and Andreas Schulze4
(4)
Division of Neonatology, Department of Pediatrics, University of Miami Miller School of Medicine, Miami, FL, USA
18.1.1 Introduction
The spectrum and severity of respiratory illness in the newly born has been changing over the past three decades with the introduction of antenatal steroids, improved management of the fetus during preterm labor and birth, and postnatal exogenous surfactant administration. Early pulmonary parenchymal failure requiring invasive ventilation occurs less frequently. Moderately preterm infants who received invasive mechanical ventilation in the past can often be managed nowadays with noninvasive respiratory support. There is, however, a “new generation” of surviving extremely small infants who depend on early initiation of invasive ventilation and who stay on the ventilator for extended periods of time with substantial risks for ventilator-induced lung injury. Such infants often require invasive ventilation for reasons other than surfactant deficiency. These comprise, among others, respiratory pump failure and intermittent failure of the immature respiratory rhythm generator and control of breathing. Diverse invasive mechanical ventilation strategies and modalities have been developed to better address these problems; however, evidence of their effectiveness is still scarce. Therefore, the clinician’s expertise in some of the basic invasive ventilation techniques and devices probably remains the key determinant of a successful application.
18.1.2 Major Indications for Invasive Assisted Ventilation
18.1.2.1 Critical Upper Airway Obstruction
Severe upper airway obstruction with or without other accompanying respiratory disorder may merit endotracheal intubation and assisted ventilation. An orally or nasally inserted artificial airway, i.e., endotracheal tube or laryngeal mask bypassing the stenosis, may already fully relieve the respiratory distress in some of these cases. It is sometimes impossible to place an artificial airway with the use of a laryngoscope. A thin flexible fiberscope is an alternative to visualize and enter the larynx and the trachea. It can subsequently serve as a guide wire to the endotracheal tube in such situation. Major obstruction can occur at all levels of the upper airway in the newborn. Bilateral choanal atresia is a rare condition with bony structures occluding the posterior nasal passage in about 90 % of cases. Other causes of obstruction at the supraglottic level include teratomas, anterior meningoencephaloceles, hemangiomas, hygromas, and variants of the Pierre Robin sequence or Treacher Collins syndromes where mandibular hypoplasia, cleft palate, and glossoptosis can be present to a varying degree. Some types of congenital intrinsic obstruction of the larynx and/or the trachea preclude an endotracheal tube placement and may require a tracheostomy.
18.1.2.2 Respiratory Pump Failure
The respiratory pump consists of respiratory musculature and the thoracic cage. Both structures undergo major developmental changes during late fetal and early neonatal life. Immaturity of the respiratory pump makes several components of this system prone to failure. Failure will occur when the load exceeds pump strength. The load can be measured as work of breathing. Lung compliance and airway resistance are major determinants of this load. Inspiratory rib cage inward distortion is characteristic of the preterm newborn in whom the thoracic skeleton is floppy and intercostal muscle tone is low. Such chest wall distortion requires additional muscle work because the diaphragmatic volume displacement during inspiration does not fully translate into lung expansion and part of the diaphragmatic movement is wasted into rib cage distortion. Respiratory pump fatigue is defined as decreasing minute ventilation (tidal volume and/or respiratory rate) during continued respiratory load. Failure can occur at three different sides: central fatigue (reduced phrenic nerve output), neuromuscular transmission failure (decreasing EMG activity at normal or increased phrenic nerve activity), and muscle contractile failure (decreased diaphragmatic pressure output despite increased EMG and phrenic nerve activity). Several indexes (tension-time index, maximum inspiratory diaphragmatic force, and others) have been proposed as means to recognize impending respiratory muscle failure and the need for assisted ventilation in the newborn. Such measures could also be helpful for predicting failure of weaning from assisted ventilation and extubation. However, evidence of the clinical utility of these indexes is still pending. Clinical judgement by an experienced neonatologist therefore currently still appears the most valuable tool to recognize impending fatigue as a reason to initiate assisted ventilation.
18.1.2.3 Central Control of Breathing Failure
The control of breathing system involves neuronal networks in the brainstem with populations of cells that exhibit rhythmic intrinsic bursting activity. They are but one component of a neural feedback with the overall objective to maintain blood gas homeostasis. Peripheral oxygen sensors, central carbon dioxide sensors, and intrapulmonary mechanoreceptors feed signals into the circuit. Commonly, this feedback provides for an overall stable breathing pattern. The majority of this system’s components undergo major maturational changes in the fetus. Respiratory instability in the immature infant may present with a wide range of breathing patterns such as periodic breathing, hypopnea, and recurrent apnea which may be unresponsive to medication and require mechanical ventilatory assist. The newborn is largely unable to mount sustained hyperventilation during hypoxia which also contributes to a propensity of the central control to fail.
18.1.2.4 Intrapulmonary Conditions Causing Respiratory Gas Exchange Failure
In the newborn, pulmonary parenchymal disease is often caused by several combined pathophysiological conditions that ultimately may lead to respiratory gas exchange failure. Among others, these are structural immaturity, surfactant deficiency, shunts across a patent ductus arteriosus, inflammatory changes and pneumonia, intrapulmonary hemorrhage, and malformations.
There are three major causes of arterial hypoxemia:
Right-to-left shunt, either intrapulmonary (mainly caused by atelectasis) or extrapulmonary (at the ductal or atrial level)
Ventilation-to-perfusion mismatch (particularly in infants with chronic lung disease)
Hypoventilation
Diffusion impairment
These conditions typically respond with an improvement in oxygenation when the fraction of inspired oxygen is increased. This, however, does not occur if there is a right-to-left shunt of more than about 30 % of cardiac output. Diffusion impairment is regarded to be a very uncommon cause of hypoxemia in the newborn.
A critical impairment of pulmonary carbon dioxide removal is usually caused by alveolar hypoventilation but ventilation-to-perfusion mismatch can also contribute to the problem.
18.1.3 Assessment of Need for Mechanical Ventilation
The decision to initiate invasive mechanical ventilation in the newborn is an important step because of the short- and long-term complications associated with this mode of therapy. This is especially relevant in the smaller preterm infants. There is no standard approach and considerable variation exists between different centers in the criterion to initiate mechanical ventilation. This decision must take into consideration the gestational age of the infant, the degree of respiratory failure, and the disease process that is producing the respiratory failure together with the alternatives available to support the infant’s respiratory function. It is also important to take into consideration the experience of the team and the expected outcome of infants exposed to mechanical ventilation in each institution. While in units with vast experience and good outcomes, ventilation may be used more liberally; in units with limited experience, safer alternatives should be considered before embarking in invasive ventilation.
The indication of invasive ventilation is usually based on the clinical assessment of the infant combined with the evaluation of arterial blood gas levels. In the smaller infants ventilation is often begun in the delivery room because of respiratory depression and bradycardia or because of the need to administer surfactant. Subsequently many preterm infants are ventilated because of recurrent episodes of apnea and hypoxemia that require mask ventilation to recover. In other situations ventilation is initiated when levels of P aCO2 rise rapidly. Although there is no specific level of P aCO2, most clinicians consider mechanical ventilation when levels rise acutely above 55–65 mmHg and the pH is below 7.25–7.20.
The other parameter considered for initiation of ventilation is hypoxemia that does not improve with continuous positive airway pressure. Again, there are no set levels of P aO2 or FiO2 to start ventilation, but in preterm infants with hyaline membrane disease (HMD), the decision to insert an endotracheal tube and initiate mechanical ventilation is frequently linked to the decision to administer exogenous surfactant. The indication varies between institutions, but there is good evidence that early administration of surfactant results in better outcome and therefore in an infant with HMD surfactant is usually given when the inspired oxygen concentration required to keep acceptable P aO2 or oxygen saturation levels increases above 30–40 %. Ventilation may be started earlier if the infant has hypercapnia or clinical signs of significant distress and impending failure. In many centers surfactant is given as prophylaxis to all infants below a certain gestational age, usually 26–28 weeks, and if the infant has good respiratory effort is then extubated to nasal CPAP.
In full-term infants, the indication for mechanical ventilation can be more conservative since these infants have better capacity to cope with increased work of breathing. It also varies depending on the underlying cause of the respiratory failure. For example, in an infant with respiratory failure due to a congenital diaphragmatic hernia, ventilation is usually started immediately after birth, while in an infant with a congenital pneumonia or meconium aspiration, a more conservative approach can be taken and ventilation is usually not started until there is evidence of rising P aCO2 and hypoxemia, requiring increasing inspired oxygen concentrations up to 40–60 %. Once again, the clinical evaluation of the infant is important in deciding whether invasive ventilation should be started or not.
It is important to note that the use of positive pressure ventilation seldom results in improved lung function and in fact frequently can produce further deterioration due to the negative effects of high inspired oxygen concentrations, pulmonary infection, and excessive airway pressure and overdistention of the lung. The effectiveness of mechanical ventilation is primarily due to the support of the infant’s failing respiratory pump by the intermittent positive pressure generated by the ventilator and reduction in the work of breathing. An exception to this is the infant with HMD where the positive airway pressure can produce recruitment of distal air spaces with improvement in ventilation perfusion matching and gas exchange.
18.1.4 Ventilation Targets
18.1.4.1 Oxygen
Because of the potential side effects of oxygen therapy, the oxygenation target is extremely important especially in the small preterm infant. Despite this, the available information on the optimal oxygen targets is limited. Although the negative effects of extreme hyperoxia and hypoxia are well established, there is little data on what the range for P aO2 or oxygen saturation should be for infants of different gestational or postnatal ages. In the small preterm infant, there is evidence that prolonged exposure to P aO2 over 70 mmHg is associated with increased risk of retinopathy of prematurity (ROP) (Bancalari et al. 1987; Flynn et al. 1992). The same has been shown for exposure to oxygen saturations over 95 % (Tin et al. 2001). For this reason it is recommended to keep P aO2 and saturations below these levels. This does not only reduce the risk of ROP, but limiting the amount of oxygen in the inspired gas can also reduce pulmonary damage and the incidence of BPD (Askie et al. 2003; Phelps et al. 2000). While this is less critical in the full-term infant, there is compelling evidence that in these infants, the exposure to excessive oxygen concentrations can also be associated with oxidative damage (Vento et al. 2003). Less clear is what the lower limit of oxygenation should be, so until evidence from ongoing clinical trials becomes available, it is recommended to keep the lower levels of P aO2 above 50 mmHg and pulse oximetry saturations above 85–88 %. Exceptions to these limits are infants with certain congenital heart diseases where lower values may be acceptable or even desirable as in cases of single-ventricle physiology where low alveolar PO2 may be used as a way of limiting pulmonary blood flow and preserving systemic blood flow.
18.1.4.2 Carbon Dioxide, pH
In general terms, the aim during mechanical ventilation is to normalize ventilation and gas exchange, and therefore the clinician tries to keep P aCO2 between 40 and 50 mmHg. While this is easily achievable in infants with relatively normal lung function, it becomes more difficult as the alteration in lung function is more severe and this requires high ventilator settings. In order to avoid these potentially damaging aggressive settings, the option of allowing higher P aCO2 levels has been used both in adults and infants as long as the pH is >7.25–7.30. This strategy has been labeled “permissive hypercapnia.” This modality has also been investigated in preterm infants, and although it was suggested that it may shorten the duration of ventilation, there was no clear evidence that led to an improved long-term pulmonary outcome (Carlo et al. 2002; Mariani et al. 1999). In fact one of the most recent trials suggested that infants with higher P aCO2 could have higher mortality and worse neurological outcomes (Thome et al. 2006). These results have discouraged clinicians to use high P aCO2 levels in small preterm infants. However, in order to wean infants with chronic lung disease, it is necessary to tolerate higher P aCO2 levels, sometimes in the 70s or 80s before and immediately after extubation. These patients frequently have a compensatory metabolic alkalosis that results in a relatively normal pH.
Some years ago, many infants, specifically those with pulmonary hypertension, were managed with hyperventilation and low P aCO2 levels. This approach has been abandoned because it produces cerebral vasoconstriction and may result in worse neurological outcome (O’Shea and Dammann 2000). Because to achieve hyperventilation it is necessary to use aggressive ventilator settings, it is also associated with increased lung damage and higher incidence of BPD (Kraybill et al. 1989). For these reasons hyperventilation has been abandoned and should be avoided in the newborn.
18.1.4.3 Airway Pressures, Lung Volume
Ideally the level of positive end-expiratory pressure (PEEP) should be based on measurements of lung volume. While there are methods to measure FRC in small infants, these measurements are not done in routine clinical practice, and therefore the selection of PEEP level is based on the degree of lung disease, arterial oxygenation, and radiographic appearance of the lungs. Although there is no clear data in the neonate, most clinicians have adopted levels of PEEP that usually range from 3 to 8 cm H2O. The higher levels are used in infants with more severe lung disease where these pressures are necessary to stabilize the distal airways and preserve FRC and oxygenation. The concerns with higher levels of PEEP are overdistention of the lungs with the risk of alveolar rupture, and decrease in venous return and cardiac output with an increase in pulmonary vascular resistance. Excessive PEEP levels can also result in lower lung compliance and hypoventilation (Alegria et al. 2006; Dimitriou et al. 1999). Work in experimental animals has also shown that high levels of PEEP can increase capillary permeability and increase neutrophil migration into the lung (Naik et al. 2001).
The selection of peak inspiratory pressure (PIP) is simpler now that most ventilators allow the measurement of flow and tidal volume. In general terms one selects the PIP that results in a tidal volume of 3–5 ml/kg body weight. The level of PIP is also adjusted depending on P aO2 and P aCO2 levels. Presently most infants are ventilated with patient-triggered ventilators, and most of the tidal volume is generated by the infant while the PIP is used only to support their effort and achieve the desired ventilation and oxygenation. With this strategy most infants can be ventilated with PIP levels below 20 cm H2O, and when the lung disease is less severe, pressures of 6–12 cm H2O above PEEP are usually sufficient to maintain ventilation. The level of pressure required depends on the severity of the derangement in lung compliance and resistance and the spontaneous effort that the infant is capable of generating at each stage of their disease process. Because higher airway pressures are associated with increased risk of acute and long-term lung damage, the PIP target should always be the lowest level required to keep adequate tidal volumes and minute ventilation.
18.1.5 Short- and Long-Term Outcome
The acute complications and survival of infants who receive mechanical ventilation are closely related to the criteria and indications for initiating ventilatory support, to the underlying condition of the infant, and to the experience and skills of the personnel providing this therapy. The more liberal the indication for IPPV, the better the results should be. In preterm infants the gestational age and associated conditions are the most important determinants of outcome.
While the survival of extreme premature infants has increased in the last two decades, the incidence of complications such as BPD has not changed or may even be increasing. This should be expected because the smallest new survivors are at increased risk for the major complications associated with extreme prematurity (St John and Carlo 2003). This may also be in part due to the less frequent use of postnatal steroids since the late 1990s.
In a retrospective analysis of prospectively collected data from the NICHD Neonatal Research Network, the overall survival of 4,752 ventilated infants with birth weight of 501–1,000 g was 74 % (Walsh et al. 2005). The median duration of ventilation was 18 days (23 days in survivors and 6 in non-survivors). Twenty-five percent of the infants remained on mechanical ventilation by 36 days and 7 % were ventilated for more than 60 days. Thirty-nine percent of the surviving infants developed chronic lung disease.
The incidence of pneumothorax associated with mechanical ventilation has decreased from 10 to 15 % in the pre-surfactant era to around 5 % after the introduction of postnatal surfactant replacement. In contrast, the incidence of pulmonary hemorrhage has remained relatively constant or even increased in the smallest infants in whom the incidence can be as high as 10–15 % (St John and Carlo 2003). This complication has been associated with the use of exogenous surfactant and with the persistence of the ductus arteriosus and increased pulmonary blood flow.
Ventilator-associated pneumonia is a common complication of mechanical ventilation in any age group, and it is likely to occur even more frequently in the immunocompromised premature infant. Unfortunately, it is not commonly diagnosed in this population because of the lack of specific criteria and the difficulty in differentiating a pneumonia from other acute and chronic pulmonary pathologies that occur frequently in infants requiring prolonged mechanical ventilation (Cordero et al. 2002).
The long-term outcome of infants treated with IPPV is more related to the patient characteristics than to the use of mechanical ventilation. Bronchopulmonary dysplasia is the most common long-term pulmonary sequela associated with mechanical ventilation, and its incidence and severity are mainly associated with the gestational age and severity of the initial respiratory failure (See Chronic Respiratory Failure in Neonates).
There is evidence that even in premature infants who do not develop BPD, there is an increased risk of lower respiratory tract infections early in life, and there are pulmonary function abnormalities reflecting obstructive airway disease that may persist later in life (Kitchen et al. 1992). It is not clear whether these abnormalities are mainly related to the premature birth or to the use of mechanical ventilation (Chan et al. 1989). There is also a reported increased incidence of asthma in preterm survivors when compared to controls (Halvorsen et al. 2004). Infants with BPD may have severe reduction in airflow later in life but this airway obstruction varied little with the severity of BPD and was also present in ventilated infants who did not develop BPD (Doyle 2006; Doyle et al. 2006).
Mechanical ventilation has also been associated with impaired neurodevelopmental outcome. Thirty-one percent of 2,755 infants 500–1,000 g exposed to mechanical ventilation and tested for neurodevelopmental outcome had an MDI < 70, and 22 % had a PDI < 70. Nine percent of the tested infants had moderate to severe cerebral palsy. These impairments were more common in infants who remained on mechanical ventilation past 28 days. Additional risk factors for poor neurodevelopmental outcome were non-white race, male gender, grade III–IV IVH, and low maternal education level (Walsh et al. 2005). It is not clear whether in infants with chronic lung disease the poor development outcome is related to the respiratory disease or to the multiple factors that predispose these infants to BPD (Laughon et al. 2009).
Infants with BPD are at a significantly higher risk for developing cerebral palsy (Short et al. 2003; Skidmore et al. 1990; Vohr et al. 1991, 2005). This may be in part due to the use of postnatal steroids, a therapy that is known to be correlated with higher incidence of CP (O’Shea et al. 1999; Shinwell et al. 2000; Yeh et al. 1998). Infants with BPD also have lower developmental scores and poorer school performance than survivors without chronic lung disease (Short et al. 2003; Gray et al. 2004; Hughes et al. 1999; O’Shea et al. 1996; Robertson et al. 1992; Taylor et al. 1998).
18.1.6 Contraindications of Invasive Mechanical Ventilation
Mechanical ventilation is usually considered a life-saving therapy that is instituted in infants with severe respiratory failure, most of whom would not survive without this therapy. Therefore, there are no specific contraindications to initiate mechanical ventilation when this is properly indicated. There are some situations when the indication may be questionable because of the poor expected outcome such as in infants at the border of viability, after severe perinatal asphyxia, or with life-threatening congenital malformations. A similar situation may occur when the risks of invasive ventilation are so high that they outweigh the possible benefits of this therapy.
Essentials to Remember
The indication of mechanical ventilation should be determined by the risk benefit analysis in each infant.
The effectiveness and safety of mechanical ventilation are related to the expertise of the clinical team.
Mechanical ventilation supplements or replaces the respiratory pump but rarely improves lung function. On the contrary it can produce severe lung injury.
The levels of pressures used during IPPV are determined by the severity of the lung disease and are adjusted to generate adequate tidal volumes and oxygenation.
The short- and long-term outcomes in infants who receive mechanical ventilation are determined by the gestational age and the underlying condition of the infants and the experience and expertise of the clinical team.
18.2 The Pediatric Patient
Kyle J. Rehder5 , David A. Turner5 and Ira M. Cheifetz6
(5)
Division Chief, Pediatric Critical Care Medicine, Pediatric Intensive Care Unit, Pediatric Respiratory Care and ECMO, Duke Children’s Hospital, Box 3046, Durham, NC 27710, USA
(6)
Division of Pediatric Critical Care Medicine, Department of Pediatrics, Duke Children’s Hospital, DUMC Box 3046, Erwin Rd, Durham, NC 27710, USA
Educational Aims
Understand the primary indications for the initiation of invasive ventilation.
Review the relationship between a child’s underlying physiologic state and disease process as they relate to the need for mechanical ventilation.
Review the causes of impaired gas exchange.
Understand the impact of neuromuscular weakness on a patient’s respiratory status.
Review the Glasgow Coma Scale score.
Recognize that neurologic dysfunction is an important indication for airway protection.
Understand the cardiopulmonary interactions created by positive pressure ventilation.
Learn the importance of a global assessment in the evaluation of the need for invasive ventilation.
Understand the consequences and potential complications related to invasive ventilation.
18.2.1 Introduction
Acute respiratory failure remains the leading diagnosis for children admitted to intensive care units (ICUs) and accounts for a significant burden of mortality and morbidity in the pediatric population. Invasive mechanical ventilation, or the use of an advanced airway to provide life support, is paramount in the care of children with lung injury as well as many other disease processes. Furthermore, duration of mechanical ventilation is a major determinant of both ICU cost and length of stay (Cheifetz 2003).
Optimizing care of ventilated children provides a host of challenges. A crucial problematic issue is the management of patients over a wide range of sizes and developmental stages. Not only are pediatric airways of different diameters and lengths, but they also change dramatically as they develop: the tongue becomes relatively smaller, the larynx becomes less anterior and moves caudad, and the subglottic space becomes less cone shaped and more cylindrical (Cote et al. 2001). As children grow, they have widely varying baseline respiratory rates, tidal volumes, and metabolic demands.
As patients change in size, the equipment needed for mechanical ventilation must adapt. Ventilators must be able to effectively deliver a wide range of tidal volumes while being sufficiently sensitive to appropriately monitor small flow rates and pressure changes. The use of equipment that is not designed for pediatric patients may lead to the delivery of unsafe tidal volumes and/or pressures or the inability to maintain appropriate ventilation targets.
Finally, children are affected by different disease processes than either adults or premature infants, yet few studies exist that evaluate ventilation strategies for the pediatric population. Much of accepted clinical practice has been derived from studies in adults, neonates, or animals. This often forces the pediatric provider to adopt ventilatory strategies which were tested on a population with different disease states and potentially dissimilar responses to treatment.
Prior to initiating invasive mechanical ventilation in the pediatric patient, the bedside clinician must address each of these questions:
1.
Do I have the support of someone who is competent in the management of pediatric airways?
2.
Do I have the proper equipment not only to secure the airway but to ventilate this child in a safe manner?
3.
Do I have the knowledge and experience to appropriately ventilate this patient’s specific disease process(es)?
Children requiring mechanical ventilation have benefited greatly from advances in both ventilation techniques and ventilator technology. When facing a critically ill child, it is imperative for front-line, noncritical care medical providers to use resources available to them, such as on-call anesthesiologists, intensivists, and referral to tertiary care centers.
18.2.2 Primary Indications for Assisted Ventilation
The need for invasive mechanical ventilation may arise from a wide range of disease processes. In children, specifically, the impact of any given disease depends not only on the pathologic process but also on the physiologic state of the child. Differing degrees of physiologic reserve exist as children progress through the normal development process, and significant variability exists between the response to illness and injury between a young infant and an adult-sized adolescent. An understanding of this normal physiologic development is crucial in the setting of critical illness when determinations are being made regarding the potential need for invasive mechanical ventilation. For example, a viral respiratory infection that may be only a minor annoyance in an otherwise healthy adult can have devastating consequences and complications in a young infant. There are a range of circumstances in which substantial variability exists in both presentation and progression of disease depending on the age and physiologic state of the child.
A wide spectrum of clinical situations may require invasive mechanical ventilation in children, including both primary respiratory failure of various etiologies and organ dysfunction not directly related to the respiratory system. Invasive ventilation may be necessary for airway protection in the setting of neurologic dysfunction, including trauma, seizures, neuromuscular weakness, and/or other causes of altered mental status. Similarly, dysfunction of the cardiovascular system, secondary to congenital disease or an acute acquired process (e.g., myocarditis), may also necessitate mechanical ventilation to decrease metabolic demands. It is important to complete a thorough global assessment, including a comprehensive history and physical examination that includes all major organ systems, as one works to determine when invasive mechanical ventilation is indicated. In this section, we will examine four major categories of illness that may require invasive ventilation: unstable airways, impaired gas exchange, respiratory muscle insufficiency, and need to reduce mechanical ventilatory load. While this list is not all-inclusive, it does provide the majority of reasons for which pediatric patients require assisted ventilation.
18.2.2.1 Unstable Airways
18.2.2.1.1 Anatomic Airway Integrity
Airway integrity can be challenged by either anatomical or neurological insults. From an anatomical view, the upper airway includes the space from the lips and nares to the subglottic region, including the tongue, teeth, nasal turbinates/septum, adenoids, tonsils, pharynx, and larynx. Obstruction to airflow may occur at any of these levels, necessitating intervention (Table 18.1). Clinically, impending respiratory failure may present as a marked increase in the patient’s work of breathing or as inadequate gas exchange (usually hypoxia).
Table 18.1
Causes of upper airway obstruction
Infectious/inflammatory |
Tracheobronchitis (croup) |
Bacterial tracheitis |
Epiglottitis |
Peritonsillar abscess |
Retropharyngeal abscess |
Anaphylaxis |
Angioedema |
Trauma |
Facial or neck trauma |
Facial or inhalational burns |
Foreign body |
Congenital/syndromic |
Macroglossia (trisomy 21, Beckwith-Wiedemann syndrome, etc.) |
Micrognathia (Pierre Robin sequence, etc.) |
Midface hypoplasia (Crouzon’s Syndrome, etc.) |
Tracheo-laryngomalacia |
Subglottic stenosis or obstruction |
Vocal cord paralysis or malformations |
Anatomic |
Cystic hygroma |
Branchial cleft cyst |
Thyroglossal duct cyst |
Vascular ring |
Intrathoracic mass or tumor |
In the pediatric population, the most common causes of upper airway obstruction are infectious, including tracheobronchitis (croup), bacterial tracheitis, and retropharyngeal abscess (Leung et al. 2004; Skolnik 1989). However, only a small percentage of children with these diseases will progress to the need for an advanced airway. Epiglottitis can also lead to an extremely tenuous airway but fortunately has been almost eliminated in the developed world following universal administration of the haemophilus influenzae type b (Hib) vaccine (Leung et al. 2004).
Congenital airway malformations such as micrognathia, macroglossia, and tracheo-laryngomalacia are also significant causes of upper airway obstruction, particularly in neonates and infants (Lahiri 1996; Lerner and Perez Fontan 1998). A variety of genetic and metabolic syndromes are associated with upper airway obstruction, including trisomy 21, Crouzon’s Syndrome, Pierre Robin sequence, mucopolysaccharidoses, and some glycogen storage disorders. The majority of cases of tracheo-laryngomalacia will not require invasive mechanical ventilation, as malacia often improves as the child’s airway grows and muscle tone improves. However, with severe fixed upper airway obstruction, providing a secure airway and mechanical ventilation may be necessary to bridge patients to surgical correction or amelioration (i.e., tracheostomy).
Trauma to the oral cavity, mandible, or maxilla may also create an unstable airway that requires early, or even immediate, intervention. Similarly, inhalational burns and facial burns may have rapid, massive swelling that may compromise a patient’s airway. It is generally recommended that these burn patients be intubated on presentation to protect the airway (Cochran 2009). Similarly, it is generally recommended to establish an advanced airway early in the course of anaphylaxis, angioedema, or any other process that involves significant (or potentially significant) swelling of the airway.
Patients with epiglottitis, congenital airway malformations, severe facial trauma, and airway or facial burns must be considered high-risk airways. Intubation should be undertaken by providers experienced with difficult airways and often with the support from fiber-optic technology. Even with supportive technology, it may not be possible to visualize such an airway, and advanced techniques may be required to secure the airway, including laryngeal mask airway (LMA), blind intubation, or emergency cricothyroidotomy or tracheostomy.
While tracheal tubes stent the airway from the lips to the subglottic space, mechanical ventilation may also be necessary for some anatomical problems of the lower airways or thorax. Flail chest represents an injury to the chest wall that requires positive pressure ventilation to inflate the ipsilateral lung. Congenital diaphragmatic hernia requires early intubation to minimize air accumulation in the intrathoracic stomach and intestinal tract. Finally, severe bronchomalacia may require positive pressure to maintain patent lower airways.
Another important unstable airway situation is the patient with a large anterior mediastinal mass. These patients may require intubation for respiratory distress secondary to tracheal and/or bronchial compression, or electively for patient transport or diagnostic procedures. However, management of the airway in the patient with an anterior mediastinal mass should be undertaken with extreme care. Airway compression may acutely worsen when the patient is placed in the supine position. In addition, administration of even small amounts of sedation/analgesia often relaxes native airway tone and may precipitate acute (and sometime irreversible) cardiopulmonary collapse. For these reasons, patients with anterior mediastinal masses should be intubated with extreme caution, and potential considerations include performing this procedure in the operating room, preserving spontaneous breathing, using fiber-optic techniques, and maintaining a sitting position.
18.2.2.1.2 Neurologic Dysfunction
Neurologic dysfunction secondary to trauma, seizures, metabolic disease, or toxic ingestion may lead to loss of airway protective reflexes. Invasive mechanical ventilation is necessary in these circumstances to not only maintain gas exchange but to also prevent aspiration and allow for necessary diagnostic and therapeutic interventions.
Depending on the age of the patient, assessment of neurologic dysfunction in pediatric patients and determination of the optimal timing of intubation and initiation of mechanical ventilation may be quite challenging. Attention should be paid not only to airway reflexes but also to mental status and global neuromuscular strength and tone. As neurologic dysfunction progresses, it is preferable to address potential airway concerns early, before respiratory deterioration occurs. This proactive approach allows for a thorough pre-intubation assessment and the carefully controlled management and establishment of a secure airway.
18.2.2.1.2.1 Seizures
In the setting of seizures and status epilepticus, respiratory insufficiency and inadequate airway protection may be secondary to the convulsive activity and/or treatment of the seizure(s). Regardless of the etiology, as many as 30 % of patients with refractory status epilepticus require intubation and mechanical ventilation, making it important to plan for this possibility as seizure treatment is initiated (Lewena et al. 2009). One special consideration in the setting of seizures includes the impact of neuromuscular blockade on monitoring for further seizure activity. In this setting, intubation without neuromuscular blockade or the use of a short-acting neuromuscular blocker (e.g., rocuronium) should be considered so as to avoid masking further/ongoing seizure activity. If more prolonged neuromuscular blockade is necessary in these circumstances, electroencephalographic monitoring should be initiated.
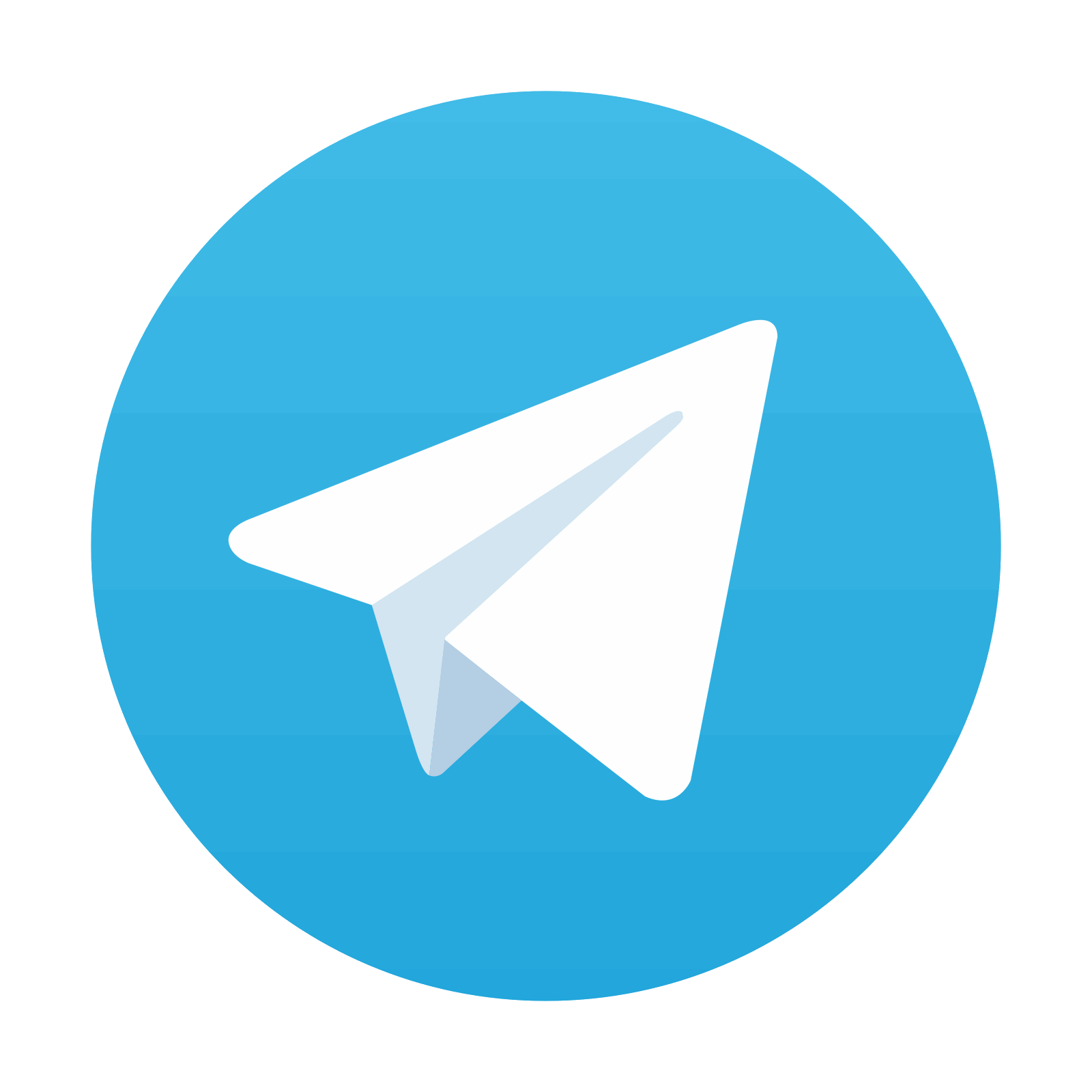
Stay updated, free articles. Join our Telegram channel

Full access? Get Clinical Tree
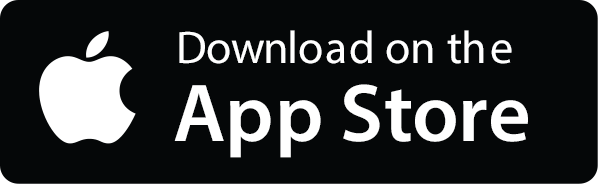
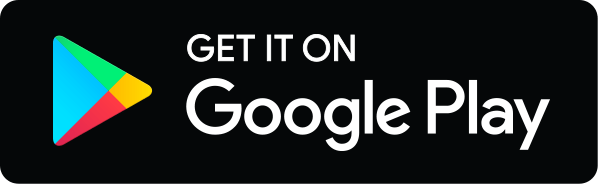
