Key Points
- •
A hallmark of allergic inflammation is the accumulation of a large number of leukocytes, including eosinophils, neutrophils, lymphocytes, basophils and macrophages, in the inflammatory tissues.
- •
Leukocyte migration into tissues is regulated by chemokines, chemoattractive cytokines.
- •
Th2 cytokines (e.g. interleukin [IL]-4 and IL-13) are potent inducers of allergy-associated chemokines (e.g. eotaxin).
- •
Chemokines are potent cellular activating factors.
- •
Leukocytes bind to the endothelium via low-affinity reversible interactions mediated by selectins, and tight adhesion of leukocytes to endothelium is mediated by specific adhesion molecules such as integrins.
- •
Animal and human experimental systems have demonstrated that allergic inflammatory responses are often biphasic.
Introduction
One of the hallmarks of allergic inflammation is the accumulation of an abnormally large number of leukocytes, including eosinophils, neutrophils, lymphocytes, basophils and macrophages, in the inflammatory tissue. There is substantial evidence that inflammatory cells are major effector cells in the pathogenesis of allergic disorders. Therefore, understanding the mechanisms by which leukocytes accumulate and are activated in tissues is very relevant to allergic diseases. Substantial progress has been made in understanding the specific molecules involved in leukocyte migration and the specific mechanisms by which effector cells participate in disease pathogenesis. In particular, cellular adhesion proteins, integrins and chemoattractant cytokines (chemokines) have emerged as critical molecules in these processes. Chemokines are potent leukocyte chemoattractants and cellular activating factors, making them attractive new therapeutic targets for the treatment of allergic disease. This chapter focusses on recently emerging data on the mechanisms by which specific leukocyte subsets are recruited into allergic tissues and how leukocytes participate in disease pathogenesis.
Animal and human experimental systems have demonstrated that allergic inflammatory responses are often biphasic. For example, asthma is characterized by a biphasic bronchospasm response, consisting of an early-phase asthmatic response (EAR) and a late-phase asthmatic response (LAR) ( Figure 5-1 ). The EAR is characterized by immediate bronchoconstriction in the absence of pronounced airway inflammation or morphologic changes in the airway tissue. The EAR has been shown to directly involve immunoglobulin(Ig) E/mast cell-mediated release of histamine, prostaglandin D 2 and cysteinyl-peptide leukotrienes (CysLTs), which are potent mediators of bronchoconstriction. After the immediate response, individuals with asthma often experience an LAR, which is characterized by persistent bronchoconstriction associated with extensive airway inflammation and morphologic changes to the airways. Clinical investigations have demonstrated that the LAR is associated with increased levels of inflammatory cells, in particular activated T lymphocytes and eosinophils ( Figure 5-1 ). The elevated levels of T lymphocytes and eosinophils correlate with increased levels of eosinophilic constituents in the bronchoalveolar lavage fluid (BALF), the degree of airway epithelial cell damage, enhanced bronchial responsiveness to inhaled spasmogens and disease severity In this chapter, we concentrate on understanding the inflammatory cells that participate in allergic responses, the mechanisms involved in their accumulation in natural human allergic responses and in experimental models, such as the biphasic response described above ( Figure 5-1 ), and the complex interplay of these diverse cells with resident cells including endothelial, epithelial, smooth muscle cells and fibroblasts.

Myelocytes
Eosinophils
Eosinophils are multifunctional leukocytes implicated in the pathogenesis of numerous inflammatory processes, especially allergic disorders. In addition, eosinophils play a role in homeostasis and may have a physiologic role in organ morphogenesis (e.g. postgestational mammary gland development) and the development of immune architecture, particularly the formation and function of IgA-secreting B cells. The gastrointestinal (GI) tract, spleen, lymph nodes, thymus, mammary glands and uterus are rich in eosinophils. In adipose tissue, eosinophils are important for the maintenance of glucose metabolic homeostasis. Additionally, experimental eosinophil accumulation in the GI tract is associated with the development of weight loss, which is attenuated in eotaxin-deficient mice that have a deficiency in GI eosinophils. It is important to note that recent attention has focussed on the key role of innate helper lymphoid cells (ILC), particularly ILC2, in regulating eosinophils via production of interleukin (IL)-5 and IL-13, and this regulation is related to nutritional intake in the GI tract.
Eosinophils express numerous receptors for chemokines (e.g. eotaxin, an eosinophil-selective chemoattractant) that, when engaged, lead to eosinophil activation, resulting in several processes, including the release of toxic secondary granule proteins ( Figure 5-2 ). The secondary granule contains a crystalloid core composed of major basic protein (MBP) and a granule matrix that is mainly composed of eosinophil cationic protein (ECP), eosinophil-derived neurotoxin (EDN) and eosinophil peroxidase (EPO). These granule proteins have been shown to be cytotoxic, helminthotoxic and virotoxic. Importantly, an anti-eotaxin antibody (bertilimumab) has been shown to decrease nasal congestion with a trend of decreased eosinophils in allergic rhinitis and is being studied currently for another eosinophil-related condition, ulcerative colitis.

Activation of eosinophils also leads to the generation of large amounts of LTC 4 , which induces increased vascular permeability, mucus secretion and smooth muscle constriction. Also, activated eosinophils generate a wide range of cytokines including IL-1, IL-3, IL-4, IL-5 and IL-13; GM-CSF; transforming growth factor (TGF)-α/β; tumor necrosis factor (TNF)-α; RANTES (regulated on activation, normal T cells expressed and secreted); macrophage inflammatory protein (MIP)-1α; and eotaxin. This indicates that they have the potential to sustain or augment multiple aspects of the immune response, inflammatory reaction and tissue repair processes. Eosinophils also have the capacity to initiate antigen-specific immune responses by acting as antigen-presenting cells. Consistent with this role, eosinophils express relevant costimulatory molecules (CD40, CD28, CD86, B7), secrete cytokines capable of inducing T cell proliferation and maturation (IL-2, IL-4, IL-6, IL-10, IL-12) and can be induced to express MHC class II molecules. Interestingly, experimental adoptive transfer of antigen-pulsed eosinophils induces antigen-specific T cell responses in vivo ( Box 5-1 ). Finally, it has been shown that the GI eosinophils have a unique and fascinating innate effector response. It appears that eosinophils may eliminate invading bacteria by ejecting their mitochondrial DNA, which is encased in highly cationic proteins. Evidence continues to emerge suggesting that eosinophils have an important role in innate immune responses, in addition to their well-established role in allergic disease.
- •
Eosinophils are multifunctional leukocytes that normally account for 1% to 3% of circulating leukocytes.
- •
Eosinophils normally reside in mucosal tissues such as the gastrointestinal tract.
- •
Eosinophil granules contain cationic (basic) proteins that are cytotoxic to a variety of host tissues (e.g. respiratory epithelium).
- •
Eosinophil expansion is regulated by the growth factor IL-5.
- •
Eosinophil tissue mobilization is regulated by the eotaxin subfamily of chemokines.
Eosinophils are important for the development of asthma-associated airway hyperresponsiveness (AHR). Eosinophils are the principal source of CysLTs and have been identified as the dominant source of leukotriene LTC 4 in asthmatic bronchial airway. These leukotrienes can initiate mucus hypersecretion, AHR and edema. MBP is cytotoxic to airway epithelial cells and may be at least partly responsible for the tissue damage that is associated with eosinophil infiltration in bronchial mucosa in asthma and has been associated with fatal asthma. Importantly, eosinophils have been implicated in the regulation of pulmonary T cell responses and appear to be required for complete Type 2 T helper cell (Th2) cytokine production and allergen-induced mucus production in the lung. Within the last few years, attempts have been made to further classify asthma phenotypes; one subtype is eosinophilic asthma, which is often a severe, steroid-refractory disease. Eosinophils selectively express the receptor for IL-5, a cytokine that regulates eosinophil expansion and eosinophil survival and primes eosinophils to respond to appropriate activating signals. Multiple studies have supported the beneficial usage of anti-IL-5 therapy for patients with asthma and nasal polyposis. Moreover, peripheral blood eosinophils and eosinophil granule protein levels are increased and correspond with disease activity in most patients with atopic dermatitis, and eosinophil granule proteins have been shown to be deposited in lesional skin. Eosinophil accumulation in the GI tract is a common characteristic of numerous disorders, including gastroesophageal reflux disease (GERD), inflammatory bowel disease (IBD), drug reactions, helminthic infections, hypereosinophilic syndrome (HES), eosinophilic GI disorders (EGIDs) and allergic colitis. EGIDs, including eosinophilic esophagitis (EoE), eosinophilic gastritis (EG) and eosinophilic gastroenteritis (EGE), often occur without peripheral blood eosinophilia, indicating the significance of GI-specific mechanisms for regulating local eosinophil levels. Although absent in the normal esophagus, eosinophils markedly accumulate in the esophagus of patients with EoE. A number of experimental models have provided evidence that eosinophils are key effector cells in EGIDs and contribute to the disease pathology. In allergic rhinitis during allergy season, there is an increase in eosinophils and their granule proteins. The above data collectively demonstrate the importance of eosinophils in allergic disease.
Mast Cells
Mast cells are normally present particularly in tissues in contact with the external environment (i.e. skin, respiratory mucosa, conjunctiva and GI mucosa). Mast cells contribute to immune responses to bacteria and venom and are important in homeostasis and wound repair. Mast cells are major effector cells involved in allergic responses; in addition, they are important cytokine-producing cells that are involved in nonallergic processes such as the innate immune response ( Figure 5-3 ). In contrast to other hematopoietic cells that complete their differentiation in the bone marrow, mast cell progenitors leave the bone marrow and complete their differentiation in tissues. Elegant studies in mice have demonstrated that development of mast cells from bone marrow cells is dependent on IL-3 and that their tissue differentiation is primarily dependent on stem cell factor (SCF). In contrast to the mast cell culture conditions in the murine system (which depend on IL-3), mature human mast cells are obtained by culturing progenitor cells with SCF, IL-6 and IL-10. Furthermore, treatment of mature human mast cells with IL-4 induces further maturation, including enhancing their capacity for IgE-dependent activation and their enzymatic machinery for synthesizing PGD 2 and CysLTs.

Mast cells exist as heterogeneous populations depending on the tissue microenvironment in which they reside and on the immunologic status of the individual. In work with rodents, the terms mucosal mast cell (MMC) and connective tissue mast cell (CTMC) have emerged, but designating these two populations of mast cells by tissue location alone is an oversimplification. In general, MMCs express less sulfated proteoglycans (chondroitin sulfate) in their granules than CTMCs and hence have different staining characteristics with metachromatic stains. In addition, mast cell populations express distinct granule proteases; in humans, the mast cell nomenclature is based on neutral protease expression. Human cells that express only tryptase (MC T ) are distinguished from mast cells that express tryptase, chymase, carboxypeptidase and cathepsin G (MC TC ). In normal tissues, MC T cells are the predominant cells in the lung and small intestinal mucosa, whereas MC TC cells are the predominant types found in the skin and GI submucosa.
Mast cell activation occurs through several pathways. Classically, a multivalent allergen cross-links IgE molecules bound to the high-affinity IgE receptor (FcεRI). Mast cells undergo regulated exocytosis of their granules, resulting in the release of preformed mediators; in addition, activated mast cells undergo de novo synthesis and release of a variety of potent mediators (such as prostaglandin D 2 and LTC 4 ). Preformed mediators in mast cells include biogenic amines such as histamine (a vasodilator), various neutral proteases, a variety of cytokines, acid hydrolases (e.g. β-hexosaminidase) and proteoglycans. Notably, nearly 20% of the protein of human mast cells is composed of tryptase, a proinflammatory protease with a wide range of activities (e.g. cleavage of complement proteins). Mast cells store a variety of cytokines in their granules (e.g. TNF-α, IL-1, IL-4, IL-5 and IL-6 and chemokines including IL-8) and, after activation with allergens or cytokines, mast cells can increase their synthesis and secretion of these cytokines.
It is well established that mast cell products contribute to the immediate allergic responses in asthma, anaphylaxis, allergic rhinoconjunctivitis and urticaria. There is also evidence that mast cells can contribute to allergic sensitization via IL-4-mediated skewing of T cells toward the Th2 phenotype via a mechanism involving dendritic cell activation of T cells.
The contribution of mast cell products such as cytokines has been less clear, though mast cells appear to be a chief source of TNF-α in asthmatic lung ( Box 5-2 ). In addition, mast cells have been shown to contribute to the chronic inflammation associated with the LAR in experimental asthma. The LAR also responds dramatically to omalizumab therapy, suggesting that LAR is initiated by mast cell activation during the EAR. Additionally, mast cells have been linked to more chronic, T cell-mediated allergic diseases such as atopic dermatitis and EoE.
- •
Mast cells are bone marrow-derived, tissue-dwelling cells.
- •
Mast cells do not normally exist in the circulation.
- •
Mast cell development is critically dependent on the cytokine stem cell factor and its receptor c-Kit.
- •
Mast cells express a high-affinity IgE receptor (FcεR) that is normally occupied with IgE.
- •
Mast cell activation results in the release of preformed mediators (e.g. histamine and proteases) and newly synthesized mediators such as prostaglandins and leukotrienes.
- •
Mast cells also produce cytokines such as tumor necrosis factor (TNF)-α and have an important role in innate immune responses (e.g. by attracting neutrophils).
Basophils
Basophils are hematopoietic cells that arise from a granulocyte-monocyte progenitor (GMP) that shares its lineage with mast cells and eosinophils. Basophils complete their development in the bone marrow and circulate as mature cells, representing less than 2% of blood leukocytes ( Box 5-3 ). Similar to mast cells, basophils express substantial levels of FcεRI and store histamine in their granules. They are distinguished from mast cells by their segmented nuclei, ultrastructural features, growth factor requirements, granule constituents and surface marker expression (c-Kit – , FcεRI + ). Basophils are more readily distinguished from eosinophils microscopically due to differences in their nuclei, cytoplasmic granules and appearance on hematoxylin- and eosin-stained tissues. In the human system, they develop largely in response to IL-3 in a process augmented by TGF-β. Mature basophils maintain expression of the IL-3 receptor, and IL-3 is a potent basophil-priming and -activating cytokine.
- •
Basophils are bone marrow leukocytes that normally account for less than 2% of circulating leukocytes.
- •
Basophils express the high-affinity IgE receptor FcεR.
- •
Basophils are distinguished from mast cells by their separate lineage, bilobed nuclei and distinct granule proteins.
- •
Basophils accumulate in tissues during late-phase responses.
Several processes activate basophils; upon cross-linking of their surface-bound IgE, basophils release preformed mediators including histamine and proteases and synthesize LTC 4 . In addition, they secrete cytokines such as IL-4 and IL-13; notably, the amount of IL-4 secreted by basophils compared with that by Th2 cells appears to be substantial. Similar to eosinophils, basophils are also activated by IgA (via FcαR) and by CCR3 ligands. Basophils also express several other chemokine receptors, including CCR2, whose ligands are potent histamine-releasing factors. Basophils also express major histocompatibility complex (MHC) class II and costimulatory molecules CD80 and CD86 and may be an antigen-presenting cell that can induce Th2 cell differentiation in the lymph node via IL-4.
Recent murine studies suggest that basophils help to expel helminths. Additionally, other animal models have suggested that basophils participate in the resistance of ectoparasitic ticks. Studies have linked basophils to the sensitization phase in EoE and the late-phase response in allergic rhinitis, asthma and allergic contact dermatitis. Basophils have also been implicated in a unique IgG-mediated mechanism of anaphylaxis in mice. It appears that this mechanism of anaphylaxis is dependent upon IgG, macrophages and platelet-activating factor (PAF). Elegant mouse studies have demonstrated that mice deficient in IgE, FcεRI and mast cells still experience anaphylaxis via an IgG-mediated process. However, IgG-mediated anaphylaxis is abolished in basophil-deficient mice. Further investigations are needed to define the role of basophils in this newly described anaphylactic pathway.
Macrophages
Macrophages are tissue-dwelling cells that originate from hematopoietic stem cells in the bone marrow and are subsequently derived from circulating blood monocytes. Under healthy conditions, bone marrow colony-forming cells rapidly progress through monoblast and promonocyte stages to monocytes, which subsequently enter the bloodstream for about 3 days, where they account for about 5% of circulating leukocytes in most species. On entering various tissues, monocytes terminally differentiate into morphologically, histochemically and functionally distinct tissue macrophage populations that have the capacity to survive for several months. Tissue-specific populations of macrophages include dendritic cells (skin, gut), Kupffer cells (liver) and alveolar macrophages (lung). Macrophage colony-stimulating factor (M-CSF) 1 promotes monocyte differentiation into macrophages, and mice with a genetic mutation in Csf1 have a deficiency of tissue macrophages. In addition, GM-CSF promotes the survival, differentiation, proliferation and function of myeloid progenitors, as well as the proliferation and function of macrophages.
Tissue macrophages contribute to innate immunity by virtue of their ability to migrate, phagocytose and kill microorganisms and to recruit and activate other inflammatory cells. By expressing Toll-like receptor mediated pathogen-recognition molecules that induce the release of cytokines capable of programming adaptive immune responses, macrophages provide important links between innate and adaptive immunity. Macrophages also express high- and low-affinity receptors for IgG (FcγRI/II) and complement receptors (CR1) that promote their activation. Activated macrophages produce a variety of pleiotropic proinflammatory cytokines such as IL-1, TNF-α and IL-8, as well as lipid mediators (e.g. leukotrienes and prostaglandins). Notably, macrophages express costimulatory molecules (e.g. CD86) and are potent antigen-presenting cells capable of efficiently activating antigen-specific T cells.
A substantial body of evidence has revealed that macrophages are critical effector cells in allergic responses. For example, peripheral blood monocytes from asthmatic individuals secrete elevated levels of superoxide anion and GM-CSF. In addition, the lung tissue and BALF from asthmatic individuals have elevated levels of macrophages. Consistent with this finding, the asthmatic lung overexpresses macrophage-attracting chemokines (e.g. mast cell protease [MCP]-1). Additionally, there appear to be different types of lung macrophages based on tissue location. Alveolar macrophages promote an inflammatory response, whereas interstitial macrophages have decreased phagocytosis and increased antiinflammatory effect. Alveolar macrophages have no antiinflammatory effect during the sensitization phase of lung immune responses, whereas interstitial macrophages suppress responses against inhaled allergens. Interstitial macrophages prevent Th2 polarization in response to inhaled antigens via an IL-10/dendritic cell mechanism.
We now recognize that there are at least two distinct subsets of macrophages, classically and alternatively activated macrophages. Classically activated macrophages (M1) are associated with a proinflammatory response and are activated by Th1 cytokines, whereas alternatively activated macrophages, so named because they are activated in the presence of Th2 cytokines, are associated with the resolution of inflammation and tissue repair. Alternatively activated macrophages (M2) may serve as a link between the innate and adaptive immune system, and further investigation into their function in allergic disorders is needed. A central mechanism for the differentiation of these macrophage subsets is the metabolism of arginine via two competing pathways, depending on their cytokine polarization. For example, interferon (IFN)-γ and lipopolysaccharide (LPS) augment the expression of inducible nitric oxide synthase (iNOS), which results in the production of NO as a product of arginine metabolism. NO is a potent smooth muscle relaxer and endothelial cell regulator. Alternatively, the treatment of macrophages with IL-4 or IL-13 induces the expression of arginase, which preferentially shunts arginine away from NO and thus promotes bronchoconstriction. Arginase metabolizes arginine into ornithine, a precursor for polyamines and proline, critical regulators of cell growth and collagen deposition, respectively. Both M1 and M2 macrophages have been reported in asthmatics.
Neutrophils
Neutrophils are bone marrow-derived granulocytes and account for the largest proportion of cells in most inflammatory sites. Neutrophils develop in the bone marrow by the sequential differentiation of progenitor cells into myeloblasts, promyelocytes and then myelocytes, an ordered process regulated by growth factors such as GM-CSF. Granulocyte-CSF promotes the terminal differentiation of neutrophils, which normally reside in the bloodstream for only 6 to 8 hours. A significant pool of marginated neutrophils exists in select tissues, allowing rapid mobilization of neutrophils in response to a variety of triggers (e.g. IL-8, LTB 4 , PAF).
Activated neutrophils have the capacity to release a variety of products at inflammatory sites, which may induce tissue damage. These products include those of primary (azurophilic), secondary (or specific) and tertiary granules, including proteolytic enzymes, oxygen radicals and lipid mediators (LTB 4 , PAF and thromboxane A 2 ). Neutrophil granules contain more than 20 enzymes; of these, elastase, collagenase and gelatinase have the greatest potential for inducing tissue damage. Neutrophil-derived defensins, lysozyme and cathepsin G have well-defined roles in antibacterial defense. In fact, studies have suggested that the major function of superoxide release into the phagocytic vesicle is increasing the intravesicular concentration of H + and K + , permitting conditions for optimal protease-mediated bacterial killing.
Although neutrophils are not the predominant cell type associated with allergic disorders, several studies have demonstrated a correlation and possible role for neutrophils in the pathogenesis of allergic disease. Individuals who die within 1 hour of the onset of an acute asthma attack have neutrophil-dominant airway inflammation, suggesting that neutrophils may have a pathogenic role in some clinical situations. Neutrophils in bronchial biopsy and induced sputum are more likely seen with severe asthma.
Patients with neutrophilic asthma appear to be less responsive to corticosteroids, and high doses of corticosteroids may increase neutrophils by reducing apoptosis. Another lung disease associated with asthma, allergic bronchopulmonary aspergillosis (ABPA), features neutrophilic inflammation and activation. Numerous risk exposures are linked to neutrophilic inflammation, including environmental exposures such as air pollution, smoking, infection and endotoxin, and health-related exposures such as high-fat, low-antioxidant diets, obesity and inflammatory states. Two fairly specific neutrophil therapies (anti-CXCL8 and anti-CXCR2) have begun to show promise in treating neutrophilic airway inflammation. Collectively, these data suggest an important role for neutrophils in the acute and chronic manifestations of allergen-induced asthma.
Dendritic Cells
Dendritic cells are unique antigen-presenting cells that have a pivotal role in innate and acquired immune responses. They are considered the quintessential antigen-presenting cells and are known for their ability to effect a primary immune response including allergen sensitization. These cells are also important in maintenance of allergic inflammation via propagation of effector responses. Dendritic cells originate in the bone marrow and subsequently migrate into the circulation before they assume tissue locations as immature dendritic cells, incidentally at locations where maximum allergen encounter occurs (e.g. skin, GI tract and airways). Immature dendritic cells are potent in antigen uptake, efficient in capturing pathogens and producers of potent cytokines (e.g. IFN-α and IL-12). By expressing pattern-recognition receptors, dendritic cells directly recognize a variety of pathogens. Immature dendritic cells express the CC chemokine receptor (CCR) 6 that binds to MIP-3α and β-defensin, which are produced locally in tissues such as those in the lung. After antigen uptake, dendritic cells rapidly cross into the lymphatic vessels and migrate into draining secondary lymphoid tissue. During this migration, the dendritic cells undergo maturation, which is characterized by down-regulation of their antigen-capturing capacity, up-regulation of their antigen-processing and -presenting capabilities, and up-regulation of CCR7, which likely promotes dendritic cell recruitment to secondary lymphoid organs (which express CCR7 ligands). After presentation of antigen to antigen-specific T cells in the T cell-rich areas of secondary lymphoid organs, dendritic cells mainly undergo apoptosis.
Dendritic cells are composed of heterogeneous populations based on ultrastructural features, surface molecule expression and function. In human blood, dendritic cells are divided into three types comprising two myeloid-derived subpopulations and one lymphoid-derived population (plasmacytoid dendritic cells). The myeloid populations can be divided into CD1 + and CD1 − . CD1 is a molecule involved in the presentation of glycolipids to T cells. CD1c + myeloid dendritic cells also express high levels of CD11c (complement receptor 4 [iC3b receptor]) and include interstitial and Langerhans dendritic cells; a skin-specific, self-renewing specialized dendritic cell; and inflammatory dendritic cells. CD1 − myeloid dendritic cells are identified by CD141 expression and are cross-presenting dendritic cells. The plasmacytoid dendritic cell population is CD1c − /CD11c − but is distinguished by its high levels of IL-3 receptor expression. This population of dendritic cells appears to be a primary source of IFN-α. Dendritic cells can be cultured from freshly isolated human cord or peripheral blood; myeloid dendritic cells are primarily derived in response to stimulation with GM-CSF, TNF-α and IL-4; plasmacytoid dendritic cells develop in culture with IL-3. Monocyte-derived dendritic cells give rise to inflammatory dendritic cells that have a potent stimulatory capacity toward naïve CD4 + T cells and the ability to cross-present antigen to CD8 + T cells and to produce key inflammatory cytokines including IL-1, IL-6, TNF-α, IL-12 and IL-23.
Dendritic cells can influence Th cell differentiation ( Figure 5-4 ). There is evidence that the same population of dendritic cells can influence Th1 and Th2 differentiation depending on several factors. For example, the ratio between dendritic cells and T cells has profound effects on influencing Th1 and Th2 differentiation. In addition, Th1-polarized effector dendritic cells induce Th1 responses, whereas Th2-polarized dendritic cells induce Th2 responses. Also, plasmacytoid dendritic cells stimulated first with IL-3 and then with CD40 ligand (before adding naïve T cells) induce strong Th1 responses but no Th2 cytokine production. MicroRNA (miR)-21 expression in dendritic cells targets IL-12p23; hence, up-regulation of miR-21 by Th2 cytokines introduces a Th2-generating propagation loop. Finally, dendritic cells that express specific costimulatory molecules may promote distinct Th differentiation; for example, expression of B7-related protein (ICOS ligand) promotes Th2 development.

Dendritic cells likely have critical roles in the development of allergic responses. Current evidence and theory suggest that allergen can induce a Th2-mediated response, either alone (with a self-adjuvant effect) or in combination with other environmental adjuvants (viral or bacterial infections or air pollution), and that this effect likely occurs via communication of resident stromal cells and dendritic cells. Pollen allergens in vitro have been shown to induce a Th2-polarizing dendritic cell, whereas house dust mite-mediated dendritic cell polarization is dependent on the allergy background of the patient. Dendritic cells are required for the development of eosinophilic airway inflammation in response to inhaled antigen. Importantly, adoptive transfer of antigen-pulsed dendritic cells has been shown to be sufficient for the induction of Th2 responses and eosinophilic airway inflammation to inhaled antigen. Elevated levels of CD1a + /MHC class II + dendritic cells are found in the lung of atopic asthmatics compared with of nonasthmatics ( Box 5-4 ).
- •
Dendritic cells normally exist as tissue surveillance cells.
- •
On contact with antigen (e.g. invading pathogen), dendritic cells migrate via lymphatics to secondary lymphoid organs.
- •
Immature dendritic cells are chief sources of innate cytokines (e.g. interferon [IFN]-α).
- •
Mature dendritic cells are potent antigen-presenting cells.
- •
Dendritic cells can preferentially activate T subset responses.
In addition to the importance of dendritic cells in sensitization, selective depletion of dendritic cells during allergen challenge in both asthma and allergic rhinitis murine models has demonstrated the importance of dendritic cells in the effector phase of these diseases. FcεRI expression on dendritic cells in humans and mice in asthma and atopic dermatitis is correlated with increased Th2 effector response.
Lymphocytes
Lymphocytes are integral to the development of a complete innate and adaptive immune response. One important function of lymphocytes is to generate adaptive immune responses and to develop a memory compartment for future responses. Innate lymphocytes serve as sentinel cells in epithelial-associated tissues, providing prompt release of cytokines that help to form the adaptive response. Lymphocytes both aid in pathogen defense and facilitate allergic disease. In addition to Th2 cells, many lymphocytes can participate in allergic inflammation including Th1 cells, Th17 cells, CD8 + T cells, B cells, γ/δ T cells, natural killer (NK) cells and natural killer T (NKT) cells.
T Cells
T cells are specialized leukocytes distinguished by their expression of antigen-specific receptors that arise from somatic gene rearrangement. Two major subpopulations were originally defined on the basis of the expression of the CD4 and CD8 antigens and their associated function. CD4 + T cells recognize antigen in association with MHC class II molecules on antigen-presenting cells, including dendritic cells, B cells and macrophages, and are primarily involved in orchestrating immune responses, whereas CD8 + T cells recognize antigen in association with MHC class I molecules and are primarily involved in cytotoxicity. Class I MHC molecules are present on the surface of all nucleated cells, and their antigens are typically intracellularly derived. More recently, populations of regulatory T cells have been characterized. Regulatory T cells are commonly identified as CD4 + /CD25 + /FOXP3 + T cells; these cells are chief sources of regulatory cytokines, including IL-4 and IL-10, and are thought to participate in tolerance induction after allergen immunotherapy. The absence of or decrease in the function of T regulatory cells leads to an increase in activity of effector T lymphocytes and is associated with the development of autoimmunity. CD4 + T lymphocytes have central roles in allergic responses by regulating the production of IgE and the effector function of mast cells and eosinophils.
CD4 + Th1-type T lymphocytes produce IL-2, TNF-β (lymphotoxin) and IFN-γ and are involved in delayed-type hypersensitivity responses. Th2 lymphocytes secrete IL-4, IL-5, IL-9, IL-10 and IL-13 and promote antibody responses and allergic inflammation ( Figure 5-4 ). Notably, there is a strong correlation between the presence of CD4 + Th2 lymphocytes and disease severity, suggesting an integral role for these cells in the pathophysiology of allergic diseases. Th2 cells are thought to induce asthma through the secretion of cytokines that activate inflammatory and residential effector pathways both directly and indirectly. The major T cell subset in allergic disease is Th2, but other subsets, such as Th1, CD8 + , Th9, Th17 and Th22, also participate, especially in severe disease. In fact, Th17 cells appear to be a critical component of the neutrophilic inflammation associated with severe asthma.
Th2-associated cytokines, IL-4 and IL-13, are produced at elevated levels in the allergic tissue and are thought to be central regulators of many of the hallmark features of the disease. However, in addition to Th2 cells, inflammatory cells within the allergic tissue also produce IL-4, IL-13 and a variety of other cytokines. IL-4 promotes Th2 cell differentiation, IgE production, tissue eosinophilia and, in the case of asthma, morphologic changes to the respiratory epithelium and AHR. IL-13 induces IgE production, mucus hypersecretion, eosinophil recruitment and survival, AHR and the expression of CD23, adhesion systems and chemokines. A critical role for IL-13 in orchestrating experimental asthma has been suggested by the finding that a soluble IL-13 receptor homolog blocks many of the essential features of experimental asthma. Furthermore, mice deficient in the IL-4Rα chain have impaired eosinophil recruitment and mucus production but still develop AHR.
Collectively, these studies have provided the rationale for the development of multiple therapeutic agents that interfere with specific inflammatory pathways. Additionally, as noted above, another important Th2-produced cytokine, IL-5, is important for eosinophil proliferation, survival and activation, and its inhibition has been linked to improved asthma and nasal polyposis ( Box 5-5 ).
- •
Mature T cells are primarily divided into CD4 + and CD8 + cells.
- •
T cells express antigen-specific T cell receptors (TCR) that recognize antigen in the context of major histocompatibility molecules (MHC).
- •
CD4 + T cells are engaged by antigen in the context of class II molecules.
- •
CD4 + T cells subsets include Th1, Th2, Th9, Th17, Th22 and T reg cells.
- •
Th1 cells are major producers of Th1 cytokines (e.g. interferon [IFN]-γ), and Th2 cells are major producers of Th2 cytokines (e.g. IL-4, IL-5, IL-13). Newly discovered subsets include Th9, Th17 and Th22, which are major producers of IL-9, IL-17A and IL-22, respectively.
B Cells
B cells play a key role in humoral allergic response through IgE production. Lymph nodes are the major anatomic structure of antigenic B cell education or affinity maturation. After these processes, B cells then become either memory B cells or antibody-producing plasma cells. IgE synthesis is regulated by IL-4 and IL-13 and may be augmented by IL-9. B cells also are found in gut lymphoid tissue and localized in diseased epithelial tissue. In allergic rhinitis, asthma and EoE, localized epithelial B cells produce Cε germ-line transcripts, as well as IL-4 and IL-13. Additionally, MHC class II-expressing B cells can function as antigen-presenting cells and drive Th2 cells.
Natural Killer Cells
NK cells lack rearranged antigen receptors and are considered part of the innate immune system. They produce high levels of IFN-γ early during infection and directly kill virally infected cells by release of cytotoxic granules and Fas ligand-induced cell death. Additionally, NK cells suppress Th2 allergic airway inflammation post respiratory syncytial virus. However, NK cells have also been shown to produce IL-5 and have been associated with eosinophilic inflammation.
Natural Killer T Cells
NKT cells are a population of CD1d-restricted T cells that express α/β T cell receptor (TCR), have some NK cell receptors, and share similar cytotoxic mechanisms to NK cells. Invariant NKT (iNKT) cells have a narrow repertoire of TCRs and respond to glycolipid antigen. iNKT cells can promote IgE production and, via cytokine production, may impact Th2 differentiation in the respiratory tract; they accumulate in the airways of asthmatics and increase with antigen challenge or exacerbation.
γ/δ T Cells
The γ/δ T cells are a subset of T cells that have a TCR formed from γ and δ chains rather than α/β chains. The γ/δ T cells reside in intraepithelial regions in the skin and mucosa and in lymphoid tissue. Intraepithelial γ/δ T cells do not recognize antigen in the context of MHC but rather respond to nonprocessed antigens, including lipids and heat shock proteins. They produce IFN-γ and IL-4 in vivo in response to Th1- or Th2-stimulating pathogens, respectively. γ/δ T cells that produce IL-4, IL-5 and IL-13 have been isolated from asthmatic airways and increase in number after antigen challenge. Interestingly, murine γ/δ T cells appear to be important both in the generation of Th2 immunity and protection from Th2-mediated disease.
Innate Lymphoid Cells
Populations of resident tissue lymphoid cells that lack B and T cell antigen receptors (BCR and TCR) and promote T helper cell development have been identified. These cells are lineage negative, meaning that they fail to express mature lymphocyte markers such as CD3, CD14, CD16, CD19, CD20 and CD56, but express high levels of stem cell markers such as CD117 (c-Kit). These cells are a small fraction of the total cell population in a given tissue but appear to be a major reservoir of T cell-skewing cytokines. Recently, it has been appreciated that these cells, like T helper cells, divide into subsets, and their nomenclature has been defined to reflect the cytokine and transcription factor similarity to their respective CD4 + T cell subsets Th1, Th2 and Th17. The proposed subsets include ILC1, ILC2 and ILC3.
ILC1s, like NK cells, are triggered by IL-12 to secrete high levels of IFN-γ and are dependent on T-bet, but unlike NK cells, they lack perforin and granzyme expression. ILC2 cells were identified in lung, intestine and blood. They produce IL-4, IL-5 and IL-13 in response to stimulation with IL-25, IL-33 and thymic stromal lymphopoietin (TSLP) and are dependent on GATA3. They are the first producers of IL-13 in the gut after helminth infection. ILC2s have been shown to be important in multiple Th2-mediated allergic diseases, including asthma and atopic dermatitis. As mentioned above, ILC2s are also important regulators of eosinophil homeostasis in the gut in a nutritionally dependent fashion. ILC3s secrete IL-17A and IL-22 upon IL-23 stimulation and are dependent on RORγt. The role of these cells in relation to specific disease phenotypes is being actively investigated ( Figure 5-5 ).

Leukocyte Recruitment
The trafficking of leukocytes into various tissues is regulated by a complex network of signaling events between leukocytes in the circulation and endothelial cells lining blood vessels. After injury or infection, resident cells at the site of injury or infection release chemokines, which interact via a gradient with the corresponding chemokine receptors on inflammatory cells. This chemokine : chemokine receptor interaction is critical for both leukocyte extravasation from the bloodstream into the tissue and leukocyte navigation within tissue to the site of injury or infection. Because each type of leukocyte expresses a different array of chemokine receptors, the type of inflammation that develops in a given situation is highly dependent on the chemokines secreted by resident cells. These interactions involve a multistep process: (1) leukocyte rolling (mediated by endothelial selectin and specific leukocyte carbohydrate ligands), which exposes chemokine receptors on leukocytes to chemokines displayed on endothelial cells; (2) rapid activation of leukocyte integrins, in which chemokine receptor signaling induces an inside-out signaling in the leukocyte leading to integrin clustering on the cell surface and an increase in the integrin’s affinity for its ligand; (3) firm adhesion between endothelial molecules and counterligands on leukocytes (via integrins); and (4) transmigration of leukocytes through the endothelial layer via junctional adhesion molecules (JAMs), platelet endothelial cell adhesion molecule (PECAM), CD99 and endothelial cell-selective adhesion molecule (ESAM) ( Figure 5-6 ). This multistep signaling cascade must occur rapidly to allow for the leukocytes to reduce rolling velocity, mediate adherence and extravasate into tissues in response to a chemokine gradient. In addition to mediating leukocyte movement from the bloodstream into tissues, chemokines use similar steps to mediate leukocyte-directed motion across other tissue barriers, such as respiratory epithelium and the extracellular matrix ( Box 5-6 ). Leukocyte adhesion deficiency (LAD) is a human disease associated with recurrent bacterial infections. The defect is in CD18, or β2 integrin, and results in the inability of neutrophils to be recruited from the blood to the site of infection.

Full access? Get Clinical Tree
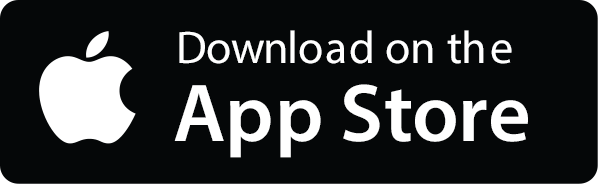
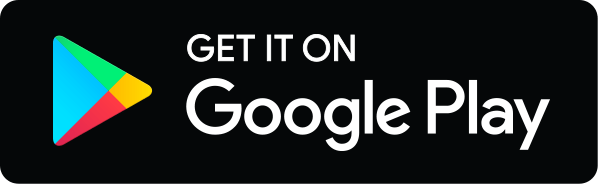