have invasive procedures over a 16-year period, Odibo and coworkers concluded that the fetal loss rate attributable to amniocentesis was 0.13%, or 1 in 769.
Recently, Akolekar et al performed a systematic review of studies published after 2000 that had included at least 1000 procedures and had a control group and reported a pooled risk for fetal loss of 0.11% (95% CI, –0.04% to 0.26%) after amniocentesis before 24 weeks. When limited to studies published over the past 10 years, the procedure-related loss rate after amniocentesis is 0.16% (95% CI, –0.57% to 0.51%). See Table 23.1 .
Study (Year) | Amniocentesis Loss Rate (% (95% CI)) | Control Group Loss Rate (% (95% CI)) | Procedure-Related Loss Rate (% (95% CI)) |
---|---|---|---|
Eddleman et al. (2006) | 1.00 (0.68–1.42) | 0.94 (0.84–1.05) | 0.06 (–0.30–0.42) |
Caughey et al. (2006) | 0.83 (0.73–0.94) | — | — |
Towner et al. (2007) | 0.46 (0.36–0.58) | 0.53 (0.52–0.65) | −0.07(–0.22–0.09) |
Odibo et al. (2008) | 0.97 (0.80–1.16) | 0.85 (0.76–0.94) | 0.12 (–0.07–0.31) |
Tabor et al. (2009) | 1.39 (1.27–1.52) | 0.90 (0.88–0.92) | 0.49 (0.39–0.60) |
Pitukkijronnakorn et al. (2011) | 0.37 (0.18–0.66) | 0.20 (0.04–0.59) | 0.17 (–0.18–0.51) |
Corrado et al. (2012) | 1.00 (0.68–1.43) | 0.82 (0.22–2.09) | 0.18 (–0.77–1.13) |
Pooled loss rate | 0.86 (0.67–1.10) | 0.70 (0.54–1.04) | 0.16 (-0.57–0.51) |
Early Amniocentesis
Early amniocentesis was explored to obviate the inconvenience for patients of having to be rescheduled if they presented for CVS but were determined to be beyond 14 weeks of gestation but earlier than 15 weeks of gestation.
The technique for EA is essentially the same as for traditional amniocentesis, except that a smaller volume of AF is withdrawn. One limitation of EA was a higher prevalence of tenting of the membrane and dry tap. The earlier in gestation one attempts amniocentesis, the more problematic membrane tenting becomes, given incomplete fusion of the chorion and the amnion. Tenting of the membranes is seen in about 10% of EA procedures.
The use of EA gained popularity in the late 1980s, but several studies over the next decade highlighted the complications associated with the procedure.
Based on the data showing that EA results in significantly higher rates of pregnancy loss and complications than performing traditional amniocentesis, the ACOG has recommended that EA (<14 weeks’ gestation) should not be performed.
Third Trimester Amniocentesis
Amniocentesis in the third trimester of pregnancy has been mostly performed to document fetal lung maturity. It can also be indicated for fetal anomalies detected after the typical second trimester screening window, and these tend to pose little or no risk for fetal loss. The technique is similar to that used for diagnostic amniocentesis in the second-trimester. The challenge is with finding an adequate pocket of AF that is free from umbilical cord or fetal parts to tap. Reports of neonatal complications even in the presence of documented positive test results for fetal lung maturity has limited the role of amniocentesis for this indication.
Third trimester amniocentesis has also been reported in small series to be useful for the diagnosis of inherited bleeding disorders before delivery. Bleeding disorders such as moderate to severe haemophilia A and B and type 3 von Willebrand disease can confer an increased risk for bleeding during delivery. For affected cases, restrictive birth plans are implemented, and unaffected cases are allowed routine obstetric management. Because these are still based on small case series, larger studies are needed to confirm the safety and reliability of this approach.
Chorionic Villus Sampling
Chorionic villus sampling was formally introduced in the 1980s and has become established as the prenatal diagnostic procedure in the first trimester after an early feasibility report in 1968 by Mohr. The indications for CVS are similar to those discussed for amniocentesis. CVS also offers the advantage of early and rapid diagnosis for pregnancies at high-risk (e.g., 25%–50% risk) for certain genetic disorders. In these scenarios, rapid DNA testing can be performed on uncultured DNA cells and the couple offered the option of early pregnancy termination if affected.
Technique
Chorionic villus sampling is typically performed between 10 and 14 weeks’ gestation. However, CVS can be performed at much later gestational ages if amniocentesis or other testing is not possible (e.g., with oligohydramnios). The drawback with late CVS is a higher rate of culture failure of the villi. Reports of possible association between CVS performed earlier than 10 weeks and fetal limb constriction and other anomalies led to proscription of the procedure before this gestational age.
Chorionic villus sampling can be performed through transabdominal and transcervical routes ( Figs. 23.2 and 23.3 and ). There is no evidence that one route is safer or more reliable than the other. Operator preference and position of the placenta are the most influential factors regarding the route chosen for CVS. In a high anterior or fundal location of the placenta, a transabdominal route is preferred; in a posterior location, a transcervical route is optimal.


In the transabdominal technique, the ideal site exposing the longest axis of the placenta is identified under ultrasound guidance. The skin is disinfected with iodine- or alcohol-based solution, and ideally, a local anaesthetic is given (when a needle larger than 20 gauge is used). We prefer the double-needle technique. This involves using an 18-gauge needle as a trocar through which a smaller gauge needle (20 or 21 gauge) is inserted into the placenta. A 20-cc syringe containing Roswell Park collection medium mixed with a small concentration of heparin is attached to the end of the needle, and a negative pressure is created. The needle is moved up and down through the placenta several times while maintaining the negative pressure. On removal, the sample is emptied unto a petri dish and examined for the presence of a sufficient amount of chorionic villi. With the double-needle technique, multiple passes at the placenta can be made without reinsertion through the uterine wall. Some operators, however, report good results using a single-needle technique.
For the transcervical route, the patient is placed in a lithotomy position. Then a sterile speculum is introduced to expose and cleanse the cervix with iodine solution. In our centre, we generally do not use a tenaculum to steady the cervix, but in rare situations, this may be needed. Under ultrasound guidance, a 16-gauge catheter with a malleable guidewire is inserted in the region of the trophoblast. The guidewire is then removed, a 20-cc syringe containing heparinised medium is attached to the end of the catheter and a negative pressure created. The catheter is withdrawn slowly and the sample transferred to a petri dish and examined for adequacy of villi concentration. Transcervical CVS can also be performed using especially designed small biopsy forceps. This technique may be associated with less pain and less failure to obtain adequate sample.
Complications of Chorionic Villus Sampling
Overall, CVS is considered safe, but as with most invasive procedures, there is a risk for potential complications. Vaginal bleeding is rare with transabdominal CVS but may occur in 7% to 10% of cases of transcervical CVS. Other complications of CVS include chorioamnionitis (incidence <1 per 1000 cases), acute rupture of membranes, oligohydramnios (0.3%), preterm rupture of membranes and preterm labour. Previous suggestions associating CVS with hypertensive disorders of pregnancy have not been confirmed by more recent studies.
In experienced hands, the procedure-related loss rate after CVS is low. It was reported to be 0.22% (95% CI, –0.71% to 1.16%) by a recent systematic review and similar to that for amniocentesis. The Canadian Collaborative experience showed no significant difference in loss rates: 7.6% in the amniocentesis group compared with 7% in the CVS group. Similarly, the US collaborative group reported no significant difference in loss rates between amniocentesis and CVS. In contrast to these studies, the Medical Research Council (MRC) working party on the evaluation of CVS reported a 4.6% higher loss rate after CVS compared with amniocentesis. The most commonly reported rates of loss from the time of CVS up to 28 weeks’ gestation is between 2% and 3%, but a recent report suggests that the rate may be much lower. It is also important to consider the higher background pregnancy loss rate in the first trimester that may not be related to the procedure. Selected studies on loss rates from CVS are summarised on Table 23.2 . The overall loss rate from the systematic review by Akolekar and associates is 0.22 (95% CI, –0.71 to 1.16). These studies have the common limitation that they are not randomised.
Study (Year) | CVS Loss Rate (% (95% CI)) | Control Group Loss Rate (% (95% CI)) | Procedure-Related Loss Rate (% (95% CI)) |
---|---|---|---|
Lau et al. (2005) | 1.85 (1.20–2.71) | 1.16 (0.62–1.97) | 0.69 (–0.29–1.67) |
Odibo et al. (2008) | 2.68 (2.26–3.16) | 3.35 (2.86–3.90) | −0.67(–1.35–0.01) |
Akolekar et al. (2011) | 1.84 (1.34–2.46) | 1.14 (1.03–1.27) | 0.69 (0.24–1.15) |
Pooled loss rate | 2.18 (1.61–2.82) | 1.79 (0.61–3.58) | 0.22 (–0.71–1.16) |
The CVS loss rate does not appear to be significantly affected by the route of the procedure. Table 23.3 summarises four studies comparing transabdominal to transcervical CVS. When all studies are pooled, the loss rate is not significantly different irrespective of route.
Study (Year) | TC-CVS Loss Rate (%) | TA-CVS Loss Rate (%) | Relative Risk (95% CI) |
---|---|---|---|
Bovicelli et al. (1986) | 3.3 | 3.3 | 1.0 (0.15–6.87) |
Brambati et al. (1991) | 7.9 | 7.4 | 1.07 (0.72–1.58) |
Smidt-Jensen et al. (1992) | 8.2 | 3.0 | 2.72 (1.82–4.07) |
Pooled loss rate | 7.9 | 4.6 | 1.72 (0.79–3.58) |
Safety of Chorionic Villus Sampling in Multiple Pregnancies
Chorionic villus sampling can be performed successfully in multiple gestations by experienced operators. Wapner and associates reported a 6-year experience with the successful performance of CVS on 81 set of twins with an overall pregnancy loss rate of 3.2% before 28 weeks’ gestation. Other groups have reported their experience with similar loss rates. The technique is similar to that used for singletons, and both transabdominal and transcervical approaches are safe. Sometimes a combination of both approaches may be used depending on the location of the placentas. With monochorionic twins, many operators would sample only one fetus, and although a heterokaryotypic genotype is possible, this is so rare and does not warrant routine sampling of both. With dichorionic twins, both fetuses must be sampled, and the increased risks quoted earlier may apply more to this type of placentation. A recent systematic review of loss rates from CVS in multiple pregnancies found no randomised trial to evaluate and from a summary of pooled studies, a loss rate of 2.75% (95% CI, 1.28–4.75) before 20 weeks’ gestation and 3.44% (95% CI, 1.67–5.81) before 28 weeks’ gestation.
Laboratory Aspects of Chorionic Villus Sampling
Chorionic villi have three major components: syncytiotrophoblasts, cytotrophoblasts and an inner mesodermal layer that contains fetal capillaries. These components arise from multiple sources with the potential to yield confounding results. These issues were problematic in the early period of the CVS technique. The US collaborative study reported only a 1.1% incidence of needing another confirmatory test with the most common indications being laboratory failure, maternal cell contamination and confined placental mosaicism (CPM). This has become a rare occurrence because of improved laboratory techniques. CPM occurs when there is a discrepancy in the cytogenetic material between the placenta and the fetus with a resultant abnormality confined to the placenta. There is potential for this to occur because only few of the cells constituting the inner cell mass in early embryonic period eventually become part of the fetus. The rest develop into extraembryonic tissues with potential for trisomies confined to these tissues. The mosaicism tends to be confined within the trophoblast because of two mechanisms, postzygotic nondisjunction within the placenta or trisomic rescue in the fetus.
Confined placental mosaicism occurs in 1.3% of CVS procedures. Although follow-up procedures such as amniocentesis or fetal blood sampling may be needed to confirm the diagnosis, CPM may also be a marker for a pregnancy that needs closer follow-up for risk for intrauterine growth restriction, perinatal death or uniparental disomy.
Fetal Limb-Reduction Abnormalities and Chorionic Villus Sampling
The possibility of an association between CVS and limb-reduction defects has been the subject of many reports. Following the first report by Firth and colleagues of four infants with oromandibular-limb hypogenesis and one with terminal transverse limb reduction defect after CVS, similar case series were reported by other groups. These reports also indicated that the complication appears confined to CVS procedures performed before 70 days of gestation. However, a large World Health Organisation registry of more than 200,000 CVS procedures found no significant association between the procedure and limb-reduction defects. Given the controversy regarding this association, it is prudent to inform women requesting CVS of these reports and that if such a risk exists, it is less than 1 in 3000 procedures and not reported for procedures performed after 70 days’ gestation.
Fetal Blood Sampling
Fetal blood sampling is most often used for rapid fetal karyotyping, evaluation of fetal haematologic disorders, identification of fetal infection (by culture or molecular typing), drug therapy and the diagnosis and treatment of fetal anaemia by transfusion.
When fetal blood is withdrawn from the umbilical cord, the procedure may be referred to in several ways, as fetal blood sampling, percutaneous umbilical blood sampling (PUBS), funicentesis or cordocentesis.
Percutaneous umbilical blood sampling for fetal blood chromosome analysis has been used to help clarify purported chromosomal mosaicism detected in cultured AF cells, chorionic villi or both. Rapid assessment of fetal chromosome complement has been accomplished by “direct” cytogenetic analysis of noncultivated nucleated blood cells. In addition, some fetal abnormalities do not become apparent until later in pregnancy, and in such instances, rapid results may prove useful for decision making with regard to obstetric management and mode of delivery.
Indications for Fetal Blood Sampling
Fetal blood sampling was once used for the prenatal evaluation of many fetal haematologic and biochemical abnormalities. Fetal haematocrit can be directly measured to assess fetal haemolysis resulting from Rh or other antigen incompatibility and alloimmunisation states. Fetal haemoglobin can be directly evaluated to diagnose sickle cell disease, α- or β-thalassemias or other haemoglobinopathies. However, these disorders can now also be correctly identified by using DNA analysis of chorionic villi or AF cells. Fetal blood sampling can also be used to assess platelet quantity and quality of function.
Fetal blood has also been used for the diagnosis of various coagulation factor abnormalities in fetuses, such as haemophilia A, haemophilia B and von Willebrand disease. In addition to haematologic studies, fetal blood samples have been used to diagnose autosomal recessive or X-linked immunologic deficiencies, including severe combined immunodeficiency (SCID), Chédiak-Higashi syndrome, Wiskott-Aldrich syndrome and chronic granulomatous disease.
Recovery of fetal blood permits assessment of viral, bacterial and parasitic infections of the fetus. Serum fetal blood titres permit quantification of antibody titres. In addition to antibody titres, PUBS can be used for direct analysis of viral, bacterial and parasitic infections by culture of or molecular amplification of vector-specific DNA sequences in fetal blood.
Technique of Fetal Blood Sampling
Fetal blood sampling is typically performed under continuous real-time ultrasound guidance from 18 weeks of gestation onward. However, successful procedures have been reported as early as 12 weeks of gestation. Maternal sedation is usually unnecessary, but when a prolonged procedure is anticipated (e.g., with fetal blood transfusion), injection of 1% lidocaine to the local site may be of benefit, and occasionally, fetal neuromuscular blockade with pancuronium or atracurium can be considered to abolish fetal movements temporarily.
A sterile field is established by cleansing the skin with an iodine-based solution or alcohol and sterile drapes are applied. Most commonly, two-dimensional ultrasonographic needle guidance is used. Although some have suggested using four-dimensional needle guidance, there is no evidence that this newer technology is an improvement over two-dimensional visualisation.
There are several potential sampling sites. Because of its fixed position, the umbilical cord insertion site to the placenta is usually the site of choice whenever it is clearly visible and accessible. Other possibilities are a free loop of the umbilical cord or the fetal intrahepatic umbilical vein ( Figs. 23.4 and 23.5 ). Many practitioners prefer using a 22-gauge, acute-angle echo-enhanced needle, but others have advocated even smaller gauged needles (e.g., 25 gauge). The amount of blood aspirated for diagnosis depends on the indication for diagnosis by PUBS but rarely exceeds 5 cc.


On completion of the fetal blood sampling procedure, the needle is withdrawn, and an ultrasound examination is performed to evaluate fetal condition. All women at risk for Rh isoimmunisation should receive 300 mg of Rh immunoglobulin (RhIG) after the procedure.
Safety of Fetal Blood Sampling
Maternal complications from PUBS are rare but include amnionitis and transplacental haemorrhage. Data from large perinatal centres estimate the fetal risks of death in utero or subsequent spontaneous abortion to be 3% or less after PUBS. Studies directly comparing loss rates in control and treated groups have been published, but none of these are randomised. The only case control study is by Tongsong and associates, who followed 1281 women undergoing freehand cordocentesis between 16 and 24 weeks’ gestation. Women with no overt fetal anomalies (and thus not requiring a procedure) served as control participants. Indications for PUBS were increased risk for thalassemia (61%), rapid karyotyping (21%) or both (8.7%). Loss rates were 3.2% (PUBS group) versus 1.8% (control participants) with no differences in obstetric complications.
These studies have a common confounding that baseline loss rates for patients undergoing PUBS or fetal blood sampling vary greatly with the indication of the procedure. Loss rates are far greater for fetuses with ultrasound-detected anomalies than for fetuses evaluated for haemolytic diseases secondary to maternal blood group sensitisation for late booking or for clarification of mosaicism at amniocentesis. Overall, procedure-related loss rates of 1% to 1.5 %, should be assumed.
The relationship between fetomaternal transfusion and pregnancy outcome was studied. Despite a positive correlation between fetomaternal haemorrhage and bleeding time, there was no association between the degree of fetomaternal transfusion and pregnancy outcome. Others also showed that PUBS is frequently associated with fetomaternal haemorrhage, which in turn was correlated with anterior position of the placenta, duration of the procedure and number of needle insertions.
Finally, in a retrospective analysis of 59 PUBS procedures performed in 30 multiple pregnancies (29 twins and 1 triplet) at a gestational age of 19.5 ± 1.6 weeks, Tongprasert and colleagues reported a 98.3% sampling success rate. In cases of continuing pregnancy, the total fetal loss rate was 10.5%; however, there were no fetal losses within 2 weeks of the procedure. There is therefore insufficient data to give a reliable procedure-related loss rate for PUBS in twins.
Rh-Alloimmunisation After Invasive Diagnostic Procedures
There is an increased risk for fetomaternal transfusion after amniocentesis, CVS and fetal blood sampling, which might have an immunising effect. However, the magnitude of this putative risk has not been determined. Nonetheless, Rh sensitisation after second trimester amniocentesis has clearly been observed. Thus there is a rationale for administering RhIG to Rh-negative women after genetic amniocentesis to prevent sensitisation. Indeed, Khalil and coworkers reported only one sensitisation among 300 (0.3%) at-risk women who received 300 mg of RhIG after amniocentesis. By contrast, among 615 Rh-negative women at risk for sensitisation who did not receive RhIG after amniocentesis, Golbus and coworkers reported that 12 (2.1%) became sensitised.
Similarly, an increase in alpha-fetoprotein and fetal red blood cells in maternal circulation has been demonstrated after CVS and fetal blood sampling. Therefore virtually all operators advocate routine use of RhIG after invasive procedures. However, the dose to be administered remains controversial. The ACOG currently recommends a 300-mg dose of RhIG after second trimester amniocentesis.
Effect of Noninvasive Prenatal Testing on Diagnostic Procedures
The risk for pregnancy loss after prenatal diagnostic testing resulted in interest in isolating fetal DNA from maternal serum. After the discovery by Lo and coworkers that pregnant women have cell-free DNA (cfDNA) in their plasma, several studies have been published confirming this finding and also that the proportion of cell-free fetal fraction can vary from 3% to 20% of maternal circulation. The initial challenges posed by the relatively small concentration of cfDNA in maternal plasma has been circumvented because of advances in molecular biology and sequencing technology.
Rapid advances in the field of noninvasive prenatal testing (NIPT) have occurred, resulting in the publication of results from several clinical trials. There are significant differences in the approach used in these trials that are important considerations when evaluating the potential impact on the prenatal diagnostic procedures. The study designs vary from retrospective cohorts to case-control to prospective approaches. In addition, the algorithms for generating results vary from z-score based systems to others incorporating both maternal and gestational ages. The majority of these studies are, however, from high-risk populations.
The advent of cell-free fetal DNA (cffDNA) in maternal blood has allowed near-diagnostic ability for fetal Down syndrome. It is, however, important to highlight some of the current limitations of NIPT. As mentioned earlier, the majority of published studies enrolled women at high risk for aneuploid for pragmatic reasons, but the few low- or mixed-risk studies published suggest that NIPT may be equally effective in these populations. As has been emphasised by many professional bodies, these and other limitations of NIPT necessitate labelling it a good screening test and not a diagnostic test. It is therefore important to emphasise that the current data and reports on the efficiency of cffDNA require a confirmatory cytogenetic testing for positive NIPT results because false-positive cases are beginning to be reported in the literature. Furthermore, with the availability of newer cytogenetic techniques that can detect subtle chromosomal abnormalities, indications for CVS and amniocentesis are expanding. There is, however, clear evidence that the introduction of NIPT has resulted in a substantial decline in the demand for invasive procedures. The complexity of choices available to women currently underlies the necessity for adequate counselling before diagnostic prenatal testing.
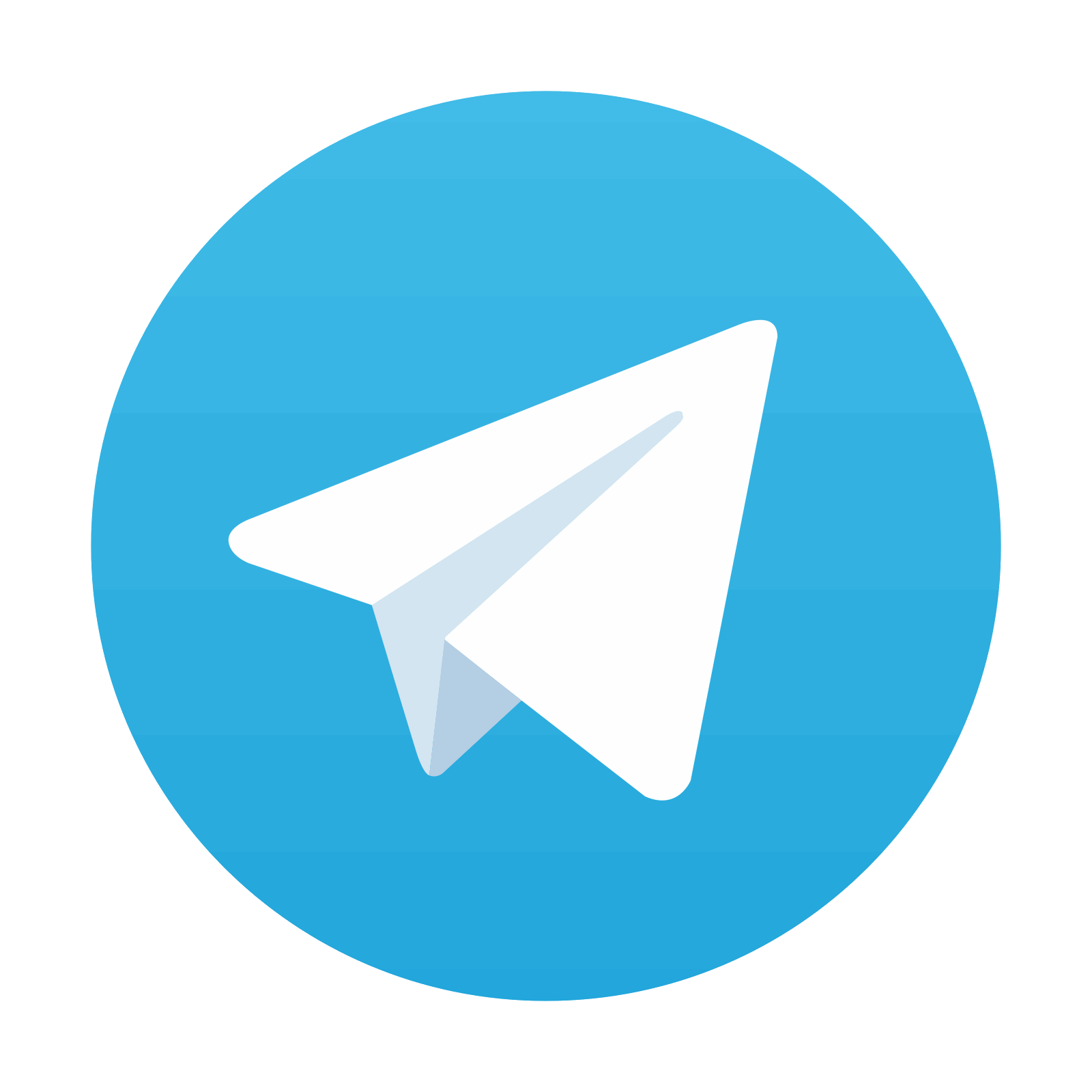
Stay updated, free articles. Join our Telegram channel

Full access? Get Clinical Tree
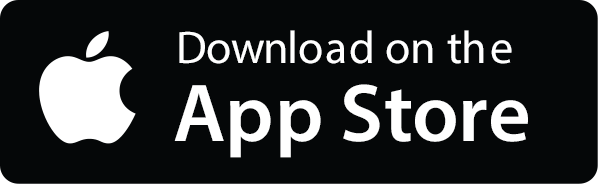
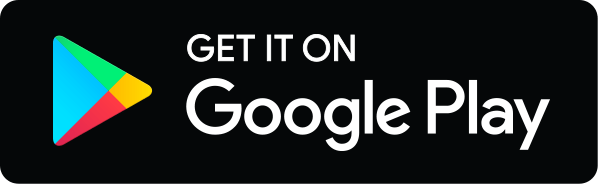