(4)
Department of Neonatology and Pediatric Pulmonary Unit, Bnai Zion Medical Center, The B. Rappaport Faculty of Medicine, Technion, Haifa, Israel
17.1.1 Introduction
The two main indications for respiratory support in preterm infants are respiratory distress syndrome (RDS) and apnea of prematurity.
RDS is the most common respiratory disorder in premature infants, and its incidence and severity increase with decreasing gestational age (Chard et al. 1997). In infants with birth weight between 501 and 1,500 g, more than 50 % have signs of RDS, increasing almost to 90 % in infants below 750 g (Hack et al. 1991).
The major cause of RDS in premature infants is lack of surfactant in the immature lung (Jobe 1993). That immature lung is susceptible to injury by various factors, including mechanical ventilation. Surfactant deficiency results in increased airway surface tension and collapsed alveoli at the end of expiration, atelectasis, uneven inflation, and regional alveolar over distension, which produces epithelial injury and pulmonary edema (Fig. 17.1). Thus, according to the “physiological rationale,” RDS should be treated by two means: (1) exogenous surfactant and (2) gentle support of functional residual capacity (FRC) with continuous positive airway pressure (CPAP).
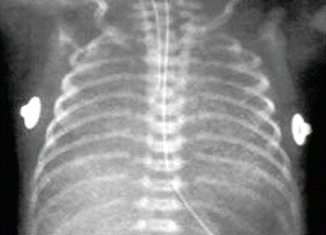
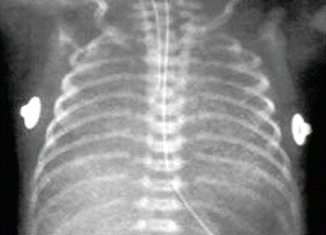
Fig. 17.1
Respiratory distress syndrome—chest radiograph
Surfactant was considered to be the “magic bullet” for treating RDS and reduced mortality of premature infants (Jobe 1993; Soll 1995; Lee et al. 1999). However, surfactant is administered via endotracheal ventilation, and this by itself can cause lung injury. Despite the new modes of ventilation (such as high-frequency ventilation, volume-targeted ventilation, triggered ventilation, and synchronized ventilation) and the use of surfactant, bronchopulmonary dysplasia (BPD) remains a significant morbidity in premature infants, and its incidence is correlated with the use of mechanical ventilation (Bollen et al. 2007; Greenough et al. 2008; Cools et al. 2010). Thus, ventilator-associated lung injury appears to be related to the duration of invasive ventilation via the endotracheal tube rather than the mode of ventilation. BPD is associated with poor neurological outcome (Majnemer et al. 2000).
Because mechanical ventilation via endotracheal intubation is associated with BPD, the trend today is to use nasal respiratory support (NRS). NRS is a noninvasive method of maintaining FRC without endotracheal ventilation (Fig. 17.2). NRS includes nasal continuous positive airway pressure (NCPAP) and nasal ventilation.
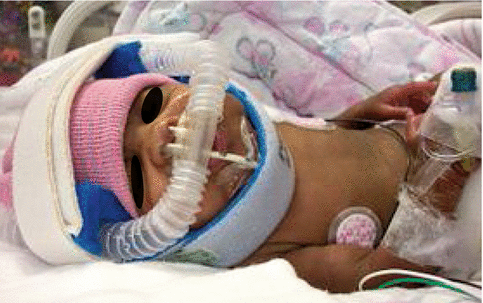
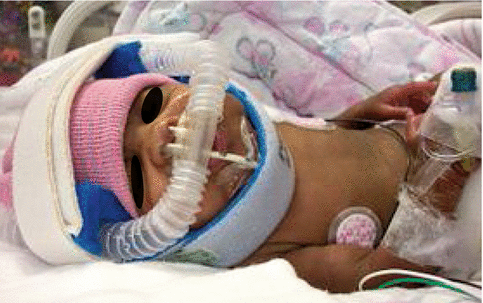
Fig. 17.2
Nasal respiratory support via nasal prongs
NRS has been used for the initial treatment of RDS, post-extubation as a “bridge” to spontaneous unsupported breathing, and for the treatment of apnea of prematurity. NCPAP was shown to be effective in treating infants with RDS (Gittermann et al. 1997; Ho et al. 2000; De Klerk and De Klerk 2001). To enhance the potency of NCPAP, it is possible to use nasal intermittent mandatory ventilation (NIMV). The rationale behind the use of NIMV is the administration of “sigh” to the infant, thus opening microatelectasis and recruiting more ventilation units (Lin et al. 1998).
Very premature infants with severe RDS may need the combination therapy of NRS and surfactant to avoid prolonged endotracheal ventilation. This is achieved by the INSURE (INtubation SURfactant Extubation) approach (Verder et al. 1999; Bohlin et al. 2007; Stevens et al. 2007), in which surfactant is administered during brief intubation followed by immediate extubation to NRS.
Term infants with respiratory morbidity (e.g., transient tachypnea of the newborn or meconium aspiration syndrome) may also require NRS. However, there is limited information on NRS in term infants. Thus, our discussion will focus on premature infants.
17.1.2 Nasal Respiratory Support During Neonatal Resuscitation in the Delivery Room
Recently, noninvasive ventilation was introduced to the delivery room, as even short duration of endotracheal intubation was shown to be associated with lung damage (Aly et al. 2005). Previous studies have shown that the lowest incidence of BPD occurred in neonatal intensive care units (NICUs) practicing early NCPAP instead of initial mechanical ventilation (Avery et al. 1987), and others reported a multivariable analysis to adjust for baseline risk and showed that most of the increased risk for BPD among VLBW infants resulted from the initiation of mechanical ventilation (Van Marter et al. 2000a).
There is no consensus as to the optimal mode of achieving early lung recruitment in the delivery room. te Pas and Walther (2007) evaluated 207 very preterm infants who were assigned randomly in the delivery room to either a sustained inflation through a nasopharyngeal tube followed by early NCPAP (early functional residual capacity intervention) or repeated manual inflations with a self-inflating bag and mask followed by NCPAP, if necessary, after arrival at the NICU. Needs for intubation, days on mechanical endotracheal intubation, days on NCPAP, air leaks, and moderate to severe BPD were significantly lower when a sustained inflation was used instead of bag and mask ventilation.
Flow-controlled pressure-limited mechanical devices, such as Neopuff Infant Resuscitators (Fisher & Paykel Healthcare Corp. Ltd., Irvine, Calif., USA) to deliver consistent CPAP in the delivery room, are recognized as an acceptable method of administering positive pressure ventilation during resuscitation, especially in preterm infants (Finer et al. 2001). Finer et al. (2001) concluded that the Neopuff, a purpose-built neonatal resuscitator ventilator, facilitates the delivery of the desired airway pressures while maximizing the operator’s ability to obtain and maintain a patent airway and facilitates the delivery of prolonged inflations.
Early administration of surfactant and immediate extubation in very preterm infants need to be considered to achieve a synergistic effect of NRS and surfactant (Sect. 17.1.7).
17.1.3 Nasal CPAP in the Premature Infant
NCPAP is currently a common practice for the treatment of premature infants with RDS (Dunn and Reilly 2003). NCPAP stabilizes the chest wall (Locke et al. 1991), reduces airway resistance (Miller et al. 1990; Gaon et al. 1999), increases FRC (Richardson and Jung 1978; Richardson et al. 1980), reduces obstructive apnea (Miller et al. 1985), and thereby improves lung volumes and oxygenation (Locke et al. 1991; Richardson and Jung 1978).
There are data from animal studies suggesting that CPAP elicits an attenuated inflammatory response in alveolar washes compared to mechanical ventilation (Jobe et al. 2002) and that surfactant treatment followed by CPAP results in less severe morphological lung injury than surfactant together with mechanical ventilation (Nold et al. 2007). Although physiologically appealing and, in parts of the world, associated with positive clinical experiences and outcomes, CPAP as a primary respiratory support option for preterm infants with RDS remains controversial due to lack of data on effectiveness from recent randomized trials (Subramaniam et al. 2005).
NCPAP was shown to be effective in treating infants with RDS and enables the avoidance of mechanical ventilation in a relatively large number of infants (Bollen et al. 2007; Greenough et al. 2008; Davis and Henderson-Smart 2003; Higgins et al. 1991; Kirchner et al. 2005). Morley et al. (2008) studied randomly assigned 610 infants who were born at 25–28 weeks gestation to CPAP or intubation and ventilation at 5 min after birth. In that study, almost half of the infants in the CPAP group required intubation during the first 5 days of life (Morley et al. 2008). Despite that, the odds ratio for death or oxygen treatment at 28 days favored early CPAP over mechanical ventilation. Other outcome measures were similar, apart from pneumothorax, which was increased with CPAP. In the (SUPPORT Study Group of the Eunice Kennedy Shriver NICHD Neonatal Research Network et al. 2010), 1,316 infants born between 24 0/7 weeks and 27 6/7 weeks of gestation were randomly assigned to intubation and surfactant treatment (within 1 h after birth) or to NCPAP treatment initiated in the delivery room, with subsequent use of a protocol-driven limited ventilation strategy. They found that “death or BPD” in the NCPAP versus surfactant groups did not differ (47.8 and 51.0 %, respectively). NCPAP versus surfactant treatment resulted in less delivery room intubations, postnatal corticosteroids for BPD, fewer days of mechanical ventilation, and need for mechanical ventilation by day 7. The authors concluded that their findings support the consideration of NCPAP as an alternative to intubation and surfactant in preterm infants. These results suggest that even very preterm infants may benefit from early CPAP.
Several centers administer surfactant, immediately extubate the infants, and then use NCPAP (Verder et al. 1999; Bohlin et al. 2007; Dunn and Reilly 2003), in order to shorten the course of mechanical ventilation.
When trying to determine if prophylactic nasal CPAP commenced soon after birth regardless of respiratory status in the very preterm or very low birth weight (VLBW) infant reduces the use of endotracheal ventilation and the incidence of BPD without adverse effects, the Cochrane review (Davis and Henderson-Smart 2003) concluded that there is currently insufficient information to evaluate the effectiveness of prophylactic nasal CPAP in very preterm infants. Neither of the included studies reviewed in that analysis showed evidence of benefit in reducing the use of endotracheal ventilation. The two recent large randomized trials (Morley et al. 2008; SUPPORT Study Group of the Eunice Kennedy Shriver NICHD Neonatal Research Network et al. 2010) did not show benefits in terms of reducing BPD in extremely low birth weight infants treated with early NCPAP.
Because the duration of endotracheal mechanical ventilation has a direct correlation with BPD, clinicians are increasingly using NRS to protect the preterm infant’s lungs and allow early extubation. NCPAP may be used post-extubation and thus decrease the incidence of reintubation (Davis and Henderson-Smart 2003). A meta-analysis of trials, assessing whether CPAP facilitates extubation in 569 infants, demonstrated that use of NCPAP reduced the need for increased respiratory support (defined as if they required higher supplementary oxygen levels than when immediately extubated and, in addition, if the headbox oxygen infants required rescue CPAP). However, NCPAP did not significantly reduce the need for reintubation or influence the oxygen requirement at 28 days (Dimitriou et al. 2000).
Nasal CPAP was used also for the treatment of apnea of prematurity (Davis and Henderson-Smart 2003; Lemyre et al. 2002). CPAP is thought to decrease the frequency of apnea by increasing the FRC and stabilizing oxygenation and/or by splitting the upper airway with positive pressure. NCPAP abolishes obstructive and mixed apnea, but not central apnea (Miller et al. 1985). It may act by distending and maintaining the upper airway rather than by a direct effect on respiratory drive. NCPAP also stabilizes the highly compliant chest wall of the infant by increasing the end-expiratory lung volume, thus improving pulmonary mechanics (Pandit et al. 2001).
NCPAP is effective and has an acceptable safety margin for road-based transportation of infants with RDS. Air transport is feasible, but larger studies are required to assess safety (Murray and Stewart 2008). This conclusion is based on a retrospective study on 220 infants treated with NCPAP, of whom 207 were transported on CPAP of 7 cm H2O. Thirty infants were transported by fixed or rotary wing aircraft and 190 by road. No infant required intubation or bag and mask ventilation during transport.
17.1.4 Nasal Intermittent Mandatory Ventilation (NIMV) in the Premature Infant
It is possible to enhance NRS by using NIMV. The rationale behind the use of NIMV is the administration of “sigh” to the infant, thus opening microatelectasis and recruiting more ventilation units (Lin et al. 1998). In apneic infants, NIMV may improve the patency of the upper airway by creating elevated pharyngeal pressures, and by intermittent inflation of the pharynx, it could activate respiratory drive (Moretti et al. 1999).
Nasal intermittent positive pressure ventilation (NIPPV) and NIMV are used interchangeably in the literature. The common definition from Davis et al. is NIMV is a method of augmenting NCPAP by delivering ventilator breaths via nasal prongs (Davis and Henderson-Smart 2003). By that definition, NIMV mode is not an assist control mode, where the infant breath triggers a ventilator breath and where the triggering might be of greater importance.
Several investigators have tried to synchronize NIMV with the infant’s breathing by using Infant Star ventilators (Infrasonics, Inc, San Diego, CA) that employed an abdominal pressure-sensing capsule (Davis and Henderson-Smart 2003; Barrington et al. 2001; Khalaf et al. 2001). Moretti et al. found that application of nasal synchronized intermittent mandatory ventilation (NSIMV) was associated with increased tidal volume and minute volume as compared with NCPAP (Davis and Henderson-Smart 2003). NSIMV was shown to be associated with improved thoracoabdominal synchrony and thus stabilizes the chest wall and improves lung mechanics (Kiciman et al. 1998). NSIMV may have advantages over NIMV. Positive pressure ventilator breaths are delivered only after initiation of respiratory effort by the infants, when the glottis is likely to be open, or after an apneic interval. It was shown that NSIMV compared to NCPAP decreases the work of breathing in premature infants (Aghai et al. 2006). Synchronization of nasal ventilation is problematic especially in small premature infants. The systems are open, and the flows and pressures generated by the premature infants are very small. Also, the abdominal sensor (capsule) may be triggered by motion artifacts other than breathing. While the effectiveness of both pressure-triggered ventilation (Kiciman et al. 1998) and NSIMV (Davis and Henderson-Smart 2003; Kiciman et al. 1998; Aghai et al. 2006) was evaluated, its use was not shown yet to be more beneficial than NIMV.
The first study to use NIMV as a primary ventilatory mode for treatment of RDS was the study of Kugelman et al. (2007). This study showed that NIMV compared to NCPAP decreased the requirement for endotracheal ventilation in premature infants (<35 weeks) with RDS (Fig. 17.3). Failure of NIMV in this study was significantly associated with lower birth weight. Other studies also correlated failure of nasal support with low birth weight or gestational age (Ammari et al. 2005). Success on NRS depends not only on the severity of RDS, birth weight, and gestational age but also on the experience of medical teams, which is increasing in recent years. Another prospective trial that used NSIMV during the acute phase of RDS just after surfactant administration is the study of Bhandari et al. (2007). They concluded that infants of birth weight 600–1,250 g with RDS receiving surfactant with early extubation to NSIMV had a significantly lower incidence of BPD/death (52 % versus 20 %, p = 0.03) and that primary mode NSIMV is a feasible method of ventilation in small premature infants. “Too sick” infants were excluded from that study which was more of a feasibility study. The best option for treatment of RDS with respect to gestational age and RDS severity should be further investigated (Dunn and Reilly 2003).
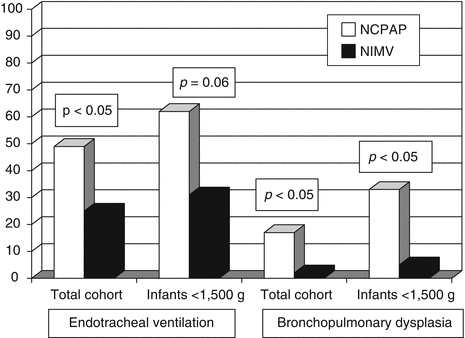
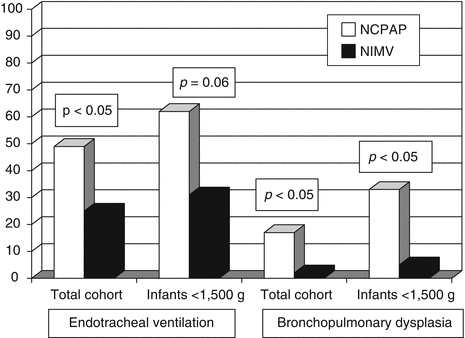
Fig. 17.3
Endotracheal mechanical ventilation and bronchopulmonary dysplasia (BPD) in infants treated with NIMV and NCPAP (Reproduced with permission from Kugelman et al.)
NSIMV was shown to be more effective than NCPAP immediately after extubation in the treatment of RDS (Moretti et al. 1999; Barrington et al. 2001; Khalaf et al. 2001; Friedlich et al. 1999; De Paoli et al. 2003; Davis et al. 2001). Three randomized controlled trials have investigated the effect of NSIMV and NCPAP after extubation (Barrington et al. 2001; Khalaf et al. 2001; Friedlich et al. 1999). All three studies showed that more NSIMV-treated infants remained extubated at 48–72 h. A pooled meta-analysis (159 infants) showed that NSIMV was more effective than NCPAP in preventing failure of extubation [RR 0.21 (0.10, 0.45)] and the number needed to treat was only three infants to prevent one extubation failure (Bhandari et al. 2007; Friedlich et al. 1999). Some infants randomized to NCPAP, and who fulfilled the reintubation criteria, were not reintubated but were given “rescue” NIMV. Eight out of nine of these infants remained extubated. The numbers were too small to detect reliable differences in the rate of BPD, days of supplemental oxygen, or hospital stay (Barrington et al. 2001; Khalaf et al. 2001). Recently, Moretti et al. have shown that by using nasal flow SIMV in infants <1,251 g post-extubation, 94 % (30/32) of infants were successfully extubated as compared to only 61 % (19/31) who received NCPAP (p < 0.005) (Moretti et al. 2008).
The time of extubation, early or only at RDS resolution, depends on the infants’ gestational age. In 28–34 weeks’ gestation, Santin et al. (2004) showed that infants of 28–34 weeks’ gestational age with RDS requiring surfactant with early extubation immediately postsurfactant to SNIMV had a shorter duration of intubation and decreased need for oxygen as compared to conventional ventilation. There was also a significant decrease in the duration of parenteral nutrition and hospitalization. They concluded that NSIMV/NIMV seems to be safe and effective primary mode of ventilation in larger premature infants. Yet, Bhandari et al. (2007) in a more recent trial found it also correct for small premature infants (600–1,250 g).
NSIMV was shown to be more effective than NCPAP also for apnea of prematurity (Lin et al. 1998; Lemyre et al. 2002). Ryan et al. (1989) randomized 20 premature infants with apnea, on NCPAP, to a 6-h crossover study of NIMV or NCPAP. They did not find any differences in number or severity of apneas. They noted that during some apneas, the peak pressure of 20 cm H2O did not produce chest movement and postulated that these apneas were due to upper airway obstruction. In 1998, Lin et al. (1998) randomized 34 spontaneously breathing infants with apnea to 4 h of either NCPAP or NIMV. The NIMV group had a significant reduction in the severity and number of apneas (3.5/h to 0.8/h, compared with 2.6/h to 1.5/h in the NCPAP group, p = 0.02). A meta-analysis of these small trials, regarding apnea of prematurity, suggests that NIMV is more efficacious with apnea that is frequent or severe. However, the studies performed addressed short-term outcomes and as such could not address properly the incidence of requirement for reintubation. Thus, more studies are needed before recommending NIMV as standard of care for apnea of prematurity (De Paoli et al. 2003).
Pressure support ventilation (PSV) is a mode of synchronized ventilation that can supplement the spontaneous breathing effort by assisting each of the infant’s spontaneous breath. A pressure support breath is initiated and terminated in synchrony with the patient’s inspiratory effort.
Due to weak musculature and a compliant ribcage, preterm infants are prone to chest wall distortion during breathing. The underlying lung disease further increases their respiratory workload, and the provision of continuous distending pressure alone is often not sufficient to support their breathing. Thoracoabdominal asynchrony, which occurs because of the negative intrapleural pressure changes transmitted to the highly compliant chest wall of preterm infant, has been suggested to be more effectively reduced by NSIMV compared to NCPAP, perhaps by better stabilization of chest wall (Kiciman et al. 1998). When compared to NCPAP, noninvasive PSV did not increase minute ventilation, but it effectively unloaded the patient’s respiratory pump as indicated by a lower inspiratory effort and reduced chest wall distortion (Ali et al. 2007).
17.1.5 Nasal Flow for Premature Infants
Nasal cannulae are frequently used to deliver oxygen. Its use is popular because it allows access to the infant and enables the parents to hold the infant. The mobility is also important in older infants for developmental stimulation. While the method is commonly used in infants with chronic lung disease, the medical teams tend to use it earlier because of its convenience. However, one should be cautious with that use because the amount of oxygen delivered should be controlled (by flow and mixer) and the amount of flow should be controlled as it may cause inadvertent CPAP when the outer diameter of the cannula is 0.3 cm (Santin et al. 2004). Complications reported with the use of high-flow nasal cannula included air leaks (Jasin et al. 2008), gas trapping (Locke et al. 1993), and nasal mucosal injury (Woodhead et al. 2006).
Nasal flow may be indicated as nasal support post-extubation and for apnea of prematurity. Besides oxygen and probably triggering the infant to breathe by the flow itself, nasal flow may provide CPAP (Locke et al. 1993), and this may explain its beneficial effect. When comparing the work of breathing in premature neonates supported with high-flow nasal cannula (HFNC) and NCPAP, it was found that HFNC provided support comparable to NCPAP (Saslow et al. 2006).
Woodhead et al. showed that HFNC that delivers humid and warm nasal flow, performed better than standard HFNC in maintaining lower respiratory effort score and averting reintubation following endotracheal extubation (Woodhead et al. 2006). Shoemaker et al. (2007), in a retrospective study, reported that HFNC usage increased (64 %) after its introduction in infants of all gestational ages, whereas the usage of NCPAP decreased from 19 to 4 %. They found that comparing the cohort of infants who received either NCPAP or HFNC as an early mode of respiratory support, there were no differences in deaths, ventilator days, BPD, blood infections, or other outcomes. More infants were intubated for failing early NCPAP compared to early HFNC (40–18 %). Use of nasal cannula flow in which CPAP can be delivered with flows exceeding 1 l/min has been suggested as an equivalent treatment modality to NCPAP (Sreenan et al. 2001). Sreenan et al. concluded that nasal cannula at flows of 1–2.5 l/min can deliver positive distending pressure in premature infants and that the HFNC is as effective as NCPAP in the management of apnea of prematurity (Sreenan et al. 2001). However, no randomized prospective study was published yet that compared NCPAP or NIMV versus HFNC.
17.1.6 Contraindications for Noninvasive Respiratory Support
NRS is not possible in a premature infant who needs to be resuscitated with endotracheal intubation immediately after delivery. Endotracheal intubation should be performed in the delivery room if heart rate does not increase to >100 per minute, if the infant has insufficient spontaneous respiratory effort, or if he shows marked and increasing dyspnea (Lindner et al. 1999).
If endotracheal intubation is not required for resuscitation, the neonatal team should decide whether to intubate every extremely premature infant at risk for RDS immediately and to administer prophylactic surfactant or to consider a trial of NRS. Preventive surfactant administration was shown to be more beneficial than rescue therapy in infants <28 weeks’ gestation (Soll and Morley 2001). To note, the included studies in that review were conducted in the context of less than optimal use of antenatal steroids. The impact of prophylactic surfactant may be less dramatic in the setting of increased treatment with antenatal steroids.
Furthermore, it was shown by several investigators that individualized intubation strategy may be safe (Aly et al. 2005; Morley et al. 2008; SUPPORT Study Group of the Eunice Kennedy Shriver NICHD Neonatal Research Network et al. 2010; Lindner et al. 1999). Lindner et al. (1999), in a retrospective cohort study, showed that adopting a policy of selective intubation in extremely low birth weight (ELBW) infants resulted in a significantly reduced need for intubation, lower incidence of BPD, intraventricular hemorrhage (IVH), and reduced length of stay. Aly et al. (2005), in a retrospective study, concluded that VLBW infants treated successfully with early NCPAP were unlikely to develop IVH of grade III or IV. Infants who experienced early NCPAP failure were at increased risk for the development of necrotizing enterocolitis. Infants who were intubated briefly in the delivery room were at increased risk for prolonged oxygen requirement. Morley et al. (2008) studied randomly assigned 610 infants who were born at 25–28 weeks’ gestation to CPAP or intubation and ventilation at 5 min after birth. In that study, early nasal CPAP did not significantly reduce the rate of death or BPD despite fewer days of endotracheal ventilation as compared with intubation. Yet, apart from increased rate of pneumothorax, there were no other serious adverse events in the CPAP group. Pneumothorax in that study was not associated with long-term pulmonary or neurological outcomes. The SUPPORT study (SUPPORT Study Group of the Eunice Kennedy Shriver NICHD Neonatal Research Network et al. 2010) also found that NCPAP is a safe alternative in extremely low birth weight infants.
17.1.7 Adjunct Therapies: Surfactant to Avoid Mechanical Ventilation
NRS is an effective mode of treatment for premature infants with RDS, especially in the era of prenatal corticosteroids. Antenatal steroids were found to reduce the rate of RDS (RR 0.66, 95 % CI 0.59–0.73, 21 studies, 4,038 infants) and need for respiratory support (Roberts and Dalziel 2006). Antenatal corticosteroid treatment clearly reduces the incidence of RDS in randomized controlled trials (Crowley et al. 1990; Kari et al. 1994).
Yet, the severity of RDS, the infant’s weight and gestational age, and the experience of the medical teams with NRS are significant factors that affect the success of noninvasive ventilation.
Finer et al. (2004) reported that CPAP/PEEP given by Neopuff in the delivery room to ELBW infants did not affect the need for intubation at birth or during the subsequent week. Overall, 20 % of infants did not need intubation by 7 days of life. Aly et al. (2005) reported that the chance of successful maintenance with early NCPAP for >48 h was not demonstrable at <24 weeks’ of gestation (10 % success). The efficacy of the use of early NCPAP improved significantly by 25 weeks of gestation (45 % success). In the study of (Morley et al. 2008) (gestational ages of 25–28 weeks), 46 % of infants in the CPAP group were intubated during the first 5 days. The intubation rate was 55 % for infants born at 25 or 26 weeks’ gestation and 40 % for those born at 27 or 28 weeks’ gestation. The median time for intubation was 6.6 h (interquartile range, 2.2–19.3). The reasons for intubation were as follows: a FiO2 of 0.60 or more (53 % of infants), a PaCO2 of more than 60 mmHg (8.0 kPa) (41 %), apneic episodes (38 %), or metabolic acidosis unresponsive to treatment (21 %); some infants had multiple reasons for intubation. Another 39 patients in the CPAP group (12.7 %) were intubated after 5 days of age. Thus, while noninvasive ventilation is probably safe, its success depends on gestational age and on RDS severity. Lack of surfactant contributes to NRS failure in the most premature infants.
Surfactant replacement treatment significantly reduces mortality in infants with RDS (Soll 1995). The introduction of surfactant therapy in the USA was reflected in an accelerated reduction in mortality from RDS and was the single most important factor for the decrease in overall neonatal mortality rate in the early 1990s (Lee et al. 1999).
Despite the effectiveness of surfactant treatment in the acute phase of RDS and new ventilation techniques such as high-frequency oscillation and volume-targeted ventilation, bronchopulmonary dysplasia (BPD) remains an important adverse outcome in preterm infants, and its incidence is correlated with use of mechanical ventilation (Bollen et al. 2007; Greenough et al. 2008; Van Marter et al. 2000). Thus, it seems that NRS and surfactant may have a synergistic positive effect in extremely premature infant.
It was shown that combining surfactant with noninvasive respiratory support is beneficial. A recent multicenter study found that when 279 infants, born between 27 and 31 weeks’ gestation, were randomly assigned within the first hour of life to intubation, very early surfactant, extubation, and NCPAP (treatment group) or NCPAP alone (control group), the addition of very early surfactant therapy without mandatory ventilation decreased the need for subsequent mechanical ventilation, decreased the incidence of air-leak syndrome, and seemed to be safe (Rojas et al. 2009). Stevens et al. (2007), in a Cochrane analysis, compared two treatment strategies in preterm infants with or at risk for RDS: early surfactant administration with brief mechanical ventilation (less than 1 h) followed by extubation versus later selective surfactant administration, continued mechanical ventilation, and extubation from low respiratory support. They concluded that the first approach was associated with less need for mechanical ventilation, lower incidence of BPD, and fewer air-leak syndromes.
The European CURPAP study is currently evaluating the efficacy of early CPAP and natural surfactant as a combination therapy versus early NCPAP and rescue surfactant for very preterm infants (Sandri et al. 2008). Implementing a strategy of surfactant administration by transient intubation during NCPAP reduces the need for mechanical ventilation (by ~50 %) without adverse effects on outcome and may be an option to more effectively treat RDS, particularly in a care setting where transfer is necessary to provide mechanical ventilation (Verder et al. 1999). Dani et al. (2004) have shown in infants <30 weeks’ gestation that the immediate reinstitution of NCPAP after surfactant administration for infants with RDS is safe and beneficial, as indicated by the lesser need for mechanical ventilation and the shorter requirement for respiratory supports, compared with the institution of mechanical ventilation after surfactant treatment. Geary et al. (2008) performed a historical cohort study of appropriately sized preterm infants. Only 1 (1 %) of 87 infants in the pre-early management practice change group received CPAP treatment in the first 24 h of life, compared with 61 (80 %) of 76 infants in the post-early management practice change group. The proportions of infants who required any SIMV during their hospital stays were 98.8 and 59.5 %, respectively. The mean durations of SIMV were 35 days and 15 days, respectively. The combined incidence rates of moderate and severe BPD at corrected gestational age of 36 weeks were 43 and 24 %, respectively. They concluded that successful early management of extremely preterm infants with surfactant treatment followed by CPAP treatment at delivery, lowered oxygen saturation goals, and early amino acid supplementation is possible and is associated with reductions in the incidence and severity of BPD.
Kribs et al. (2008; 2007; 2009) developed a method of surfactant administration during spontaneous ventilation on NCPAP in ELBW infants using thin endotracheal catheter. They reported in a retrospective trial that the choice of NCPAP as initial airway management significantly increased from 69 to 91 % and for NCPAP with surfactant from 75 to 86 %. The rate of NCPAP failure decreased from 46 to 25 %. Survival increased significantly from 76 to 90 %, and survival without BPD rose from 65 to 80 %. No changes in nonpulmonary outcomes were observed.
Perspectives and Future Developments
The current data suggest that in “extremely premature” infants, endotracheal ventilation, surfactant administration, and extubation (immediate or delayed) to NRS may be the preferred approach. The definition of “extreme prematurity” for that decision or for the decision for immediate or delayed extubation is not determined yet and is open for future debate. Furthermore, it relies not only on gestational age and birth weight but also on the severity of RDS, the individual infant condition, and the specific setup in the NICU (time of day, available personnel, etc.). In the future, new effective modes of surfactant administration should be developed that will enable the administration of surfactant without endotracheal intubation and ventilation. There are reports of less invasive surfactant administration through a feeding catheter without intubation (Kribs et al. 2007). Although not yet shown to be effective, aerosolized surfactant may become available in the future.
If NRS is to be tried, NIMV/NSIMV seems to be more beneficial than NCPAP for acute RDS and after extubation, as a “bridge” to spontaneous unsupported breathing. Effective synchronization, pressure support ventilation, and HFV via nasal ventilation (Kugelman et al. 2007; van der Hoeven et al. 1998; Hoehn and Krause 2000) may improve NRS in the future. Extubation with no NRS may be suitable for larger infants. This algorithm needs to be further supported by large, prospective studies in VLBW and ELBW infants.
Essentials to Remember
NRS is an effective treatment of premature infants with RDS.
It is safe to use a selective intubation approach in the delivery room for premature infants.
In the very preterm infants, combined therapy with surfactant and NRS may be required.
NIMV is more effective than NCPAP alone for the initial treatment of RDS, after extubation in the recovery phase of RDS, and probably for apnea of prematurity.
The definition of “extreme prematurity” that mandates intubation and surfactant and dictates immediate or delayed extubation is not determined yet and is open for future debate.
17.2 The Pediatric Patient
Federico Martinon-Torres5, 6
(5)
Pediatric Emergency, Intermediate and Critical Care Service, Department of Pediatrics, Hospital Clinico Universitario de Santiago, Santiago de Compostela, Spain
(6)
GENVIP, Instituto de Investigación Sanitaria de Santiago (IDIS), Santiago de Compostela, Spain
Educational Aims
To provide a comprehensive review of the current indications of noninvasive ventilation (NIV) in children
To detail pediatric NIV indications in either acute or chronic conditions
To establish the NIV indication according to physiopathological criteria defining the type of respiratory failure
To prepare physicians for adequate patient selection (a key factor in NIV success)
To describe the role of NIV in combination with helium–oxygen mixtures
To review and discuss NIV contraindications in children
17.2.1 Introduction
Noninvasive ventilation (NIV) in the pediatric patient is reasonably safe and efficacious. Owing to constant advances in NIV ventilators and interfaces, as well as the increasing familiarity of hospital staff with this technique, clinical use of NIV is increasing both in frequency, scope, and efficacy. However, the objective available data supporting its use is limited, the majority of our evidence being extrapolated from adult studies. Data regarding the use of NIV in infants and children are scarce, and case series constitute the majority of our knowledge in both acute and home settings. Furthermore, in many of these studies, different ages and clinical entities are mixed making it more difficult to draw concrete conclusions.
NIV should not be seen as a therapeutic “bridge” between plain oxygen therapy and invasive mechanical ventilation but as the “bridge” to recovery (or wellness) of our pediatric patient without the need of intubation and its related risks and inconveniences. However, it is important to prevent the eagerness to avoid invasive ventilation from unduly delaying endotracheal intubation in those cases where intubation really is necessary. The growing demands that noninvasive ventilation entails for hospital staff require the development of clear indications and treatment guidelines and protocols. Achieving a positive outcome with NIV is dependent on a number of factors; however, the most important of which appears to be patient selection. This chapter aims to review and establish general clinical guidelines and indications of NIV in the pediatric patient based on the existing evidence and author’s experience.
17.2.2 Indications in Acute Respiratory Failure
The indications of NIV in respiratory failure are becoming numerous and increasingly clear, leading to safer and more successful results of the technique. These indications are based on the criteria for pediatric acute respiratory failure (ARF) (Table 17.1). These can be extrapolated to establish a general classification for the different acute and chronic pathologies that can be treated with NIV (Table 17.2). However, from a practical perspective, we consider more useful to classify the indications according to the type of ARF following physiopathological criteria (Table 17.3):
Table 17.1
Criteria for respiratory failure in children
Clinical criteria | Physiological criteria |
---|---|
Weak cough, retention of respiratory secretions | Vital capacity <15 mL/kg |
Increased use of accessory muscles | Maximum inspiratory force <20 cm H2O |
High respiratory frequency (based on age) | PaCO2 >45 mmHg and pH <7.35 |
Paradoxical breathing | PaO2/FiO2 <300 mmHg |
Incompetent swallowing | SatO2 <97 % for ambient air |
Reduced activity level or diminished function |
Table 17.2
Pediatric indications of noninvasive ventilation
Acute respiratory failure (ARF) | Chronic respiratory failure (CRF) |
---|---|
A. Decompensated central nervous system (CNS) diseases | A.Respiratory sleep disorders |
1. Apneas in preterm infants | 1. Obstructive sleep apnea syndrome (OSAS) |
2.Apneas in full-term infants | 2. Primary or acquired central alveolar hypoventilation |
B. Decompensated respiration due to chest wall or spinal abnormalities | B. Neuromuscular diseases (NMDs) that affect respiratory musculature |
1. Obesity–hypoventilation syndrome | 1. Diseases of the 2nd motor neuron (e.g., spinal muscular atrophy [SMA]) |
2. Prader–Willi syndrome | 2. Diseases/damage of the phrenic nerve |
C. Upper airway diseases | 3. Myasthenia gravis/other congenital myasthenic syndromes |
1. Infectious etiology (e.g., laryngitis, epiglottitis, tracheitis) | 4. Myopathies (e.g., congenital, mitochondrial, metabolic, or inflammatory and deposition diseases) |
2. Inflammatory etiology (e.g., post-extubation subglottic edema, recurrent or spasmodic croup) | 5. Muscular dystrophies (MDs) |
D. Lung diseases | C. Upper airway diseases |
1.Asthma | 1. Tracheomalacia |
2. Bronchiolitis | 2. Down’s syndrome (prominent tongue, maxillary hypoplasia) |
3. Pneumonia | 3. Craniofacial syndromes with mandibular or midfacial hypoplasia |
4. Atelectasis | 4. Subglottic stenosis |
5. Acute pulmonary edema (APE) | 5. Neoplastic etiology (laryngeal or tracheal growths, extrinsic compression of the larynx, trachea) |
E. Other situations | D. Respiratory diseases of the lower tract or parenchyma |
1. Apneas after tonsillectomy | 1. Bronchopulmonary dysplasia (BPD) |
2. Postoperative period after corrective surgery for scoliosis | 2. Cystic fibrosis |
3. Pulmonary complications from sickle cell disease | 3. Bronchiectasis |
4. Early extubation | 4. Diffuse interstitial pulmonary diseases |
5. Sedation procedures | E. Rib cage and chest wall anomalies |
6. Palliative indication (i.e., severe respiratory failure in terminal illness) | 1. Progressive scoliosis |
2. Asphyxiating thoracic dystrophy (mild forms) | |
F. Other situations | |
1. Spina bifida (pulmonary complications, restrictive lung) | |
2. Cerebral palsy (laryngeal dystonia, restrictive lung) |
Table 17.3
Physiopathological classification of acute respiratory failure (ARF)
Type I ARF | Type II ARF |
---|---|
Acute Lung Injury (ALI) | Respiratory center |
Acute respiratory distress syndrome (ARDS) | Drugs (opiates, barbiturates, and anesthetics) |
Neonatal distress syndrome | Central congenital hypoventilation syndrome (CCHS) |
Bronchoaspiration | Upper motor neuron |
Acute bronchiolitis | Spinal column trauma |
Cardiogenic pulmonary edema | Syringomyelia |
Cystic fibrosis | Demyelinating diseases |
Pulmonary embolism (air, fat, or blood) | Tumors |
Interstitial pulmonary disease | Anterior horn neurons |
Postobstructive pulmonary edema | Poliomyelitis |
Radiation | Werdnig–Hoffmann syndrome |
Sepsis | Lower motor neuron |
Severe pneumonia (of bacterial, viral, fungal, or parasitic origin) | Post-thoracotomy lesion of the phrenic nerve |
Inhalation of poisons or toxic gases | Guillain–Barré syndrome |
Multiple transfusions | Neuromuscular junction |
Trauma (pulmonary contusion) | Botulism, multiple sclerosis (MS), and myasthenia gravis |
Antibiotics that block neuromuscular function | |
Organophosphorus compound poisoning | |
Tetanus | |
Pleura and chest wall | |
Lesions from chest wall burns with retraction | |
Massive pleural effusion and morbid obesity | |
Muscular dystrophy (MD) and pneumothorax | |
Increased airway resistance | |
Obstruction of the larynx (e.g., laryngitis, diphtheria, epiglottitis, aspiration of foreign bodies, post-extubation edema, and paralysis of the vocal chords) | |
Lower airway obstruction (e.g., emphysema, asthma, and acute bronchiolitis) |
(a)
Type I ARF. This is characterized by an imbalance between ventilation and perfusion (V/Q mismatch) without alveolar hypoventilation. It first appears as hypoxemia with no increase in arterial carbon dioxide pressure (PaCO2). Patients may exhibit condensations when examined by chest X-rays.
(b)
Type II ARF. The main feature of type II ARF is alveolar hypoventilation. It is defined by the presence of hypercapnia (PaCO2 >45 mmHg) with a normal alveolar–arterial oxygen gradient (A-a O2). Patients may present with hypoxemia due to hypoventilation. Chest X-rays do not reveal any condensations (excluding atelectases).
Based on this classification, the indications of NIV in the pediatric patient are summarized in Table 17.4. Entities such as acute bronchiolitis can be classified under each type of ARF as a function of their clinical characteristics. The use of this guide facilitates the selection of the patient, the choice of the NIV technique and interface, as well as the programming of the initial NIV settings (Fig. 17.4). Out of the absolute contraindications (see below), in our opinion, a trial of NIV should probably be offered in time to all forms of acute respiratory failure, considering that with a predominant hypercapnic failure (type II ARF), the patient will be more prone to respond, while in those patients with hypoxemic ARF (type I ARF), we should have a lower threshold for abandonment of the technique because the chance of success is lower and the risk of overuse higher.
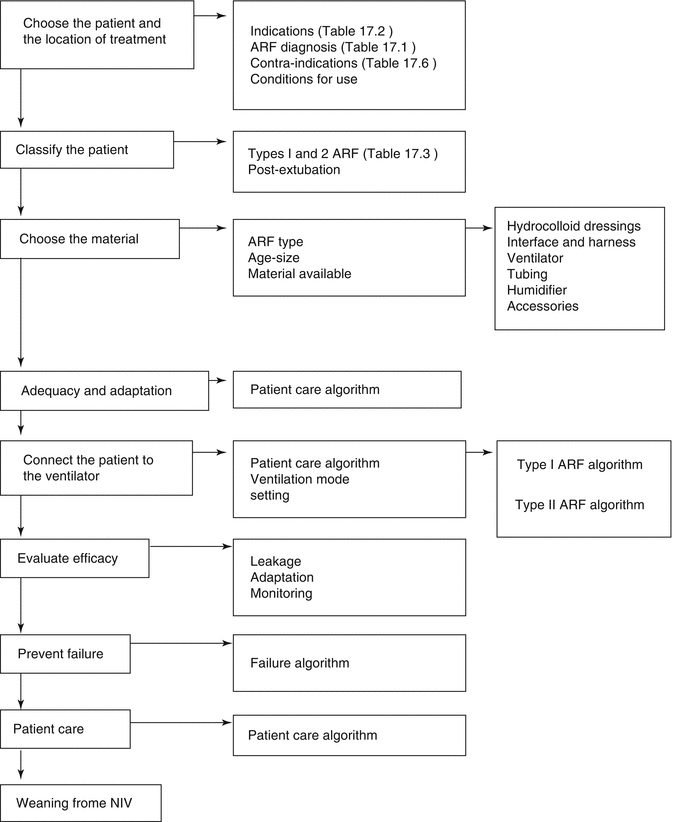
Table 17.4
Objectives of long-term home noninvasive ventilation
(a) Maintain and prolong the patient’s life |
(b) Improve the quality of life of the patient and their family |
(c) Maintain adequate (i.e., age-appropriate) growth and development of the patient |
(d) Promote lung growth and expansion, and prevent chest wall deformities |
(e) Reduce morbidity |
(f) Facilitate return of the patient into their family circle |
(g) Optimize the cost to benefits ratio of the patient’s healthcare |
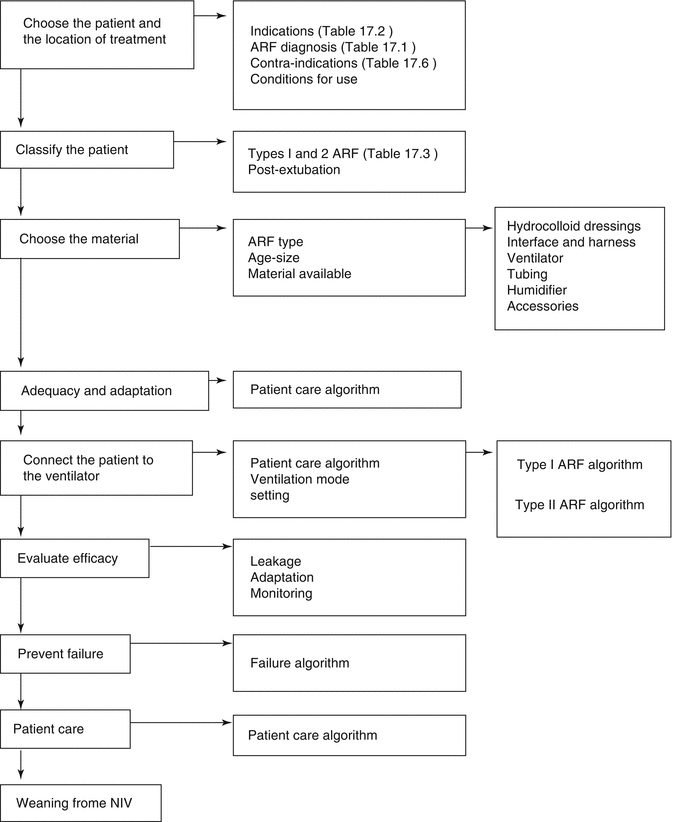
Fig. 17.4
General algorithm for noninvasive ventilation (Adapted with permission from original table in Medina et al. (2009))
In patients with acute airway obstruction, such as that which occurs in laryngitis and in certain pharyngeal–tonsillar infections, NIV can markedly reduce the muscle effort required for breathing and improve gas exchange. It must be noted that use of NIV here is not devoid of risks and should be performed in the presence of experts in managing complicated airways, with adequate material available for establishing a definitive airway and only if the patient maintains their physiological reflexes for airway protection.
For ARF related to bronchiolitis, NIV can be indicated at different moments and for different reasons. For the youngest patients, who present with apneas, CPAP tends to be indicated as in the case of apneas in preterm infants. Patients tend to respond favorably, except in the case of severe apneas and/or if the infection evolves into pneumonia. NIV then takes on the same indications and limitations described for severe cases of hypoxemia. For patients with obstructive respiratory failure, with an increase in respiratory muscle effort as well as secondary retention of CO2, NIV with two levels of pressure (bi-level positive pressure airway ventilation, or BiPAP) tends to be indicated, namely, for improving gas exchange and reducing respiratory effort. Nonetheless, a recent random study which demonstrated that use of CPAP alone was sufficient to improve hypercapnia warrants mention. The benefits of NIV employing mixtures of helium and oxygen in severely affected patients are also noteworthy (see section below). The greatest challenge in this type of pathology resides in the fact that adequate material is often difficult to find for these patients (typically, infants younger than 3 months).
Indication of NIV in pediatric patients with severe hypoxemia due to asthma remains highly controversial. The complications implied in conventional mechanical ventilation (CMV) for this pathology have led to the search for alternate ventilatory strategies. NIV has been shown to improve oxygenation in patients during severe asthmatic attacks. NIV treatment of children with this pathology has scarcely been studied, and the few existing reports deal with small patient populations: 33 (Mayordomo-Colunga), five (Carroll), and three (Akingbola). Nonetheless, in the study by Mayordomo-Colunga, NIV only failed in one case. This is accomplished through a minor adjustment of the V/Q mismatch, whereby using a PEEP which does not exceed 80–90 % of the auto-PEEP in combination with an inspiratory positive airway pressure (IPAP) or a pressure support (PS) that lowers the level of respiratory effort required to maintain an adequate flow volume for gas exchange. The greatest challenge in clinical practice is to ensure that the patient couples well to the ventilator, without any agitation; this often requires light sedation. However, in respiratory failure with hypercapnia due to an asthmatic attack, NIV has not proven superior to CMV nor has it been shown to lead to lower number of required intubations. Early administration of NIV (i.e., before total respiratory failure) and use of ventilators that are very sensitive to the respiratory efforts of the patient and that consequently enable good synchronization may be important factors in the success of the treatment.
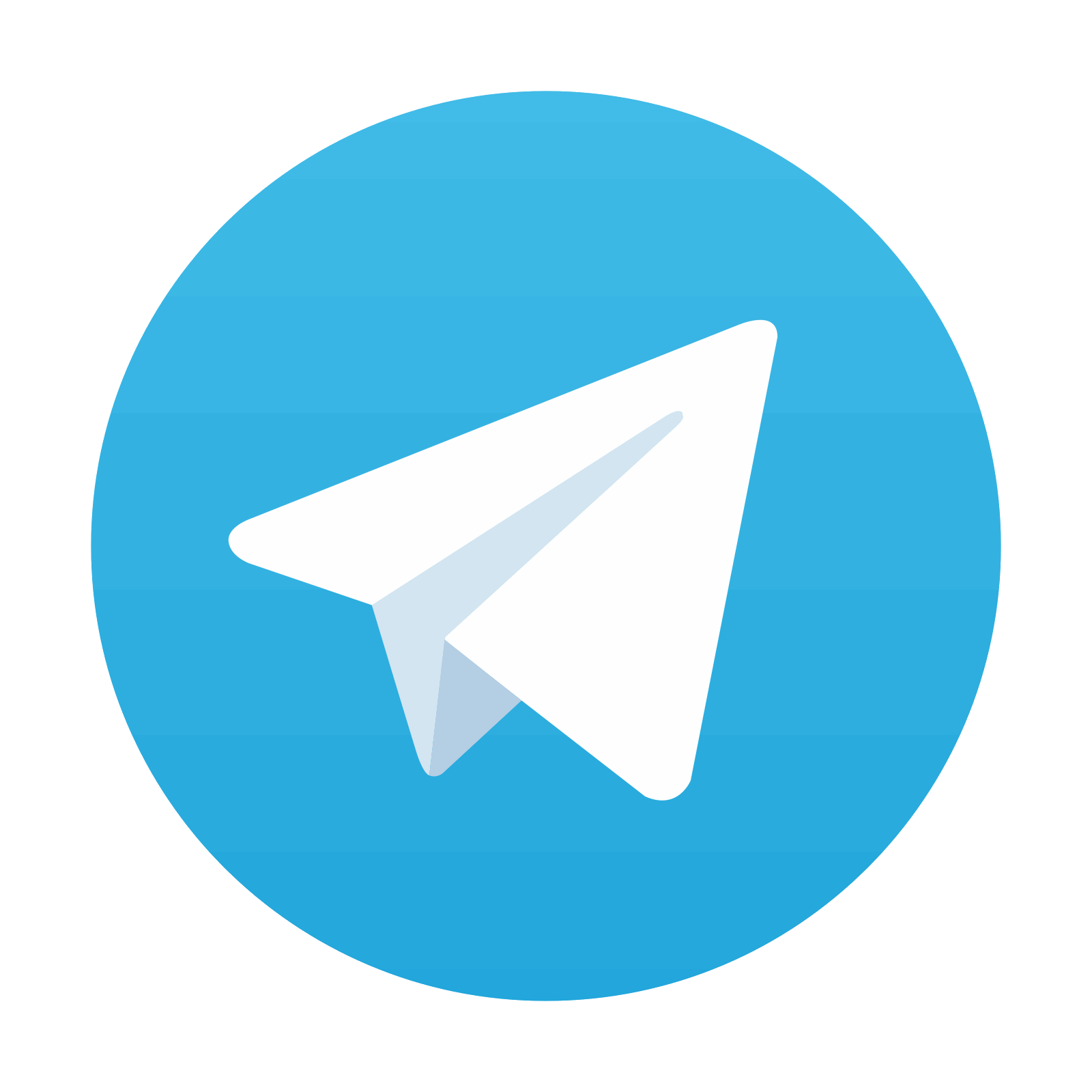
Stay updated, free articles. Join our Telegram channel

Full access? Get Clinical Tree
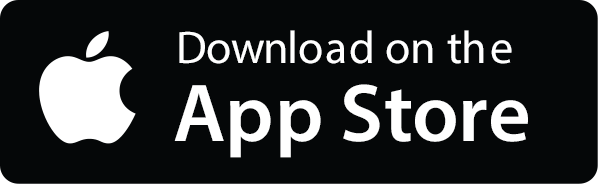
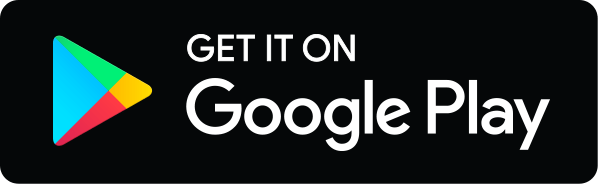