Introduction
Respiratory illnesses are among the most common and potentially life-threatening conditions encountered in the newborn. This makes the radiologic examination of the infant chest a common and essential tool to guide the diagnosis and treatment of neonatal lung disease. Using and interpreting imaging studies well requires teamwork and open lines of communication between clinicians (including obstetricians, surgeons, and pediatric subspecialists as appropriate) and radiologists. This is particularly true now, as digital radiology and point-of-care ultrasound make direct interaction between clinicians and radiologists less common. Although the plain radiograph remains the mainstay of neonatal chest imaging, other modalities such as ultrasound and computed tomography are increasingly used in the evaluation of acutely ill neonates. Technologic advances in fetal imaging also now permit improved prenatal diagnosis and in some cases fetal treatment of congenital chest lesions.
To provide high-quality care to the ill neonate, clinicians must understand the capabilities and limitations of common imaging tools and learn to identify the radiographic findings of common neonatal respiratory conditions. Although written materials aid in this process, pattern recognition can be gained only through regular review of films. This chapter highlights important aspects of neonatal chest imaging and provides specific clinical examples directed toward trainees and practicing clinicians. More in-depth discussions of neonatal chest imaging can be found in other reviews.
Radiation Exposure
Several common imaging modalities including plain radiography, computed tomography (CT), fluoroscopy, and nuclear medicine use ionizing radiation to produce images. As radiation dose exposure is cumulative and even low total exposure in infancy is associated with slightly increased risk of later malignancy, clinicians must weigh the diagnostic advantage of an imaging modality with its expected radiation dose. The typical dose from a single chest X-ray is approximately 0.008 to 0.03 millisieverts (mSv) depending on the technique and equipment. When combined with an abdominal radiograph, the dose is 1.5 to 2 times higher. By comparison, the total natural radiation exposure from sources such as the sun and radioactive materials in the air and soil amounts to about 3 mSv per year at sea level and 5 to 6 mSv at an elevation of 5000 feet. The dose from a continuous digital fluoroscopic voiding cystourethrogram is approximately 0.45 to 0.59 mSv. The use of pulsed fluoroscopy optimized for children reduces the dose to 0.05 to 0.07 mSv. The effective dose received from an upper gastrointestinal series is 1.2 to 6.5 mSv. For a chest CT taken without consideration of a patient’s weight or dose-lowering techniques, the radiation dose is approximately 4 to 7 mSv. With low-dose, high-resolution CT, the dose is reduced to about 1 mSv with negligible loss in image quality.
To minimize radiation exposure, clinicians and radiologists should adhere to the practice of as low as reasonably achievable (ALARA), which requires weight-based protocols, limiting the number of studies performed, and the use of non-radiation modalities such as ultrasound (US) and magnetic resonance imaging (MRI) when appropriate. The use of collimation (restriction of the X-ray beam to the desired anatomic area) and shielding of adjacent structures, particularly the gonads, is essential to reduce unnecessary radiation exposure. Health care workers also need to be aware of the potential risks to themselves and take appropriate precautions to minimize exposure.
Imaging Modalities
Chest Radiograph
The plain chest radiograph or X-ray (CXR) is the most widely used imaging modality in the neonatal intensive care unit (NICU). The CXR utilizes the natural contrast between air (dark or black on a standard radiograph) and fluid or tissue (white or gray). Appropriate positioning of the infant is essential to produce a high-quality radiograph. A “rotated” CXR may lead to a false diagnosis of cardiomegaly, mediastinal shift, atelectasis, or abnormal central line location ( Fig. 9-1 ). When possible, the infant’s arms should be extended away from the chest to prevent the scapulae from obscuring the upper lung fields.

In most cases, a single anterior–posterior (AP) view with the infant in a supine position is adequate to assess the chest wall, heart, airway, and lungs, and any invasive lines or tubes. Lateral radiographs of the chest are not routinely necessary but can aid in specific circumstances such as assessment of the retrocardiac lung fields, diagnosis of air-leak syndromes, and evaluation of pleural drain location. A systematic approach is recommended when describing a CXR to avoid missing potentially important findings. The “alphabet” approach reminds clinicians to assess the Airway (trachea and its branches), Bones (clavicles, ribs, scapulae, vertebrae, and humeri), Cardiac structures (heart site, size, shape, and border and great vessels), Diaphragm, Effusions (small effusions blunt the costophrenic angle), Fields and fissures (adequacy and symmetry of lung field expansion and thick or fluid-filled fissures), Gastric fundus (stomach bubble and other visible intra-abdominal structures), and Hilum and mediastinum.
In a normal, correctly positioned AP CXR the neonatal chest is trapezoid in shape with horizontal ribs ( Fig. 9-2 ). The diaphragm is domed bilaterally and lies at the level of the sixth to the eighth ribs. Both lungs should be symmetrically aerated with uniform radiolucency soon after birth. Air bronchograms in the lung bases, particularly in the lower left lobe behind the cardiac border, are normal. Pulmonary vascular markings are visualized centrally and become less prominent toward the periphery. The transverse cardiothoracic ratio should not exceed 60% to 65%. Elimination of the normal border between the radiopaque thoracic structures (e.g., heart, great vessels) and the radiolucent lung, commonly referred to as the “loss of the silhouette” sign, suggests lobar atelectasis or pneumonia, a localized fluid collection, or intrathoracic mass. The thymus is often prominent after birth and may occupy the majority of the upper chest. It can involute during the first days of life, particularly in stressed infants. If a thymic shadow is not visualized on CXR, a US may help evaluate for thymic aplasia.

Ultrasound
US is an oscillating sound wave with a frequency outside the human hearing range. The most common form of US used in diagnostic imaging is the pulse-echo technique with a brightness-mode (B-mode) display. B-mode involves transmission of a US pulse into the body. The depth of penetration of the pulse depends on the acoustic impedance of the tissue. The reflection of the pulse (“echo”) by the tissue back to the US transducer generates the image. Doppler and motion mode are additional US modes that facilitate visualization of blood flow and motion of tissue over time ( Fig. 9-3 ).

The use of US to examine the airway and lungs of neonates is gaining popularity; however, further studies are needed to define its diagnostic accuracy and interrater reliability. The advantages of US include its relative ease of use, “real-time” imaging capabilities, and lack of ionizing radiation. In the NICU, lung US is most commonly used to diagnose pleural effusion and to assess diaphragmatic movement. US can also be used to evaluate for suspected congenital lung lesions and for point-of-care diagnosis of air-leak syndromes and assessment of endotracheal tube and vascular catheter location. The use of US to differentiate between common respiratory conditions such as respiratory distress syndrome, transient tachypnea of the newborn, and meconium aspiration has been reported but requires further study.
In the healthy neonate, the normal pleural–lung interface appears on lung US as “A-lines,” echogenic horizontal lines that move continuously with respiration ( Fig. 9-4 ). The remaining lung is air filled and appears black. The presence of fluid or consolidation of the interstitial or alveolar compartments produces “B-lines,” white projections that extend from the pleural line to the edge of the screen and obscure A-lines (see Fig. 9-4 ). B-lines are often present in the first few days of life even in infants without respiratory distress and indicate the presence of unabsorbed fetal lung fluid.

Computed Tomography
CT combines a series of X-rays performed helically around an axis of rotation to generate high-quality cross-sectional images of the body. Chest CT is useful for the identification and diagnosis of space-occupying lesions such as congenital pulmonary airway malformation (CPAM), bronchopulmonary sequestration (BPS), and congenital lobar emphysema. CT and CT angiography (CTA) are also increasingly used in the diagnosis and management of severe bronchopulmonary dysplasia (BPD) and pulmonary hypertension. Although low-dose CT substantially reduces the effective radiation dose compared to previous techniques, clinicians should carefully assess the risks and benefits of this imaging modality prior to ordering any study.
Fluoroscopy
Fluoroscopy produces real-time, dynamic images using rapid, sequential X-rays. Fluoroscopy is most commonly used in neonates and infants to image the gastrointestinal and genitourinary tracts. In infants with respiratory distress of unclear etiology, fluoroscopy may aid in the identification of a tracheoesophageal fistula or occult aspiration. Fluoroscopy can also assess diaphragmatic excursion and diagnose large-airway disease such as tracheobronchomalacia. As described earlier, fluoroscopy can result in substantial radiation exposure if dose-limiting procedures such as pulsed fluoroscopy, reduced pulse widths and pulse rates, appropriate beam filtration, increased source-to-skin distance, and proper collimation are not used appropriately.
Magnetic Resonance Imaging
MRI utilizes the energy emitted by magnetically aligned protons to image anatomic structures within the body. MRI does not require exposure to ionizing radiation; however, its use is limited by its lack of availability, its expense, and the frequent need to administer anesthesia to infants to acquire high-quality images of the lung. The primary role of MRI in the neonate is to image the brain and heart. It can also evaluate mediastinal structures, osseous lesions, and vascular malformations. At present the utility of MRI to assess the lung parenchyma is limited. The introduction of arterial spin labeling and hyperpolarized gas imaging, which provide information about lung perfusion and regional ventilation, may expand the role of MRI in the future.
Invasive Support Devices
There is no evidence to support daily use of CXRs to evaluate the position of invasive support devices, but if malposition is suspected, imaging is warranted to prevent or ameliorate iatrogenic complications. In ventilated infants, the endotracheal tube (ETT) should be placed in the mid trachea between the second and fourth thoracic vertebral bodies and at least 1 cm above the carina. Deep positioning, typically into in the right main stem bronchus, can result in unilateral baro- and volutrauma, pneumothorax, and collapse of the contralateral lung ( Fig. 9-5 ). When performing a radiograph to determine ETT location, care should be taken to position the infant without rotation and with the neck in neutral position. Extension of the neck will misleadingly suggest high placement of the ETT, and flexion the opposite.

The umbilical artery and vein are commonly used to gain vascular access during the first days of life in preterm and ill neonates. On radiograph, an umbilical vein catheter (UVC) should lie above the liver and diaphragm near the junction of the inferior vena cava and the right atrium. Lateral CXR and thoracic vertebral level on AP CXR do not accurately identify UVC tip location. Echocardiogram is a more reliable, but costlier means to determine UVC location. An umbilical artery catheter (UAC) can be placed in a “high” position, with the tip above the abdominal visceral arteries between the T7 and the T9 vertebral bodies, or in a “low” position with the tip between the L3 and the L5 vertebral bodies ( Fig. 9-6 ). Data from a small number of randomized infants suggest that “high” placement of UACs is associated with a lower incidence of vascular complications (e.g., ischemic injury, aortic thrombosis).

Peripherally inserted central venous catheters (PICCs) are optimally placed in an upper or lower extremity with the terminal end in a major vein near the heart ( Fig. 9-7 ). As a reference, the cavoatrial junction is located approximately two vertebral bodies below the carina on AP CXR. US can identify peripheral veins during PICC placement and assess line position. As PICCs are prone to migration, periodic surveillance is recommended to prevent iatrogenic complications such as pleural effusion or cardiac tamponade ( Fig. 9-8 ). In particular, sudden worsening of respiratory status when an upper extremity PICC is present and new onset limb swelling warrant prompt evaluation of the catheter’s location and function. Arm positioning during placement and surveillance of upper extremity PICCs also requires specific attention as movement (flexion/extension at the elbow and abduction/adduction at the shoulder) can alter tip location.


Infants with gestational ages greater than 34 weeks with early, severe, and reversible cardiorespiratory failure who do not respond to conventional therapy may require extracorporeal membrane oxygenation (ECMO). Venoarterial (VA) and venovenous (VV) ECMO are both utilized in neonates. In VA ECMO, catheters are inserted into the internal jugular vein and common carotid artery. The tip of the venous cannula is most commonly placed in the mid-right atrium and the tip of the arterial cannula is placed at the junction of the common carotid artery and the aortic arch ( Fig. 9-9 ). In VV ECMO, blood is drained and reinfused though a dual-lumen catheter inserted though the internal jugular vein into the right atrium. CXR can confirm correct placement of ECMO catheters, but echocardiography is a more reliable technique.

Common Etiologies of Respiratory Distress in Infants
Respiratory Distress Syndrome
Primary respiratory distress syndrome (RDS) results from surfactant deficiency in the premature neonate and is the most common respiratory disorder among infants born at less than 32 weeks’ gestation. Lower gestational age and birth weight, lack of exposure to antenatal corticosteroids, perinatal asphyxia, and maternal diabetes are important risk factors for RDS. Clinical signs of RDS often present at or soon after birth and include tachypnea, grunting, chest wall retractions, nasal flaring, and hypoxia. Importantly, these signs may be indistinguishable from other causes of early respiratory failure such as congenital pneumonia (especially secondary to group B Streptococcus ), sepsis, and persistent pulmonary hypertension of the newborn (PPHN) in more mature infants. Secondary surfactant deficiency may also occur later in the neonatal period concomitant with episodes of hypoxemia, pulmonary edema, or infection (e.g., respiratory syncytial virus, bacterial pneumonia).
The CXR appearance in RDS depends on the severity of the disease. The classic findings are low lung volumes with a fine granular or “ground-glass” appearance with air bronchograms ( Fig. 9-10 ). In mild RDS, a diffuse, linear granular pattern is most common. Air bronchograms become more prominent as the disease worsens and in severe cases the lungs are opaque and the cardiac border is often difficult to identify ( eFig. 9-1 ). These CXR findings are modified by the administration of positive airway pressure and exogenous surfactant. Exogenous surfactant typically distributes throughout the lung in a nonuniform way and can result in a heterogeneous radiographic appearance with asymmetric multifocal opacities. This pattern may mimic the appearance of pneumonia and can be difficult to differentiate if earlier imaging is not available. When severe “RDS” is observed in more mature infants and fails to respond to conventional management, clinicians should consider congenital etiologies such as surfactant deficiencies and alveolar capillary dysplasia.

Transient Tachypnea of the Newborn
Transient tachypnea of the newborn (TTN) is characterized by mild to moderate respiratory distress that gradually improves during the first 48 to 72 hours of life. TTN results from the delayed clearance of fetal lung fluid and is more commonly observed in infants with a history of maternal diabetes and those born via cesarean section. The CXR shows normal to mildly overexpanded lungs with a diffuse hazy appearance and increased interstitial streaky shadowing extending to the periphery. Small pleural effusions, typically seen as prominence of the interlobar fissures, are common ( Fig. 9-11 ). Early CXRs in TTN can appear similar to those of more serious conditions such as infection, surfactant deficiency, or cardiac failure.

Meconium Aspiration Syndrome
Meconium is a viscous, hyperosmolar substance composed of water, mucus, gastrointestinal secretions, bile acids, pancreatic enzymes, lanugo, vernix caseosa, and blood. Meconium-stained fluid is present in approximately 10% to 15% of live births, but only 1% to 2% suffer significant respiratory compromise due to aspiration of meconium. Fetal stress (e.g., hypoxemia, acidosis, infection) is a common antecedent of in utero passage of meconium, and most infants with meconium aspiration syndrome (MAS) are born at or near full term. Severe respiratory distress and hypoxia are characteristic of MAS, and ventilation perfusion mismatch and acidosis can worsen PPHN.
The CXR findings in MAS range from lung hyperexpansion with or without heterogeneous patchy infiltrates to complete opacification of the thorax ( Fig. 9-12 ). Pleural effusion can also develop and air trapping due to a “ball–valve” phenomenon may lead to pneumothorax. Exogenous surfactant therapy is often beneficial, but similar to RDS, it can transiently increase the heterogeneous appearance of the lung parenchyma.


Pneumonia
Pneumonia is an important cause of neonatal morbidity and mortality. The estimated incidence ranges from 1.5 to 5 cases per 1000 live births. Neonatal pneumonia is generally classified as early or late in onset. Early-onset pneumonia presents within the first week of life with respiratory distress and systemic signs of sepsis including poor perfusion, lethargy, and jaundice. Most early-onset pneumonias are acquired congenitally from transplacental inoculation or perinatally from the maternal vaginal tract. The presentation of early-onset pneumonia is similar to RDS, but a history of prolonged rupture of membranes, chorioamnionitis, or elevated inflammatory markers may help distinguish between the two. Pleural effusion is also more common in pneumonia than in RDS ( Fig. 9-13 ). The most common pathogens associated with early pneumonias are group B Streptococcus and gram-negative rods. Congenital viral infections and occasionally atypical bacterial (e.g., Chlamydia trachomatis ) and fungal infections can also present as early pneumonia.

Late-onset pneumonia presents after the first week of life and is often a nosocomial complication of mechanical ventilation. Common pathogens include coagulase-negative Staphylococcus , Staphylococcus aureus , gram-negative rods (e.g., Pseudomonas ), and Candida . Viral infections such as rhinovirus, respiratory syncytial virus, and influenza can also cause late-onset pneumonia. The CXR in late-onset pneumonia is diffusely hazy, similar to RDS, but in contrast to early-onset pneumonia, the lungs often exhibit normal or hyperexpansion with heterogeneously distributed densities ( eFig. 9-2 ). Localized lobar pneumonias are uncommon in neonates but can develop in older infants and, when severe, result in lung necrosis and empyema. A chest CT can help confirm the diagnosis in these cases ( eFig. 9-3 ).


Air-Leak Syndromes
The application of positive airway pressure to poorly compliant or “stiff” lungs can result in leakage of air from the alveoli into the extra-alveolar space. The most common conditions that arise from alveolar air leak are pneumothorax, pneumomediastinum, pneumopericardium, and pulmonary interstitial emphysema (PIE). Rarely, pneumoperitoneum and subcutaneous emphysema can also develop.
Pneumothorax
Pneumothorax is the most common air-leak syndrome in infants and occurs in approximately 2% to 10% of those with birth weights between 500 and 1500 g. The clinical presentation of pneumothorax varies from an incidental finding on CXR to severe respiratory distress and cardiovascular collapse. A large pneumothorax is easily detected on AP CXR. The characteristic findings are air in the pleural space with compression of the affected lung, flattening of the diaphragm, and shift of the mediastinum to the contralateral side ( Fig. 9-14 , eFig. 9-4 ). In contrast, small pneumothoraces can be difficult to appreciate. In the supine infant, free air collects anterior to the lung and a collapsed edge may not be visible. Increased lucency on the affected side or a tiny lucent rim along the diaphragm may be the only findings. A lateral decubitus X-ray with the affected side up is often helpful in this situation. Large pneumothoraces generally require percutaneous decompression, whereas smaller ones that do not cause cardiorespiratory compromise may resorb spontaneously.

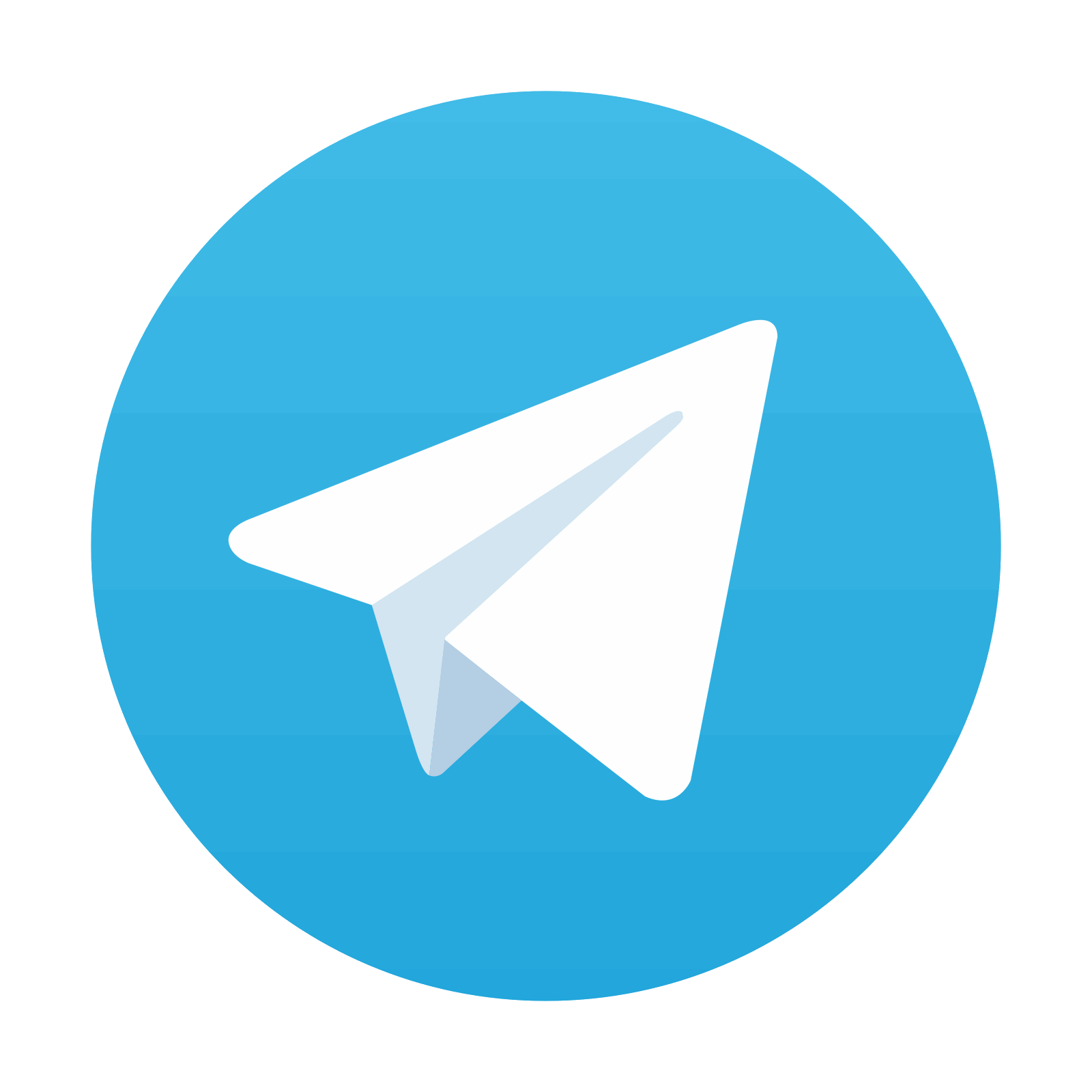
Stay updated, free articles. Join our Telegram channel

Full access? Get Clinical Tree
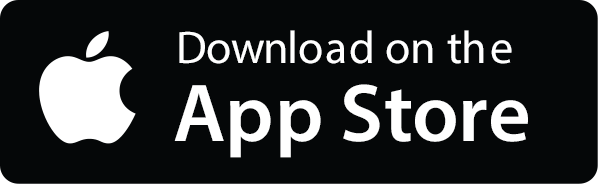
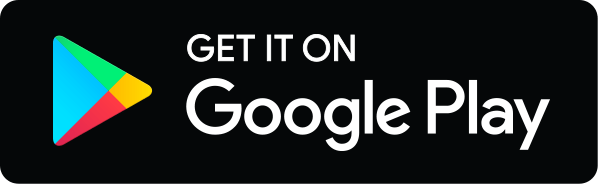
