Objective
This study aimed at determining the relationship between fetal chromosomal disorders (CDs), including trisomy 21 (T21), and on first- and second-trimester maternal blood plasma, to identify the time-course metabolic adaptations to the conditions and the possible new plasma biomarkers. Furthermore, a definition of a joint circulatory (plasma) and excretory (urine) metabolic description of second-trimester CDs was sought.
Study Design
Plasma was obtained for 119 pregnant women: 74 controls and 45 CD cases, including 22 T21 cases. Plasma and lipid extracts (for T21 only) were analyzed by nuclear magnetic resonance spectroscopy, and data were handled by variable selection and multivariate analysis. Correlation analysis was used on a concatenated plasma/urine matrix descriptive of second-trimester CD, based on previously obtained urine data.
Results
CD cases were accompanied by enhanced lipid β-oxidation (increased ketone bodies) and underutilization of glucose, pyruvate, and citrate. Lower circulating high-density lipoprotein levels were noted, along with changes in the proline and methanol in the first trimester, and also the urea, creatinine, acetate, and low-density lipoprotein plus very low-density lipoprotein in the second trimester and the different urea and creatinine levels, suggesting fetal renal dysfunction. In terms of plasma composition, T21 cases were indistinguishable from other CDs in the first trimester, whereas in the second trimester, increased methanol and albumin may be T21 specific. Furthermore, first-trimester lipid extracts of T21 showed decreased levels of 18:2 fatty acids, whereas in the second trimester, lower levels of 20:4 and 22:6 fatty acids were noted, possibly indicative of inflammation mechanisms. In both trimesters, high classification rates for CDs (88-89%) and T21 (85-92%) generally relied on variable selection of nuclear magnetic resonance data. Plasma/urine correlations confirmed most metabolic deviations and unveiled possible new ones regarding low-density lipoprotein plus very low-density lipoprotein, sugar, and gut-microflora metabolisms.
Conclusion
This work partially confirmed previously reported data on first-trimester T21 and provided additional information on time-course metabolic changes accompanying CD and T21, in particular regarding plasma lipid composition. These results demonstrate the potential of plasma metabolomics in monitoring and characterizing CD cases; however, validation in larger cohorts is desirable.
The use of metabolomics to unveil metabolic biomarkers of disease has gained increasing recognition in the development of new biomarkers and improved clinical strategies. In prenatal health, metabolomic studies have investigated changes in amniotic fluid, placental extracts, umbilical cord blood plasma/serum, maternal urine, and blood plasma/serum. Understanding of the impact of prenatal disorders on fetal/maternal metabolisms has been sought regarding preeclampsia, fetal malformations, preterm delivery, gestational diabetes mellitus, small for gestational age, and fetal chromosomal disorders (CD).
The underlying challenges have been 2-fold, either searching for predictive biomarkers of conditions such as preeclampsia or preterm or seeking disease metabolic descriptions to complement existing clinical strategies. In the case of CDs, current diagnostic methods comprise invasive procedures such as chorionic villous sampling (CVS) and amniocentesis performed at 10–13 gestational weeks and 15–20 gestational weeks, respectively. These procedures involve some risk for mother and fetus (0.5–1% fetal loss ) and, currently noninvasive screening tests are used in the first and second trimesters (T) based on ultrasound and maternal serum concentrations of free β-human chorionic gonadotrophin, pregnancy-associated plasma protein A, alpha-fetoprotein, unconjugated estriol, or inhibin A.
First-T screening combines maternal age, fetal nuchal translucency thickness and serum free β-human chorionic gonadotrophin, and pregnancy-associated plasma protein A, with the detection and false-positive rates (FPRs) of 85-95% and 5% for trisomy 21 (T21), and > 90% and 1% for other aneuploidies (trisomy 18 [T18] and trisomy 13 [T13]). In the second T, biomarker combination provides 70–75% detection rates and 5% FPR for T21.
Analysis of cell-free fetal DNA in maternal blood has higher performance, with first T detection rates of 98.6-100%, 100% and 91.7% for T21, T18 and T13, respectively, and FPR < 1%. However, the high cost of such tests and their relative low detection rates for T13 presently hinder their use as replacements of CVS or amniocentesis. Hence, there is still a need for biomarkers with improved reliability as well as for a deeper knowledge of how CDs have an impact on pregnancy metabolism, thus enlightening the conditions’ mechanisms and interaction between genetic and metabolic traits.
The metabolic impact of CDs on second-T amniotic fluid was low, viewed by nuclear magnetic resonance (NMR) spectroscopy, but a subsequent high-performance liquid chromatography study unveiled amino acid changes in T21 cases. The NMR of second-T maternal urine resulted in a CD classification with 87% sensitivity and 84% specificity, suggesting a higher glucose excretion and, again, amino acid alterations.
T21 cases were distinguished from other CDs by possible changes in energy, nucleotide, and amino acid metabolisms. A subsequent mass spectrometry study of first- and second-T maternal urine of T21 revealed increased dihydrouracil (possibly caused by liver abnormalities) and decreased progesterone (probably reflecting impairment of placental function caused by oxidative stress).
Regarding maternal blood, an initial indication of lipid changes in second-T maternal plasma of T21 was later confirmed by lipidomics, which revealed changes in lysophosphatidylcholines and sphingomyelins along with ether-linked phosphatidylcholine (PC) and phosphatidylethanolanine species related to lipid oxidation. The NMR of T21 first-T maternal blood reported changes in 11 metabolites, particularly in 3-hydroxybutyrate, 3-hydroxyisovalerate, and 2-hydroxybutyrate (75% sensitivity, 86.2% specificity).
These results were interpreted as reflecting oxidative stress, poor myelination, and neurotoxicity of the brain in T21 fetuses. A similar study of T18 advanced a combination of 2-hydroxybutyrate, glycerol, and maternal age for T18 detection (73% sensitivity, 97% specificity), whereas trimethylamine, threonine, and creatine levels seemed to differentiate T18 from T21.
In this work, a NMR metabolomics study of first- and second-T maternal plasma of a general CD group and a T21 subgroup is presented in comparison with controls to determine the following: (1) establish the time-course adaptations of maternal/fetal metabolism to the conditions and (2) unveil dynamic metabolic biomarkers. Furthermore, blood plasma and lipid extracts are compared in terms of T21 classification, the latter adding specific information on lipid composition. Finally, the CD-related metabolic changes measured in second-T maternal plasma are correlated to the corresponding urine samples, thus providing a more complete metabolic description of CD in midpregnancy.
Materials and Methods
Samples
Blood samples were collected for women carrying CD fetuses and healthy pregnant women, in their first and second Ts. These groups were independent because the CD diagnosis was performed in one of the trimesters only. First- and second-T CD cases were diagnosed by CVS (12–13 gestational weeks) and amniocentesis (14–23 gestational weeks), respectively. Table 1 lists sample numbers and group details.
Subject group | n | Maternal age, y | Gestational age, wks | Prepregnancy BMI, kg/m –2 |
---|---|---|---|---|
Whole-blood plasma | ||||
Controls, first T | 25 | 24–37 (31) | 11–13 (13) | 19–38 (25) [for n = 23] |
CD, first T a | 15 | 21–44 (35) | 12–13 (13) | 19–39 (26) [for n = 9] |
T21, first T | 8 | 21–44 (35) | 12–13 (13) | 21–30 (24) [for n = 5] |
Controls, second T | 49 | 25–42 (36) | 16–24 (17) | 20–33 (24) [for n = 37] |
CD, second T b | 30 | 25–43 (36) | 14–23 (17) | 19–34 (26) [for n = 26] |
T21, second T | 14 | 26–43 (36) | 15–23 (17) | 20–33 (25) [for n = 12] |
Plasma lipid extracts | ||||
Controls, first T | 15 | 24–37 (31) | 12–13 (13) | 22–35 (25) [for n = 15] |
T21, first T | 7 | 32–44 (37) | 12–13 (13) | 21–30 (25) [for n = 4] |
Controls, second T | 15 | 28–42 (37) | 16–22 (17) | 20–26 (22) [for n = 11] |
T21, second T | 12 | 26–43 (37) | 14–23 (17) | 22–33 (27) [for n = 8] |
a First-trimester CDs comprise the following: trisomies 21 (n = 8), 13 (n = 2), and 9 (n = 1); triploidy (69, XXX, n = 1); translocations (familial, n = 2); and mosaicism (autosomal, n=1)
b Second-trimester CDs comprise the following: trisomies 21 (n = 14) and 18 (n = 2); Kleinfelter syndrome (n = 2); XYY syndrome (n = 2), XXX syndrome (n = 1); triploidy (69, XXX, n = 1); mosaicism (autosomal, n = 1); marker chromosome (autosomal, n = 1); translocations (2 familial plus 1 de novo, n = 3); inversions (familial, n = 2); and deletion (autosomal, n = 1).
Samples were collected under the approval of the Coimbra Hospital Centre Ethical Committee (references 18/04 and 29/09), and individual informed consents were obtained. Because of the medical restrictions in controlling/restricting pregnant women’s diet, plasma samples were collected under nonfasting conditions, the effect of which on NMR-viewed plasma metabolome having been found residual. Blood (9 mL) was collected into sodium heparin tubes and centrifuged (1500 × g , 4°C, 10 minutes) within 30 minutes of collection. Supernatants were stored at –80°C until NMR analysis. Sample preparation for NMR is described elsewhere.
Lipid extracts were prepared, for T21 cases only, by extracting plasma samples in duplicate, using the methyl-tert-buthyl ether method. Organic phases were dried and stored at –80°C until NMR analysis. Before analysis, extracts were thawed at room temperature, followed by the addition of 600 μL CDCl 3 (99.96%) with tetramethylsilane (0.03% [vol/vol]). The mixture (580 μL) was transferred into a 5 mm NMR tube. Urine collection has been described elsewhere.
NMR spectroscopy
NMR spectra were recorded, at 300 K, on a Bruker Avance DRX 500 spectrometer operating at a 500.13 MHz frequency for proton and equipped with an actively shielded gradient unit with a maximum gradient strength output of 53.5 G/cm (Bruker, Ettingen, Germany). For each plasma sample, 3 types of 1D 1 H NMR spectra were recorded: a standard spectrum (1D NOESY-presat), a Carr-Purcell-Meiboom-Gill (CPMG) spectrum, and a diffusion-edited spectrum. The plasma acquisition parameters are listed elsewhere.
For lipid extracts, 1 H NMR spectra were acquired at 298 K using a standard 90° pulse sequence (zg), with a 2.34 second acquisition time, 5 second relaxation delay (d1), 128 scans, 32 k data points, and 7002.801 Hz spectral width. Each free induction decay was zero filled to 64 k points and multiplied by a 0.3 Hz exponential function prior to Fourier transformation. Spectra were manually phased, baseline corrected, and chemical shift referenced to α-glucose H1 (δ 5.23) or tetramethylsilane (δ 0.00), for plasma and extracts, respectively.
Peak assignments were carried out using 2D NMR and several databases, including the Bruker Bioref database. In some cases, statistical total correlation spectroscopy was performed to aid assignment (Matlab; The MathWorks, Inc, Natick, MA). The NMR analysis of urine samples is described elsewhere.
Chemometrics
For blood plasma, full-resolution 1D spectra were used to construct data matrices, after exclusion of the water (δ 4.5–5.0) and ethanol (δ 1.15–1.20 and δ 3.62–3.68) regions, the latter found randomly in blood samples, possibly caused by a skin disinfection prior to collection. For lipid extracts, full-resolution spectra were also used, excluding water (δ 1.60–1.97) and CDCl 3 (and corresponding satellites) (δ 7.04–7.05, δ 7.21–7.32 and δ 7.46–7.47) peaks. 1D spectra were aligned using recursive segment-wise peak alignment (RSPA) and normalized using probabilistic quotient normalization (Matlab 7.9.0; The MathWorks, Inc).
A principal component analysis (PCA) and partial least squares discriminant analysis (PLS-DA) were performed on data scaled by unit variance, Pareto, and centered scaling (SIMCA-P 11.5; Umetrics, Umeå, Sweden), with unit variance providing the best results. PCA and PLS-DA loadings were backtransformed, multiplying each variable by its SD and colored according to variable importance to the projection (VIP) (Matlab 7.9.0; The MathWorks, Inc). For PLS-DA models, a randomized (Monte Carlo) cross-validation approach (in-house developed) was carried out with recovery of Q 2 values (predictive power) and confusion matrices; simultaneously, a randomized class-permutation procedure assessed the null hypothesis.
Classification rates, specificity, and sensitivity were computed, and model predictive power was further assessed by a receiver-operating characteristic map. PLS-DA models were considered robust for minimal overlap of the distributions of true (alternative hypothesis) and permuted (null hypothesis) Q 2 values. Variable (spectral data points) selection was applied as described previously, followed by PLS-DA. Because the best results were obtained with CPMG spectra, those were further considered for signal integration (Amix 3.9.5; Bruker BioSpin).
Integrals were normalized by probabilistic quotient normalization and variations assessed by univariate analysis (Shapiro-Wilk test, Student t test, and Wilcoxon test) (R-statistical software ). Bonferroni correction was used to adjust P values for multiple comparisons by setting the significance cutoff to α/n with α = 0.05 and n = number of metabolites/resonances simultaneously tested.
Choline resonances in extract spectra were deconvoluted prior to integration. For significantly changed metabolites ( P < .05), effect size was calculated. A row-concatenated matrix (augmented row-wise) of second-T plasma and urine spectra obtained from common donors (22 controls, 26 CDs, 12 T21) was considered for PLS-DA. Contributing metabolites were used for intra- and interbiofluid Pearson product-moment correlation analysis (R-statistical software). Correlation coefficients (r) ranged from –0.609 to 0.988 and correlations were confirmed by scatter plot inspection.
Results
Metabolic fingerprinting of chromosomal disorders in the first trimester
The average CPMG 1 H NMR spectrum of control first-T maternal plasma ( Figure 1 , A) is dominated by broad lipoprotein and glucose peaks, whereas the corresponding lipid extract spectrum ( Figure 1 , B) shows additional information on lipid composition, namely several different methylene and methine environments, sphingomyelins and PC choline resonances, triglycerides (TG) and phospholipids (PL) glyceryl resonances, and 18:2, 20:4, and 22:6 fatty acids (FA).

The PCA of the CPMG 1 H NMR spectra of first-T blood plasma of controls and CD samples (not shown) revealed no trends, and the corresponding PLS-DA model had poor performance (Q 2 0.300) ( Table 2 and Supplemental Figure 1 , A). Variable selection was applied to the spectra, and the subsequent PLS-DA first-T plasma model ( Figure 2 , A) was indeed much improved (Q 2 0.550, Table 2 and Supplemental Figure 1 , B), the set of variables selected being shown as gray dots in Figure 1 , A.
Subject group | Original spectra | Spectra after variable selection | |||||||||
---|---|---|---|---|---|---|---|---|---|---|---|
LV | Q 2 | CR | Sens | Spec | LV | Q 2 | CR | Sens | Spec | VS, % | |
First trimester | |||||||||||
Whole blood plasma | |||||||||||
Controls (n = 24)/CD (n = 15) | 2 | 0.3 | 70% | 41% | 89% | 1 | 0.7 a | 88% a | 78% a | 94% a | 34% a |
Controls (n = 24)/T21 (n = 8) | 1 | 0.2 | 76% | 18% | 96% | 1 | 0.7 a | 92% a | 72% a | 99% a | 32% a |
Plasma lipid extracts | |||||||||||
Controls (n = 15)/T21 (n = 7) | — | — | — | — | — | 1 | 0.8 a | 87% a | 78% a | 91% a | 25% a |
Second trimester | |||||||||||
Whole blood plasma | |||||||||||
Controls (n = 49)/CD (n = 30) | — | — | — | — | — | 2 | 0.7 a | 89% a | 79% a | 94% a | 31% a |
Controls (n = 49)/T21 (n = 14) | 1 | 0.3 | 78% | 26% | 93% | 1 | 0.7 a | 85% a | 44% | 97% a | 32% a |
Plasma lipid extracts | |||||||||||
Controls (n = 15)/T21 (n = 13) | 2 | 0.7 a | 94% a | 94% a | 94% a | 2 | 0.9 a | 98% a | 100% a | 97% a | 33% a |
Whole plasma and urine | |||||||||||
Controls (n = 22)/CD (n = 26) | 1 | 0.3 | 63% | 62% | 64% | 1 | 0.6 a | 90% a | 85% a | 95% a | 32% a |
Controls (n = 22)/T21 (n = 12) | — | — | — | — | — | 1 | 0.8 a | 95% a | 85% a | 100% a | 32% a |

The fact that some selected variables fall in regions of weak/broad resonances or slight baseline nuances (eg, in the aromatic region) indicates the importance of spectral detail for sample classification. Computed metabolite changes ( Table 3 ) showed that CD samples carry lower amounts of high-density lipoprotein (HDL) lipoproteins, proline, and methanol and increased levels of β-hydroxybutyrate and pyruvate (as well as qualitative increases in acetate, acetone, citrate, creatine, and glucose), as clearly seen in a Volcano plot format ( Figure 2 , A, right panel).
Compound | Chemical shift per ppm a | Variation (E.S., b P value c ) | |||
---|---|---|---|---|---|
First trimester | Second trimester | ||||
Controls vs CD | Controls vs T21 | Controls vs CD | Controls vs T21 | ||
Whole-blood plasma | |||||
CH 3 lipids HDL | 0.78–0.86 (br) | ↓ –0.86 ± 0.67, 1.3 × 10 –2 | ↓ –1.10 ± 0.84, 8.8 × 10 –3 | ↓ | ↓ |
(CH 2 ) n lipids HDL | 1.20–1.25 (br) | ↓ –0.68 ± 0.66, 4.9 ×10 –2 | ↓ | ↓ | ↓ –0.56 ± 0.60, 2.2 × 10 –2 |
CH 3 lipids LDL plus VLDL | 0.86–0.90 (br) | — | — | ↑ d | ↑ |
(CH 2 ) n lipids LDL plus VLDL | 1.25–1.30 (br) | — | — | ↑ d | ↑ |
CH 3 HDL/LDL plus VLDL | ↓ –0.73 ± 0.67, 4.7 × 10 –2 | ↓ | ↓ | ↓ | |
Alanine | 1.46 (d) | — | — | ↑ d | ↑ |
C H 2 CH 2 CO lipids | 1.52–1.60 (br) | ↑ | ↑ | — d | ↑† |
Acetate | 1.91 (s) | ↑ | ↑ | ↓ –0.51 ± 0.47, 1.2 × 10 –2 d | ↓ |
Acetone | 2.22 (s) | ↑ | ↑ | — | — |
β-hydroxybutyrate | 2.39 (m) | ↑ 0.69 ± 0.66, 2.6 ×10 –3 d | ↑ 0.70 ± 0.82, 1.8 × 10 –2 | ↑ | — e |
Pyruvate | 2.36 (s) | ↑ 0.81 ± 0.67, 3.0 × 10 –2 | ↑ 1.22 ± 0.85, 4.6 × 10 –2 | ↑ | ↑ |
Citrate | 2.51 (d) | ↑ | ↑ | ↑ 0.51 ± 0.47, 3.3 × 10 –2 | ↑ |
Albumin-lysyl | 2.92–3.03 (br) | — | — | — | ↑ e |
Creatine | 3.03 (s) | ↑ | ↑ | — d | — |
N(CH 3 ) 3 choline HDL | 3.20–3.23 (br) | ↓ –1.06 ± 0.69, 3.9 × 10 –3 | ↓ –1.28 ± 0.86, 5.1 × 10 –3 | ↓ | ↓ |
Proline | 3.34 (br) | ↓ –0.78 ± 0.67, 1.7 × 10 –2 | ↓ –1.02 ± 0.84, 1.8 × 10 –2 | ↓ | — e |
Methanol | 3.36 (s) | ↓ –0.98 ± 0.68, 3.0 × 10 –3 f | ↓ –1.01 ± 0.84, 2.3 × 10 –2 | — d | ↑ e |
Creatinine | 4.05 (s) | — | — | ↑ 0.60 ± 0.47, 1.4 × 10 –2 d | — e |
Glucose | 5.23 (d) | ↑ | ↑ | ↑ | ↑ 0.47 ± 0.60, 3.0 × 10 -2 |
Urea | 5.77 (br) | — | — | ↓ –0.81 ± 0.48, 6.7 × 10 -4 d,f | ↓ –0.87 ± 0.61, 2.6 × 10 –3 f |
Unknown 1 | 7.19–7.38 (br) | — | — | — | ↑ e |
Plasma lipid extracts | |||||
Fatty acids | |||||
-CH 3 | 0.89 (t) | n.d. | ↓ –0.99 ± 0.67, 1.3 × 10 –2 | n.d. | ↓ |
-(CH 2 ) n – | 1.25 (br) | n.d. | ↓ | n.d. | ↓ –0.56 ± 0.54 |
=CHC H 2 (CH 2 ) n – | 1.26-1.40 (br) | n.d. | ↓ | n.d. | — d |
-CH 2 CH= 18:2 | 2.05 (q) | n.d. | ↓ –0.86 ± 0.66, 1.7 × 10 –2 | n.d. | — d |
-CH 2 CH= 20:4 | 2.10 (q) | n.d. | ↓ | n.d. | ↓ |
-C H 2 COOC- | 2.28–2.36 (m) | n.d. | ↓ | n.d. | ↓ |
-C H 2 COOC- 22:6 | 2.37 (t) | n.d. | ↑ | n.d. | ↓ –0.94 ± 0.56, 8.5 × 10 –4 d,f |
=CHC H 2 CH= 18:2 | 2.77 (t) | n.d. | ↓ | n.d. | ↑ d |
=CHC H 2 CH= 20:4 | 2.79-2.86 (m) | n.d. | ↓ | n.d. | ↓ –0.83 ± 0.55, 3.5 × 10 –3 f |
-HC=CH- 18:2 | 5.29–5.35 (m) | n.d. | ↓ | n.d. | — d |
-HC=CH- 20:4 | 5.35–5.43 (m) | n.d. | ↓ | n.d. | ↓ –0.79 ± 0.55, 6.5 × 10 –3 |
Phospholipids | |||||
-N(CH 3 ) 3 choline SM | 3.31 (br) | n.d. | ↓ | n.d. | — d |
-N(CH 3 ) 3 choline PC | 3.34 (br) | n.d. | ↓ –1.36 ± 0.70, 2.1 × 10 –4 d | n.d. | ↓ –1.11 ± 0.57, 5.5 × 10 –5 f |
-CH 2 N(CH 3 ) 3 choline total | 3.78 (br) | n.d. | ↓ –1.59 ± 0.72, 8.7 × 10 –5 d | n.d. | ↓ –1.30 ± 0.58, 2.5 × 10 –5 f |
Glyceryl-C3H 2 | 3.95 (m) | n.d. | ↓ –1.53 ± 0.71, 1.1 × 10 –4 d | n.d. | ↓ –1.14 ± 0.57, 1.7 × 10 –4 f |
Glyceryl-C1H 2 | 4.34 (br) | n.d. | ↓ –0.83 ± 0.66, 6.8 × 10 –3 | n.d. | ↓ –1.36 ± 0.59, 3.0 × 10 –5 f |
-POCH 2 – | 4.40 (br) | n.d. | ↓ –1.08 ± 0.67, 4.2 × 10 –3 | n.d. | ↓ –0.79 ± 0.55, 6.1 × 10 –3 |
Unknown 3 | 7.71 (m) | n.d. | — | n.d. | ↑ 1.08 ± 0.57, 3.9 × 10 –4 d,f |
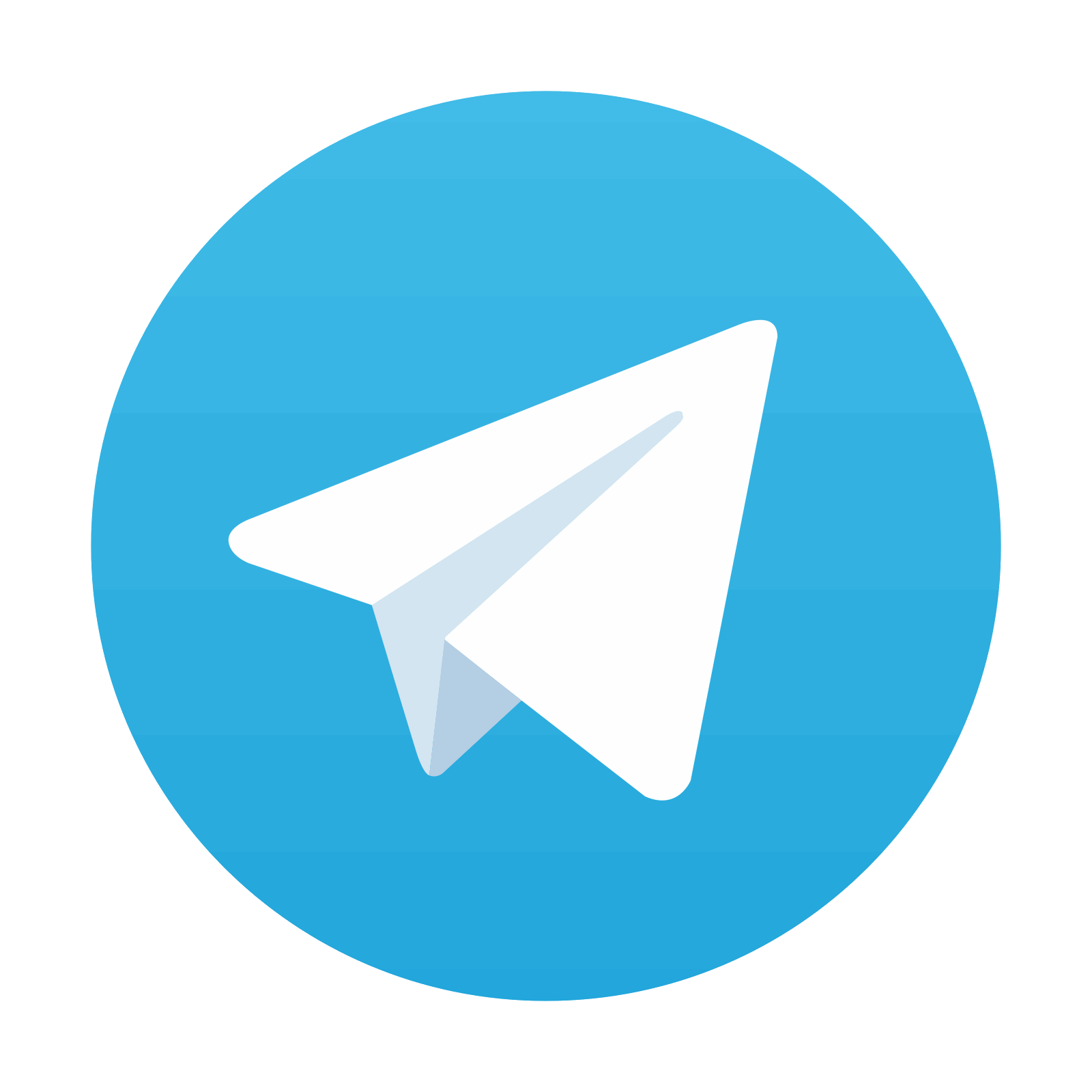
Stay updated, free articles. Join our Telegram channel

Full access? Get Clinical Tree
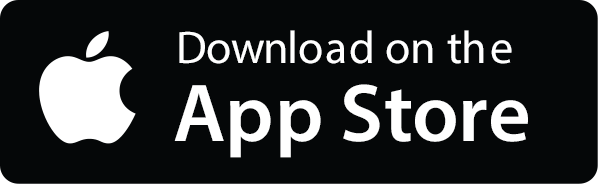
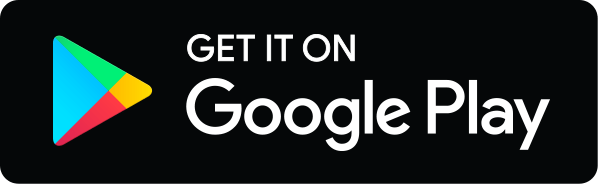
