KEY POINTS
- 1.
Endotracheal tube ventilation, also known as invasive ventilation, although life-saving, is associated with lung injury, especially in premature infants.
- 2.
Volume-targeted ventilation is the preferred mode of invasive conventional mechanical ventilation.
- 3.
High-frequency ventilation is recommended as a rescue mode in conditions not responsive to conventional mechanical ventilation.
- 4.
Early nasal continuous positive airway pressure (NCPAP) and selective early surfactant use is beneficial over intubation and prophylactic surfactant administration in preterm infants at risk of respiratory distress syndrome.
- 5.
Nasal intermittent positive pressure ventilation is preferred over NCPAP to prevent extubation failure in preterm infants.
- 6.
There is not enough evidence to suggest the use of a high-flow nasal cannula as the primary respiratory support in preterm infants, although it is a reasonable alternative for postextubation support compared with NCPAP.
- 7.
Short binasal prongs are preferred for the delivery of noninvasive respiratory support.
- 8.
Noninvasive neurally adjusted ventilatory assist and nasal high-frequency ventilation are promising techniques on the horizon, but more trials are needed before recommending their routine use.
Introduction
Mechanical ventilation is perhaps the most significant life-saving intervention in neonatal medicine to date. Invasive ventilation refers to the ventilation of lungs through the endotracheal route. This is achieved by the use of either a conventional mechanical ventilator or in some circumstances a high-frequency ventilator. Although invasive mechanical ventilation definitely reduces neonatal mortality, there is some morbidity associated with its use. Newborn lungs, especially those of premature infants, are vulnerable to direct damage caused by ventilation, referred to as ventilator-induced lung injury (VILI). The mechanisms of VILI include damage caused by excessive stretch of the lung tissue from large gas volumes (volutrauma), by high airway pressures (barotrauma), by repeated alveolar collapse and reexpansion (atelectotrauma), and by release of inflammatory mediators from the injured alveolar epithelium (biotrauma). Injury to the immature lung can disturb the normal postnatal lung development, potentially leading to bronchopulmonary dysplasia (BPD), and it can also have untoward effects on other organs such as the brain, resulting in poor neurodevelopment. The recognition of these untoward effects sparked a renewed interest in noninvasive ventilation strategies. Noninvasive ventilation encompasses any form of ventilation delivered through the nares, and the established modes include nasal continuous positive airway pressure (NCPAP), nasal intermittent positive pressure ventilation (NIPPV), and high-flow nasal cannula (HFNC). Emerging techniques of noninvasive ventilation include neurally adjusted ventilatory assist (NIV-NAVA) and noninvasive high-frequency ventilation (NIHFV). The focus of this chapter is to discuss the various modalities of invasive and noninvasive ventilation and to review the evidence, or lack thereof, supporting their use in management of newborn respiratory disease.
Invasive Ventilation
Despite the recognition of the potential hazards of invasive mechanical ventilation, it remains an invaluable asset in the initial management of extremely preterm and critically ill infants. The goal of mechanical ventilation should be facilitation of adequate gas exchange with limitation of additional lung damage. Broadly, mechanical ventilation can be classified into two categories based on the tidal volumes delivered by the machine: (1) conventional mechanical ventilation (CMV), where volumes approximating physiologic tidal volumes are exchanged intermittently through a conventional ventilator, and (2) high-frequency ventilation (HFV), where low tidal volumes, at times less than the volume of the anatomic dead space, are exchanged at an extremely rapid rate through a high-frequency ventilator.
Conventional Mechanical Ventilation
There are multiple ventilator devices commercially available in the market, with each ventilator equipped to offer many different modes of ventilation. A thorough review of the devices, modes, and modalities of ventilation is beyond the scope of this chapter, and we chose to highlight the frequently used modes and modalities of mechanical ventilation and the rationale supporting their use in the next few paragraphs.
Modes of Ventilation
There are four main modes of CMV, depending on the manner in which the ventilator initiates and terminates the inspiratory phase of the respiratory cycle (e.g., based on time or on change in flow).
Intermittent Mandatory Ventilation
Intermittent mandatory ventilation (IMV) was the standard mode of ventilation used prior to the availability of synchronized ventilation. IMV is a time-cycled, pressure-controlled mode where the ventilator delivers a set number of mandatory inflations. Here, the operator sets the rate, peak inspiratory pressure (PIP), inspiratory time, positive end expiratory pressure (PEEP), and flow rate. The patient may breathe spontaneously between the mandatory breaths, and these spontaneous breaths are supported only by the PEEP, resulting sometimes in inadequate and unstable tidal volumes. Ventilator dyssynchrony is a major disadvantage of this mode, with potential consequences of gas trapping, air leaks, and intraventricular hemorrhage (IVH). , The mode is useful when the patient is completely apneic or is under heavy sedation, but it has largely been replaced by newer modes that can synchronize the inflations to the patient’s respiratory efforts and can deliver mandatory breaths.
Synchronized Intermittent Mandatory Ventilation
In synchronized IMV (SIMV) ( Fig. 12.1A ), the inflations delivered by the ventilator are synchronized to the onset of spontaneous patient breaths or are delivered at a mandatory rate if the patient has inadequate or absent respiratory effort. The operator sets the rate, PIP or tidal volume (depending on whether the modality is pressure controlled or volume targeted, respectively), inspiratory time (if time cycled), PEEP, and flow rate. The spontaneous breaths are supported by the PEEP, so there can be a wide variation in tidal volumes, depending on the respiratory effort of the patient. The advantages include faster weaning from mechanical ventilation and reduced need for sedation/paralysis due to ventilator-synchrony.




Assist Control
In assist control (AC) mode (see Fig. 12.1B ), every spontaneous breath is supported (assist), and the ventilator provides a set number of inflations if the patient does not breathe (control). AC is time cycled and pressure controlled or volume controlled. The operator sets the control rate, PIP or tidal volume (depending on pressure controlled or volume targeted modality, respectively), inspiratory time, PEEP, and flow rate. The advantages include ventilator synchrony and uniform tidal volume delivery for each breath and improved work of breathing compared with SIMV. It is important to set a control rate just below the infant’s spontaneous breath rate, because a high rate can make the patient not breathe spontaneously and a low rate can lead to excessive fluctuations in minute ventilation during periods of apnea. Weaning is done by reducing the PIP or tidal volume.
Pressure Support Ventilation
Pressure support ventilation (PSV) is a flow-cycled, pressure-controlled ventilation mode (see Fig. 12.1C ) used in spontaneously breathing patients and is similar to AC in that every breath is supported but is flow-cycled. The operator sets the PEEP, the flow rate, and a pressure support level, which is the support delivered to spontaneous breaths in addition to the PEEP. With flow cycling, an inflation is terminated when inspiratory flow declines to a preset threshold, generally 5% to 15% of peak flow. This eliminates the need for unnecessary inflation time (inspiratory hold) if inspiration is completed early. This results in automatic adjustment of inspiratory time depending on the lung mechanics and leads to better ventilator–patient synchrony. However, because the inspiratory time is typically shorter, this might result in lower mean airway pressures, and hence adjusting the PEEP as necessary is important to prevent atelectasis. PSV can also be used along with SIMV, where the SIMV rate serves as the control and the spontaneous breaths are supported by the pressure support.
Modalities of Ventilation
The modality refers to the target or limit variable of the mechanical breath. There are two modalities of CMV: pressure targeted and volume targeted.
Pressure Targeted Ventilation
Ventilation where the operator targets the pressure while the volume delivered is dependent on the lung mechanics is termed pressure targeted ventilation . This modality of ventilation can be delivered using any of the above-mentioned modes. Time-cycled, pressure-limited (TCPL) ventilators were used extensively in the past to provide this modality of respiratory support.
Volume-Targeted Ventilation
In volume-targeted ventilation (VTV) (see Fig. 12.1D ), the operator targets the volume, and the pressure delivered is dependent on the lung mechanics. Ever since the recognition that volutrauma, as opposed to barotrauma, is more injurious to the lung, there has been increased emphasis on the use of ventilation modalities that control the delivered tidal volume. Fluctuations in tidal volume can occur, with changes in lung mechanics leading to hyperventilation and excessive alveolar stretch on one hand and atelectasis and inadequate gas exchange on the other hand. Having control over the delivered tidal volume is therefore much more desirable than controlling the pressure. Despite the evidence of benefits of VTV over pressure-controlled (PC) ventilation, a survey of practicing neonatologists in the United States and Canada revealed that a majority of physicians still prefer to use PC ventilation. In this survey, half of the responders cited a lack of understanding as the reason for not using VTV. The use of uncuffed endotracheal tubes with resultant leak around the tube and the inability of the older ventilators to accurately measure the tidal volumes were potential barriers to the use of VTV for a long time. Most of the modern ventilators have the ability to measure exhaled tidal volume at the airway opening and have algorithms to calculate leak compensation, features that make the use of VTV more convenient.
The term volume-controlled (VC ) ventilation is not to be used synonymously with volume-targeted ventilation . The VC mode, which is frequently used in adult and pediatric populations, involves delivery of a set tidal volume into the proximal end of the circuit, and the pressure changes in inverse proportion to the lung compliance. Here, the set tidal volume is not the same as the delivered tidal volume due to factors such as variable endotracheal tube (ETT) leak and compression of gas in the circuit, making it difficult to assess the desired tidal volumes. In VTV, the operator chooses a target tidal volume and a pressure limit. The ventilator compares the exhaled tidal volume of the previous inflation and adjusts the pressure to reach the set tidal volume. There is a limit on the pressure increase from one inflation to the next to avoid excessive tidal volume delivery. Inflation is also terminated if the tidal volume exceeds a set percentage above the target volume, as a safety feature. Thus, autoregulation of the inflation pressure occurs with changing lung compliance, and this results in automatic weaning without the need to manually adjust the settings based on blood gas measurements. This feature of VTV is termed the volume guarantee (VG) mode on Draeger ventilators (Draeger Medical, Lubeck, Germany) and the pressure-regulated volume control mode on Servo ventilators (Maquet, Solna, Sweden). Keszler has discussed a detailed description of VTV and practical guidelines for its use in different disease conditions in a review article.
Evidence for Use of Different Modes and Modalities of Ventilation
Multiple studies on synchronized (patient-triggered) ventilation have shown beneficial effects of improved gas exchange and ventilation, consistent tidal volume delivery, and reduced breathing effort, stress, and blood pressure variability with use of synchronization. However, a Cochrane meta-analysis of randomized clinical trials (RCTs) comparing synchronized (patient-triggered) to nonsynchronized CMV demonstrated no difference in outcomes of mortality, BPD, air leaks, or IVH but a benefit of shorter duration of ventilation with synchronization (mean difference [MD], −38.3 hours; 95% confidence interval [CI], −53.90 to −22.69). The AC mode, compared with SIMV, was associated with a trend toward shorter duration of weaning in this meta-analysis (MD, −42.38 hours; 95% CI, −94.35 to 9.60). In another meta-analysis of 20 RCTs with patients on 1 of 16 different ventilation modes, TCPL, high-frequency oscillatory ventilation (HFOV) mode, SIMV + VG mode, and VC ventilation mode were associated with lower mortality compared with the SIMV + PSV ventilation mode. , There is moderate-quality evidence from multiple RCTs to support the use of VTV in neonates. A Cochrane review of 20 RCTs comparing VTV with pressure-limited ventilation in infants concluded that use of VTV had resulted in decreased rates of death or BPD at 36 weeks’ gestation (relative risk [RR], 0.73; 95% CI, 0.59–0.89), rates of pneumothorax (RR, 0.52; 95% CI, 0.31–0.87), mean days of mechanical ventilation (MD, 1.35 days; 95% CI, −0.86 to −1.83), rates of hypocarbia (RR, 0.49; 95% CI, 0.33–0.72), rates of grade 3 or 4 IVH (RR, 0.53; 95% CI, 0.37–0.77), and rates of the combined outcome of periventricular leukomalacia (PVL) with or without grade 3 or 4 IVH (typical RR, 0.47; 95% CI, 0.27–0.80). A similar meta-analysis of 18 RCTs by Peng et al. comparing the outcomes of these two modes of ventilation in preterm infants showed reduced BPD, rates of hypocarbia, duration of mechanical ventilation, failure of primary mode of ventilation, grade 3 or 4 IVH, pneumothorax, and PVL with VTV but no difference in the outcome of death. In a systematic review carried out to identify the optimal ventilation strategy in full-term newborns, the authors concluded that SIMV with a tidal volume of 6 mL/kg and a PEEP of 8 cm H 2 O may be advantageous in full-term newborns.
Infants must be weaned off invasive mechanical ventilation aggressively as tolerated and switched to noninvasive ventilation if needed to limit VILI. There are no RCTs comparing the efficacy of having a weaning protocol to limit exposure to ventilation and decrease the length of hospital stay. Rather than relying on a protocol, clinicians must be vigilant about the changing dynamics of the lung and make constant adjustments as required.
Recommendations
For infants needing intubation and mechanical ventilation, there is enough evidence to suggest the use of volume-targeted ventilation modes. Synchronization of ventilation might be beneficial, with advantages of faster weaning and shorter duration of mechanical ventilation, but there is no evidence of long-term benefit. When pressure targeted ventilation is used, care should be taken to aim for adequate tidal volumes that prevent volutrauma.
High-Frequency Ventilation
HFV refers to any form of assisted ventilation in which small tidal volumes are delivered to the patient at extremely rapid rates. The advantages of HFV include the ability to deliver tidal volumes less than that of the anatomic dead space and to use low transpulmonary pressures that can result in decreased volutrauma and barotrauma compared with CMV. The small tidal volumes and attenuated pressure amplitudes at the level of the alveoli also enable the use of higher mean airway pressures, thus helping to maintain optimal lung volume without exacerbating lung injury. Another advantage is the ability to adequately and independently manage oxygenation and ventilation with HFV.
Gas transport in HFV occurs based on the mechanisms of convective flow, convection and diffusion, diffusive flow, high-frequency pendelluft, and laminar flow with Taylor-type dispersion. The conventional linear relationship between minute ventilation, ventilator rate, and tidal volume does not apply in HFV. Small changes in tidal volumes bring about significant changes in ventilation. In fact, one of the primary risks of the use of HFV is the potential to cause hyperventilation leading to hypocarbia with subsequent cerebral injury, thus limiting its frequent use for respiratory insufficiency in neonates.
The two most commonly used forms of HFV are high-frequency jet ventilation (HFJV) and HFOV. Although a variety of devices are approved to deliver HFV, the approved devices most commonly used in the United States are the Bunnell Life Pulse jet ventilator (Bunnell, Inc., Salt Lake City, Utah) and the CareFusion 3100A High Frequency Oscillatory Ventilator (CareFusion, San Diego, California).
High-Frequency Jet Ventilators
In the HFJV form of HFV, short pulses of pressurized gas are directly injected into the upper airway through the ETT at a rapid rate ranging from 240 to 660 pulses per minute. The gas volumes delivered are very small and even less than that of the anatomic dead space. The jet ventilator is used in conjunction with a conventional ventilator that provides PEEP and can be used to deliver sigh breaths if needed. The operator sets the PIP, rate, and inspiratory time on the jet ventilator and adjusts the PEEP on the CMV. Exhalation during HFJV is passive and results from the elastic recoil of the lungs. The inspiratory times are short (0.02–0.034 seconds) with prolonged expiratory times, making this ventilator very effective in the management of air leaks. Inhaled nitric oxide can be delivered with HFJV.
High-Frequency Oscillation
In HFOV, air is moved back and forth at the proximal ETT by a piston attached to a diaphragm at a rapid rate (typically 480–900 per minute). A constant mean airway pressure is set, which helps in alveolar recruitment and oxygenation. The amplitude of the oscillations around this mean airway pressure determines the tidal volumes delivered to the lungs and thus affects ventilation. The rapid rates make the inspiratory times very short. The operator sets the mean airway pressure, amplitude, frequency, and inspiratory time, which makes HFOV relatively easier to use than HFJV. Exhalation is an active process in HFOV, resulting from the negative pressure applied during expiration. The ability to recruit lung volume by controlling the mean airway pressure directly also makes HFOV an effective device to deliver inhaled nitric oxide.
Evidence for Use of HFV in Neonatal Respiratory Disease
Despite animal studies showing favorable outcomes with use of HFV compared with CMV, the beneficial effects were not consistently reproduced in human studies. There are no RCTs directly comparing outcomes in preterm infants who were on HFJV versus HFOV. Hence, there is no evidence to support one over the other as an elective or rescue mode in these infants.
A Cochrane meta-analysis of 19 RCTs involving 4096 infants and comparing the elective use of HFOV versus CMV in preterm infants with respiratory distress syndrome (RDS) revealed no difference in mortality between the two groups. There was a reduction in chronic lung disease (CLD) at 36 to 37 weeks’ postmenstrual age in the HFOV group (17 RCTs; N = 2786 infants; RR, 0.86; 95% CI, 0.78–0.96) but at the expense of an increase in the incidence of air leaks (13 RCTs; N = 2854; RR, 1.19; 95% CI, 1.05–1.34). Caution must be exercised in interpreting these data; there was significant heterogeneity between the groups, and this analysis was done on RCTs conducted over 25 years, with significant perinatal and neonatal practice changes during the period. , A systematic review by Bhuta et al. on rescue use of HFOV versus CMV in preterm infants included 1 RCT with 182 infants and it showed no significant difference in mortality or CLD but reduction in any new air leak (RR, 0.73; 95% CI, 0.55–0.96) with use of HFOV. A Cochrane review of two RCTs (N = 199) comparing elective or rescue use of HFOV versus CMV in infants ≥35 weeks with pulmonary dysfunction did not show a difference in the studied outcomes, including mortality and CLD. Another Cochrane review of three RCTs compared the elective use of HFJV versus CMV in preterm infants with RDS and found reduced CLD at 36 weeks in the HFJV group (two RCTs; N = 170; RR = 0.59; 95% CI, 0.35–0.99) with no difference in mortality. Given no data on long-term pulmonary or neurodevelopmental outcomes from these trials, the authors did not recommend routine use of HFJV in preterm infants. An RCT by Keszler et al. compared the rescue use of HFJV versus CMV (N = 166) in preterm infants with pulmonary interstitial emphysema and reported no difference in their studied outcomes including mortality and CLD. A Cochrane review of the rescue use of HFJV in which only the trial by Keszler et al. met eligibility criteria concluded that there was not enough evidence to support routine use of HFJV as rescue therapy in preterm infants. Table 12.1 summarizes the Cochrane reviews comparing HFV with CMV. It is important to remember that some of the above studies were done in the presurfactant era and before synchronized ventilation gained acceptance.
Authors | Groups | Mode | RCTs/N | Criteria (GA, BW) | Mortality | CLD | Air Leaks |
---|---|---|---|---|---|---|---|
Cools et al. | HFOV versus CMV | Elective | 19/4096 | Preterm and low BW (<36 weeks, 2 kg) | No difference | Reduced CLD at 36 weeks with HFOV (RR, 0.86; 95% CI, 0.78–0.96) | Increased with HFOV (RR, 1.19; 95% CI, 1.05–1.34) |
Bhuta et al. | HFOV versus CMV | Rescue | 1/182 | <35 weeks | No difference | No difference in IPPV at 30 days | Any new air leaks reduced with HFOV (RR, 0.73; 95% CI, 0.55–0.96) |
Henderson-Smart et al. | HFOV versus CMV | Elective or rescue | 2/199 | ≥35 weeks | No difference | No difference | No difference |
Bhuta et al. | HFJV versus CMV | Elective | 3/262 | <35 weeks/<2 kg | No difference | Reduced CLD at 36 weeks with HFJV (RR, 0.58; 95% CI, 0.34–0.98) | No difference |
Rojas-Reyes et al. | HFJV versus CMV | Rescue | 1/166 | <35 weeks/<2 kg | No difference in overall mortality but decreased mortality with HFJV before crossover (RR, 0.66; 95% CI, 0.45–0.97) | No difference | No difference in new air leaks |
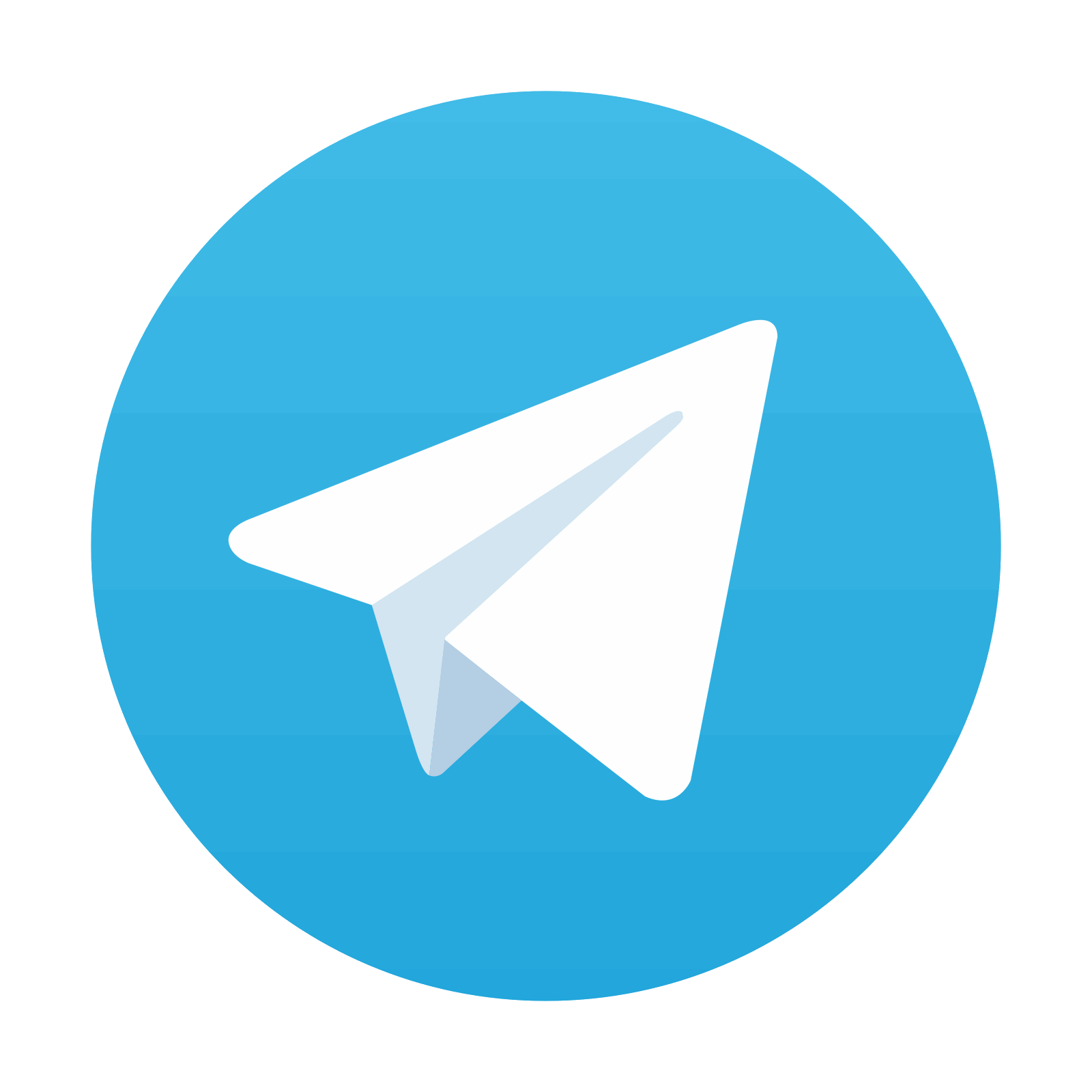
Stay updated, free articles. Join our Telegram channel

Full access? Get Clinical Tree
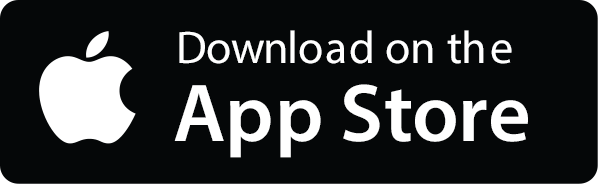
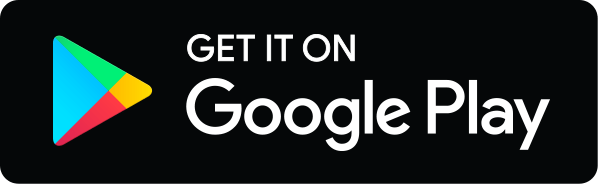
